the Creative Commons Attribution 4.0 License.
the Creative Commons Attribution 4.0 License.
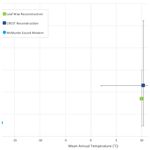
Miocene Climatic Optimum fungal record and plant-based CREST climatic reconstruction from southern McMurdo Sound, Antarctica
Mallory Pilie
Martha E. Gibson
Ingrid C. Romero
Noelia B. Nuñez Otaño
Matthew J. Pound
Jennifer M. K. O'Keefe
Sophie Warny
Deep-time palynological studies are necessary to evaluate plant and fungal distribution under warmer-than-present scenarios such as those of the Middle Miocene. Previous palynological studies from southern McMurdo Sound, Antarctica (SMS), have provided unique documentation for Neogene environments in the Ross Sea region during a time of pronounced global warming. The present study builds on these studies and provides a new climate reconstruction using the previously published SMS pollen and plant spore data. Additionally, 44 SMS samples were reanalyzed with a focus on the fungal fraction of the section to evaluate the fungal distribution under warmer than present conditions. The probability-based climate reconstruction technique (CREST) was applied to provide a new plant-based representation of regional paleoclimate for this Miocene Climatic Optimum (MCO) locality. CREST reconstructs a paleoclimate that is warmer and significantly wetter than present in SMS during the MCO, with mean annual precipitation reconstructed at 1147 mm yr−1 (95 % confidence range: 238–2611 mm yr−1) and a maximum mean annual temperature of 10.3 ∘C (95 % confidence range: 2.0–20.2 ∘C) for the warmest intervals of the MCO. The CREST reconstruction fits within the Cfb Köppen–Geiger climate class during the MCO of SMS. This new reconstruction agrees with previous reconstructions using various geochemical proxies. The fungal palynological analyses yielded surprising results, with only a single morphotype recovered, in low abundance, with concentrations ranging up to 199 fungi per gram of dried sediment. The taxa present belongs to the Apiosporaceae family and are known to be adapted to a wide range of climate and environmental conditions. As fungi are depauperate members of the SMS MCO palynofloras and because the one morphotype recovered is cosmopolitan, using the fungi record to confirm a narrow Köppen–Geiger climate class is impossible. Overall, the study demonstrates refinement of plant-based paleoclimatic reconstructions and sheds light on the limited presence of fungi during the MCO in Antarctica.
- Article
(8190 KB) - Full-text XML
- BibTeX
- EndNote
The Miocene Climatic Optimum (MCO) was a striking climatic event worldwide, but its impact on the Antarctic continent and its massive ice sheets had dramatic implications on many other parts of the world. This is in part because the MCO warming affected an ice-covered continent that is currently covered by ice sheets equivalent to a ∼ 55 m sea level rise (Fretwell et al., 2013). The onset of continental-scale glaciation in Antarctica occurred at approximately 34 Ma (Shackleton et al., 1975; DeConto and Pollard, 2003; Stickley et al., 2004; Pollard and DeConto, 2009; DeConto and Pollard, 2016). Palynological records from ∼ 34 Ma display a shift from rich and diverse vegetation prior to the Eocene–Oligocene (E/O) transition (Askin, 1992, 2000; Askin and Ricci, 1997; Anderson et al., 2011) to an abrupt decline in terrestrial palynomorph concentrations at or around the E/O transition (Warny and Askin, 2011a; Warny et al., 2019). Studies show there was a sharp decrease in vegetation abundance and diversity in Antarctica after the Oligocene (Raine, 1998; Askin and Raine, 2000; Warny and Askin, 2011b) apart from a brief interval of time during the MCO (Warny et al., 2009). The glacial history of Antarctica during the MCO is starting to be well constrained. Geochemical data from deep-sea proxy records highlight a climate during the MCO that was significantly warmer than modern-day temperatures (Lear et al., 2000; Zachos et al., 2001, 2008). At the start of the Miocene, the large glacial event of the Oligocene–Miocene Transition (OMT) is well documented as a disconformity in the ANDRILL southern McMurdo Sound (SMS) record, suggesting expansion of the Antarctic Ice Sheet (AIS) into the Ross Sea at that time (Steinthorsdottir et al., 2021). During the Miocene, the East AIS underwent oscillations across the Sabrina Coast continental shelf, with evidence from erosive surfaces and subglacial tunnel valleys that indicates massive melting of the ice sheet surface generating meltwater pulses (Gulick et al., 2017). By the Middle Miocene, localized pockets of limited tundra still existed at least until 13.85 Ma in the McMurdo Dry Valley area (Lewis et al., 2008) and 12.8 Ma in the Antarctic Peninsula (Anderson et al., 2011). There are no records of vegetation in Plio-Pleistocene sediments younger than these dates. The transition from temperate, alpine glaciation to a dynamic, polythermal ice sheet took place during the Middle Miocene (Anderson et al., 2011). During the MCO, temperatures have been reconstructed ∼ 7–8 ∘C warmer than modern day, and summer sea surface temperatures (SSTs) likely reached 6–10 ∘C in the Ross Sea (note that global mean surface temperature reached 18.4 ∘C) with a brief interval of open-water conditions. CO2 levels reached at least 600 ppm during this interval (You et al., 2009; Levy et al., 2016; Steinthorsdottir et al., 2021). The MCO interval was not driven by a single event, it instead is comprised of a series of maxima and minima in δ18O records, which suggests a complex story of ice sheet fluctuation and reorganizations of oceanic circulation (Holbourn et al., 2022). This complex climatic optimum was succeeded by long-term climatic deterioration towards icehouse conditions (Flower and Kennett, 1994; Shevenell et al., 2004; Holbourn et al., 2007). The ANDRILL AND-2A record, drilled in McMurdo Sound, Antarctica (Fig. 1), is the focus of the present study. It is the most high-resolution Antarctic stratigraphic record of the MCO time interval (Fig. 2) drilled to date in proximity to the Antarctic coastline.
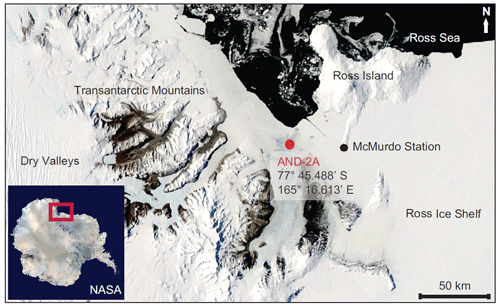
Figure 1Location of the AND-2A core hole in southern McMurdo Sound, western Ross Sea, Antarctica (Warny et al., 2009).
Previous studies associated with the ANDRILL SMS drilling campaign (AND-2A core) depict a warm, moist Antarctica during the MCO interval, with two major abrupt peaks in the abundance of marine, freshwater, and terrestrial palynomorphs (Warny et al., 2009; Feakins et al., 2012; Griener et al., 2015). An interval within the core from 458.11 to 403.47 m b.s.f. is characterized by a concentration increase in in situ palynomorphs composed mostly of pollen, plant spores, and freshwater algae that is dated from 16.56–16.45 Ma (Warny et al., 2009; Griener et al., 2015). A younger interval, ranging in depth between 311.49 to 289.93 m b.s.f., is also characterized by an increase in pollen, plant spores, freshwater algae, and dinoflagellate cysts; this interval is constrained in age between 15.89 and 14.54 Ma (Griener et al., 2015; Warny et al., 2009). The unusually high concentration of palynomorphs recovered for this site suggests a relatively short period of time during the peak of the MCO in which Antarctica became suddenly much warmer (Warny et al., 2009). These authors noted that the absolute abundance of Podocarpidites pollen (podocarp conifer) increased up to 16-fold and that Nothofagidites pollen (southern beech) increased more than 60-fold. These were the most abundant plants on land in the sediment source area adjacent to the Ross Sea during the MCO, along with mosses and a variety of ground cover angiosperms. This vegetation composition suggests low tundra on coastal plains and a mosaic of tundra with shrub-like southern beech and low podocarp trees in sheltered areas (Warny et al., 2009). Based on the pollen and spores recovered, these authors suggested that Antarctica's summer temperatures likely reached at least 10 ∘C around 15.7 Ma. The climate was much warmer, and a substantial increase in moisture delivery to the Antarctic coast also occurred. On the basis of hydrogen isotope data measured from leaf waxes, Feakins et al. (2012) reported that Antarctic coastal precipitation averaged 848 mm yr−1 and ranged from 228–1500 mm yr−1 during the Middle Miocene. These climate fluctuations, along with the release of fresh meltwater to the Ross Sea region, were probably the result of a poleward shift of the jet stream in the Southern Hemisphere. This shift in the jet stream resulted in warmer water being pushed toward the South Pole, allowing some dinoflagellate species to flourish in ice-free conditions and woody plants to thrive in warmer continental temperatures (Warny et al., 2009). A second Antarctic Middle Miocene marine record in Wilkes Land, which is located at a slightly lower latitude compared to ANDRILL AND-2A, also shows a dominance of Podocarpidites conifers and Nothofagidites pollen in an environment of woody sub-Antarctic or sub-alpine vegetation, with some plants representative of shrub tundra and peatlands (Sangiorgi et al., 2018).
Here, we propose revised plant-based temperature and precipitation estimates reconstructed using a climate reconstruction technique (CREST) for the terrestrial source area, and we provide a new fungal record to examine how fungi reacted to the MCO warming.
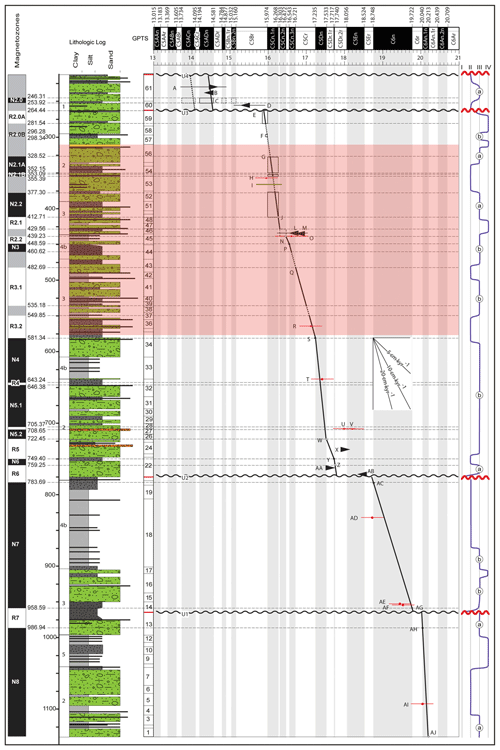
Figure 2Age–depth model of AND-2A. The highlighted red rectangle signifies the depth of samples studied, covering the Middle Miocene Climatic Optimum. The summary lithologic column is plotted on the far left of the age–depth diagram. The magnetic polarity stratigraphy is plotted to the left of the lithologic column. The black and white bar running along the top of the plot is the geomagnetic polarity timescale with selected chrons labeled (Levy et al., 2016).
Fungi play key roles in ecosystems; many have restricted geographic distributions, have known host and substrate preferences, and are important local environmental indicators due to their short dispersal distances. Yet, the distribution of terrestrial fungi in marine sediments is very poorly understood, and no data of this type exist for Antarctic sediments deposited during the Miocene Climate Optimum (MCO), between approximately 16.9 and 14.7 Ma. While terrestrial organic matter is relatively scarce in MCO marine sections worldwide (Li et al., 2023), this study of coastal sediments from Antarctica aims to evaluate the presence and type of fossil fungi in addition to plant palynomorphs for interpretation of the expression of terrestrial climate and ecosystem change in areas adjacent to the marine depocenters. Characterizing what the landscape, especially in Antarctica, and climate looked like during the MCO is critical for the modeling of future ecological and climatic warming scenarios; however, excepting a few studies focusing on deeper geologic times, the fungi have been overlooked (Harper et al., 2012, 2016). As the warmest interval of the Neogene Period, the MCO has been identified internationally as an analogue for the Intergovernmental Panel on Climate Change Representative Concentration Pathway (IPCC-RCP) 4.5–8.5 warming scenarios, both in terms of strength and speed of warming and in terms of latitudinal effects (Intergovernmental Panel on Climate Change (IPCC), 2014, 2021, 2022, 2023). The global synthesis of terrestrial and marine proxies for paleoclimate and paleoecology of the MCO (Steinthorsdottir et al., 2021) did not include the impact of fossil fungal population in their discussion of biodiversity and carbon cycle dynamics simply because of the lack of fungal records. Yet, fungi are vital components of the terrestrial carbon cycle, and their distribution and diversity provide insights into the local ecosystem structure. The study of fungal assemblages and their ecological requirements can also aid in increasing our knowledge of biodiversity changes during rapid climatic shifts (Nuñez Otaño et al., 2021). Fungal remains, including spores and hyphae, are commonly, but not always, preserved as part of the palynological assemblage contained by rocks and sediments (Elsik, 1996; Shumilovskikh et al., 2021). When present in sedimentary sequences, fossil fungi provide a reliable proxy for investigating how fungi respond to warmer global temperatures (Pound et al., 2022). However, studies examining fungal biodiversity in Neogene sediments are historically rare, and it is only recently that focus has shifted to that group (e.g., Rivero de Dibi et al., 2001; Guimarães et al., 2013; O'Keefe, 2017; Quattrochio et al., 2018; Pound et al., 2019; Romero et al., 2021; Pound et al., 2022). While the majority of fungi are mesophiles (tolerance range 5–35 ∘C, optima 20–30 ∘C), a large number of species are able to grow close to or below 0 ∘C (Dix and Webster, 1995; Robinson, 2001). In order to use fungi for future climatic predictions, we need an in-depth understanding of past fungal community structure and interactions in warmer-than-present conditions. Without these data, it is difficult to predict future changes in this group and how these will affect ecosystems and, as a consequence carbon cycling, for example. Understanding how climate and ecosystems will adapt to future climate change involves modeling future scenarios by making inferences from geological datasets (Dowsett et al., 2016; Hollis et al., 2019). Paleoclimate research, specifically from the Cenozoic (e.g., Pound et al., 2012; Salzmann et al., 2013; Pound and Salzmann, 2017; Holbourn et al., 2010; Birks, 2019; Warny et al., 2009; Feakins et al., 2012) provides an invaluable source of long-term data that can be used to help understand future climate change. The Fungi in a Warmer World (FiaWW) project, of which this paper is a part, aims to remedy this by establishing a database of Miocene fungal assemblage distributions, including ecological and climatological tolerances of assemblage members. The FiaWW project was designed to gather globally distributed fossil fungi data and work towards overcoming the difficulties associated with taxonomic identification whenever possible by building collaboration and implementing intensive training between both modern and paleopalynologists, mycologists, and climate scientists. The ultimate goal of the entire FiaWW project is to produce a novel predictive model for fungal assemblage behavior under future warming events by generating a global dataset of fungal assemblages before, during, and after MCO warming that encompasses equatorial, mid-latitude, and high-latitude sites in both hemispheres. As part of this project, fossil fungi extracted from Miocene age sediment have been documented and assigned to modern fungal taxa. The newly acquired Miocene records provide a unique chance to examine fungal assemblages preserved in marine sediments and can be compared with near-coeval assemblages documented on land (e.g., Thailand, Romero et al., 2021). Such comparisons can demonstrate where fungal assemblages are useful as climate proxies and where they are not, a key piece in the development of this new climate change proxy. Recent studies indicate that fungi can be used in Miocene terrestrial settings in the upper midlatitudes (Pound et al., 2022; Worobiec et al., 2022), midlatitudes (O'Keefe et al., 2021; Romero et al., 2021), and tropics (Guimarães et al., 2013; Romero et al., 2021), but it is unknown whether the proxy can translate into high latitudes.
The results presented herein are the first detailed MCO Antarctic fungal record analyzed. Our hypothesis was that fungal diversity and yield would increase to match our plant pollen and spore record during the warm and humid conditions of the MCO.
ANDRILL's southern McMurdo Sound (SMS) project core hole AND-2A, recovered at 77∘45.488′ S, 165∘16.605′ E (Fig. 2), represents the only complete proximal record of the MCO sampled in Antarctica (Acton et al., 2008). The AND-2A core was drilled from a sea ice platform to a total depth of 1138.54 m below the seafloor (m b.s.f.) and recovered over a kilometer of core, which resulted in ∼ 98 % recovery (Acton et al., 2008; Florindo et al., 2008). The cored section is composed of three distinctive stratigraphic intervals from Lower Miocene to Pleistocene. This research is focusing on the Middle Miocene interval (Fig. 2). Biostratigraphy, magnetostratigraphy, and radiometric dating from this core concluded that an approximately 600 m thick Lower Miocene and Middle Miocene section, which includes the MCO, is disconformably overlain at 224.82 m b.s.f. by a thinner Upper Miocene to Pleistocene section (Acton et al., 2008). The lithology changes throughout the deposits reflect variations in sea level, glacial proximity, and climate (Florindo et al., 2008; Harwood et al., 2009). To conduct a comprehensive palynological analysis of the AND-2A core, the science team at McMurdo station, Antarctica, initially collected 230 palynological samples between the depth of 1108 and 28 m b.s.f. at ∼ 5 m intervals. After a preliminary review of the entire sampled interval, 39 additional samples were selected between the depth 578.24 and 310.07 m b.s.f. to provide a high-definition sampling distribution through the entire time interval of the Miocene Climatic Optimum (MCO). For the fungal study discussed herein, a total of 44 samples were analyzed. This includes the set of 39 high-resolution samples (Table 1, set 1) from the original AND-2A study that were re-analyzed for fungal diversity as fungal spores were not the focus of the previous ANDRILL studies. Because the preliminary evaluation of the samples revealed low recovery in fungi, five additional samples (Table 1, set 2) were requested from the Oregon State University Marine and Geology Repository (OSU-MGR) and processed with an acid-free, more gentle technique (see Sect. 4 for details).
Table 1Detailed information of total samples analyzed from AND-2A core with corresponding depth, sample processing method, and age correlation from Levy et al. (2016). Samples were processed by two separate methods (set 1: acid-based technique; set 2: gentler technique) to evaluate whether or not the acid-based techniques destroyed some of the more fragile fungal palynomorphs.
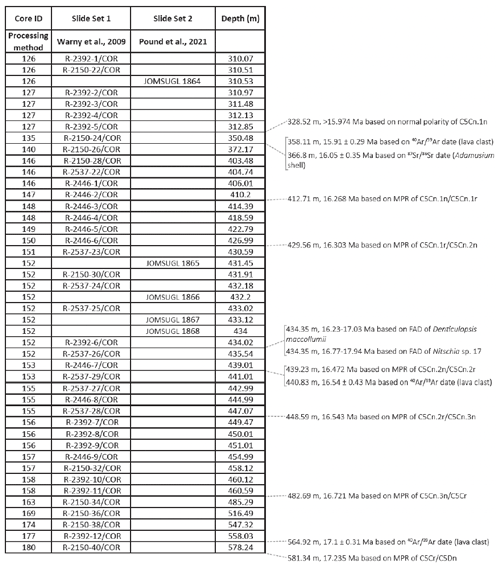
4.1 Palynological techniques
All 39 original samples were processed at Global Geolab Limited (Alberta, Canada) following a palynological technique using HCl-HF demineralization, oxidation, neutralization, and sieving (5 µm mesh) protocols outlined in Warny et al. (2009). For each sample preparation, the available amount of dried sediment was weighed out (weights range from 2.4 to 27.3 g), and the palynomorph samples were spiked with a known quantity of Lycopodium spores to allow for the quantitative assessment of organic-walled microfossil abundance. As mentioned above, considering that the preliminary evaluation of the samples revealed low recovery in fungi, five additional samples (Table 1, set 2) were processed in the OPaL Lab at Morehead State University with a gentler processing method following deflocculation–sieving and swirling–short centrifugation–heavy-density separation protocols (O'Keefe and Eble, 2012; Pound et al., 2021). Mineral-acid-based methods, usually involving HF, have sometimes resulted in damage to non-pollen palynomorphs, including fungi (van Asperen et al., 2016; Pound et al., 2021). Acid-free methods of palynological processing have demonstrated better preservation of fungal palynomorph structure and features (Clarke, 1994; van Asperen et al., 2016) and generally higher recovery (O'Keefe and Eble, 2012), so making sure the initial processing did not influence recovery was essential.
Fungal spores present in the samples were analyzed using an Olympus BX41 microscope under 60× and 100× oil immersion lenses. A database of fungal spores recovered was prepared and documented photographically with a digital Q-Capture camera system mounted on the microscope and then managed with Q-Capture (3.1.1) software. Photomicrographs taken were identified and plotted on a stage-based x–y coordinate grid for geolocation. Z-stacked photomicrographs at 100× were constructed using Helicon Focus® to resolve intricate features. Nearest-living-relative (NLR) techniques rely primarily on presence or absence of taxa when applied to dispersed remains (Utescher et al., 2014). The number of fungal palynomorphs produced compared with the number preserved is very poorly understood (Taylor et al., 2014; Perrotti et al., 2022). For these reasons, numbers of individual fungal palynomorphs were not counted for every slide in this study, rather, fungal presence–absence was documented. Quantitative counts were made for a few samples to gauge a range in relative fungal abundances. However, due to scarcity of fungal remains, standard palynology counts of 300 grains were unobtainable. Instead, fungal spores and Lycopodium spores were counted on every line and on each slide using a transect method to ensure maximum identification of taxa and recovery. Fungal concentration was calculated using the equation of Benninghoff (1962),
where C is concentration (specimens per gram of dried weight of sediment sampled, or gdw−1), Pc is the number of fungal spores counted, Lt is the number of Lycopodium spores per tablet, T is the total number of Lycopodium tablets added per sample, Lc is the number of Lycopodium spores counted, and W is the weight of dried sediment (with dried weight (dw) in grams).
An Excel® table of dominant palynomorphs recovered was prepared, and key taxa were documented photographically. Taxa were separated by morphology using the following criteria: shape, septation, ornamentation, color, color variation, number, type of apertures, and/or attachment scars (Hughes, 1953; Pirozynski and Weresub, 1979; Elsik et al., 1983; Jansonius and Kalgutkar, 2000; Seifert and Gams, 2011; Guarro, 2012; Gulis et al., 2020; Nuñez Otaño et al., 2021). Taxonomic evaluation was completed utilizing established literature, collections curated at the Louisiana State University Center for Excellence in Palynology (CENEX), digital collections compiled by the FiaWW research group, and a variety of databases to identify associations to family or genus.
4.2 Climate reconstruction techniques
4.2.1 Köppen–Geiger climate classes
The lack of modern-day fungal distribution records produces limitations when reconstructing the paleoenvironment. To resolve this shortcoming, fungal remains have been used in recent studies to reconstruct the climate qualitatively by assigning Köppen–Geiger climate classes to known taxa (Beck et al., 2018). The Köppen–Geiger system utilizes threshold values of modern climatology and seasonality of temperature and precipitation to classify terrestrial areas into five main classes (tropical, arid, temperate, continental, and tundra), and 30 sub-types (Beck et al., 2018). Fungal palynomorphs can thus theoretically be assigned Köppen–Geiger climate classes following the methodology of Pound et al. (2022).
4.2.2 CREST
To reconstruct the regional paleoclimate of Antarctica and better capture uncertainties in climate reconstructions during the MCO, pollen data from the Antarctic geologic drilling (ANDRILL) program's AND-2A core in the Ross Sea (Warny et al., 2009), and CREST (Climate Reconstruction SofTware), a Bayesian (probability-based) paleoclimate reconstruction technique (Chevalier, 2022a) was used. Five bioclimatic variables were assessed in detail: mean annual temperature (MAT), mean temperature of warmest quarter (MTWQ), mean temperature of coldest quarter (MTCQ), mean annual precipitation (MAP), and precipitation seasonality (CoV × 100). These results provide a new detailed plant-based representation of paleoclimate for the Ross Sea region during the MCO and enable a Köppen–Geiger class to be determined from the pollen data following Beck et al. (2018) for direct comparison with the fungal-based reconstruction.
Because plants are known as accurate climate proxies (Utescher et al., 2014; Chevalier et al., 2020; Spicer et al., 2021), the CREST reconstruction also provides a means of examining the validity of fungal assemblage proxies, as well as a serving for meaningful support for discussion of possible reasons why fungal assemblage as proxy do not work in every setting. Furthermore, CREST provide suitable quantification of error in Middle Miocene and MCO terrestrial climate reconstructions (Romero et al., 2021;) and produces reconstructions consistent with those of the co-existence approach (Gibson et al., 2022). Taxa lists and nearest living relatives were extracted from the literature (Warny et al., 2009; Feakins et al., 2012) and used as the input for CREST. CREST reconstructions were carried out in R using the “crestr” package (RStudio Team, 2022; Chevalier, 2022a). Original documentation of the “crestr” R code is available with the package code (https://github.com/mchevalier2/crestr, last access: 28 October 2022).
CREST generates a mean and optimized value and 50 % and 95 % (2σ) uncertainties. Here we focus on mean and the 95 % (2σ) confidence interval. All proxies have assumptions and sources of inherent uncertainty. For paleobotany and palynology these are well explored and documented (e.g., Utescher et al., 2014; Chevalier et al., 2020; Spicer et al., 2021). Specifically, for the MCO of Antarctica, it is likely that the vegetation has no exact extant analogue and the propagule-producing species may be extinct. For these reasons, we have chosen to include the 95 % confidence interval in the presentation of our results to aid with comparisons with other paleobotanical and independent proxy paleoclimate reconstructions of the MCO interval in Antarctica. The reconstructed paleoclimate values, including optima, can be found in Table 2. CREST reconstructed paleoclimate estimates are compared to the modern climatology for McMurdo Station (77.8419∘ S, 166.6863∘ E) extracted from WorldClim2.1 (Fick and Hijmans, 2017).
5.1 CREST
CREST requires a minimum of five taxa to work effectively (Chevalier et al., 2014; Chevalier, 2022a). The reconstruction presented herein is based on 13 taxa with a widespread distribution. The palynomorph assemblage used in the paleoclimate reconstruction is thus diverse enough for CREST to use effectively. When compared to the modern-day climate of Antarctica, CREST reconstructs a drastically warmer (by ∼ 28 ∘C), drastically wetter (115 % increase in precipitation), and less seasonal eastern Antarctica during the Miocene Climatic Optimum (Table 2). Details of the reconstruction are presented in Figs. 3 to 7. CREST reconstructs a mean annual temperature (MAT) of 10.3 ∘C (2.0–20.2 ∘C) with summer temperatures (MTWQ) of 16.4 ∘C (9.3–25.3 ∘C) and winter temperatures (MTCQ) of 5.5 ∘C (−5.2–18.6 ∘C). Mean annual precipitation (MAP) is reconstructed at 1145 mm yr−1 (238–2611 mm yr−1) with a seasonality (PS) of 30.0 (CoV × 100) (6.8–68.45). These reconstructions point to a temperate (∘C) Köppen–Geiger climate class with a warm summer (b). Although summer and winter precipitation were not reconstructed, the modest nature of PS would imply a Cfb Köppen–Geiger climate class (Table 2).
Table 2Summary of paleoclimate values reconstructed for southern McMurdo Sound, Antarctica, by CREST on the basis of palynological results from AND-2A.
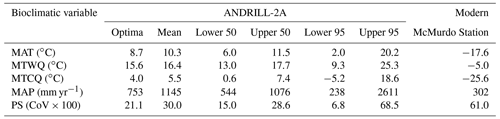
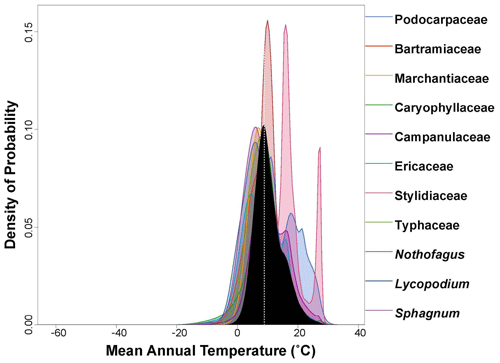
Figure 3Probability density function (PDF) graphical diagnosis of mean annual temperature (MAT) showing the combination of the PDFs of all the plant taxa recorded in the Andrill2-2A sample. The solid black curve represents the posterior MAT reconstruction, and the dotted white line represents the CREST optima.
5.2 The fungal palynological record
The newly acquired fossil fungal record from the AND-2A core shows that fossil fungi are low in both diversity and abundance. Although one processing technique (Pound et al., 2021) is known to be gentler and more optimal for the recovery of fragile palynomorphs, there are no differences observed between the two processed sets, consistent with findings from the Miocene of the UK (Pound et al., 2022). A total of 28 samples are barren, with 16 of those samples appearing consecutively (between the depth of 578.4 and 441.01 m b.s.f.). Only members of the Apiosporaceae, a family that includes a series of genera with simple round brown amerospores ranging in size from 5 to 9 µm, were present within those 16 samples (Plate 1). Due to their simple morphology, the fungal spores recovered could only be identified at the family level. The first recovery of these fungal spores occurred at 439.01 m b.s.f., but the presence of fungi is sporadic between 439.01 and 310.07 m b.s.f., except in a consistent interval from 434.02 to 431.91 m b.s.f. The concentration of fungal spores tabulated from those samples where recovery was greatest is very low, ranging between 72 and 199 fungal spores per gram of dried sediment, providing maximum values for fungal abundances during the MCO in southern McMurdo Sound.
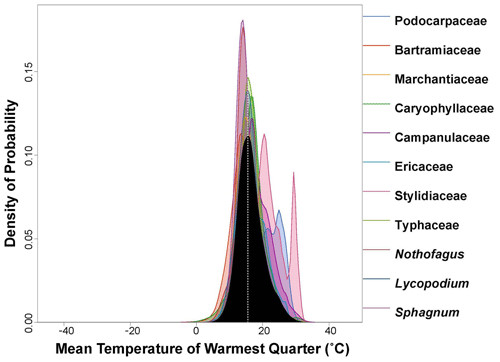
Figure 4PDF graphical diagnosis of mean temperature of warmest quarter (MTWQ) showing the combination of the PDFs of all the plant taxa recorded in the Andrill2-2A sample. The solid black curve represents the posterior MTWQ reconstruction, and the dotted white line represents the CREST optima.
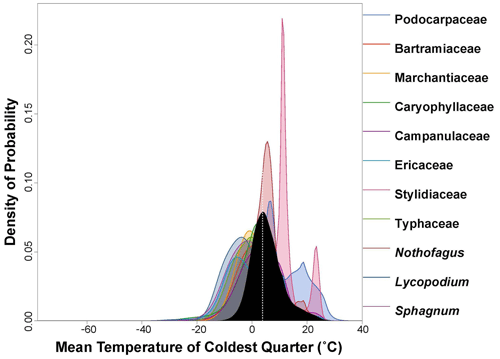
Figure 5PDF graphical diagnosis of mean temperature of coldest quarter (MTCQ) showing the combination of the PDFs of all the plant taxa recorded in the Andrill2-2A sample. The solid black curve represents the posterior MTCQ reconstruction, and the dotted white line represents the CREST optima.
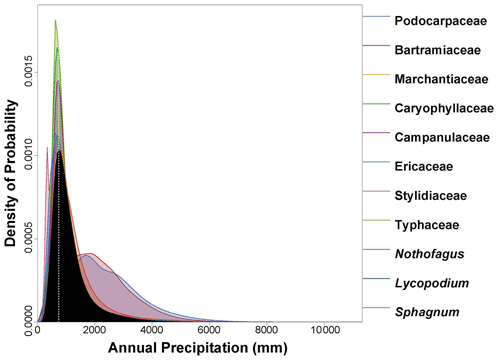
Figure 6PDF graphical diagnosis of mean annual precipitation (MAP) showing the combination of the PDFs of all the plant taxa recorded in the Andrill2-2A sample. The solid black curve represents the posterior MAP reconstruction, and the dotted white line represents the CREST optima.
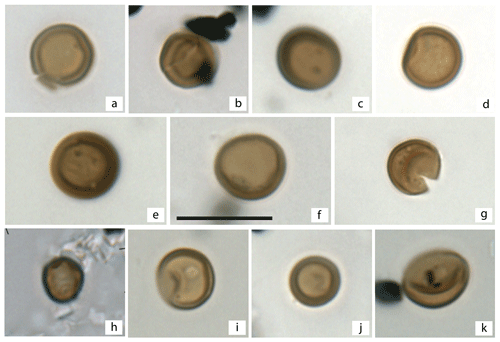
Plate 1Fungal spores recovered from the AND-2A Core, southern McMurdo Sound, Antarctica. (a)–(k) Apiosporaceae. The scale bar is 10 µm.
Ecological information (Põlme et al., 2020; Zanne et al., 2020) and climatological tolerances (Větrovský et al., 2020) for the genera accepted as part of the Apiosporaceae fungal family are summarized in Table 3.
Despite the taxonomic limitations, all lifestyles, substrates, and habitats associated with the Apiosporaceae genera (Table 3) point to a cosmopolitan group present in all environments outside of dry desert conditions, and extant genera within this fungal family are mainly saprotrophic on wood and plant litter but also include plant pathogens found in aquatic habitats (Põlme et al., 2020).
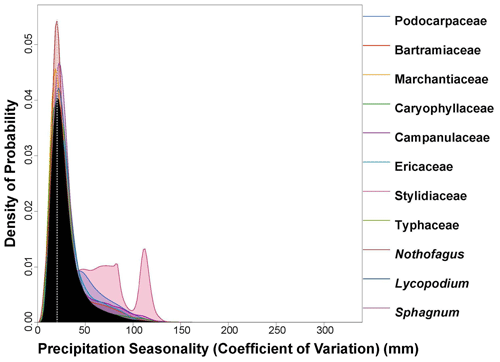
Figure 7PDF graphical diagnosis of precipitation seasonality (CoV × 100) showing the combination of the PDFs of all the plant taxa recorded in the Andrill2-2A sample. The solid black curve represents the posterior Precipitation Seasonality reconstruction, and the dotted white line represents the CREST optima.
5.3 Fungal-spore-based climatological inferences
Despite the limitations noted above, online databases were reviewed for all locations where Apiosporaceae are found today and each location was assigned the associated Köppen–Geiger climate class. This fungal-based reconstruction was made for the 16 samples from SMS that contained fossil fungi. Since each sample contained only Apiosporaceae, their Köppen–Geiger climate class reconstructions were identical. A total of 23 climate classes appear within the reconstruction, including 5 broad classes: A (tropical), B (arid), C (temperate), D (cold), and E (polar), each with their own sub-groups. This means that this specific taxon is found in a broad variety of climates today. Thus, the widely cosmopolitan nature of Apiosporaceae means that for our site the fungi-based climate reconstruction for SMS is of no value. It only shows that the fungi entering the fossil record during the MCO in Antarctica are those tolerant of a wide range of climatic conditions. The same trait was observed for the dinoflagellate assemblages, in which Operculodinium centrocarpum, a known cosmopolitan alga, was the main species able to recolonize the Antarctic coastal waters during the MCO.
6.1 Palynology
This study focused on describing fossil fungal composition from southern McMurdo Sound with a trait-based morphological approach. During the time interval analyzed, an important recolonization of plants was observed at the SMS site (Warny et al., 2009). The most abundant plant palynomorphs recovered indicate a proliferation of woody plants, specifically a variety of Nothofagus species (Southern beech trees) and Podocarpaceae (podocarp conifer). Also observed were other components of the vegetation, including bryophytes (mainly Coptospora spp., moss, Bartramiaceae), Marchantiaceae (liverworts), a Lycopodium (clubmoss) similar to an extant Patagonian species, and angiosperms characteristic of modern subantarctic and austral-alpine environments such as Caryophyllaceae (Colobanthus type, pearlwort), Stylidiaceae (trigger plants), Droseraceae (sundews), Campanulaceae (bell flowers), Ericales (heaths), Poaceae (grasses), and Typhaceae and/or Sparganiaceae (bullrushes, burr reeds).
This new study of the fungal fraction of the SMS site indicates that, while vegetation during the MCO was somewhat abundant and diverse, fungal recovery from Antarctica during the Middle Miocene was sparse and only represented by spores from a single family, Apiosporaceae, of which a member (Spegazzinia) is extant along the Antarctic coast and in southernmost Patagonia today (Musotto et al., 2017). Apiosporaceae are endophytes, parasites, and saprophytes and have a cosmopolitan distribution, except in dry desert climates. This trait and the associated adaptability of the group is probably the reason why they were the one group able to thrive during the brief episode of improved climatic conditions in Antarctica. These fungi were likely associated with the various forms of plant remains (leaves, wood) that thrived during the MCO interval.
Despite the poor recovery, this study provides the first record of fossil fungal spores from Antarctic MCO sediments (Fig. 8). This information is useful for paleoecological inferences concerning relative abundance of decaying plant remains accumulated during favorable climatic conditions of MCO.
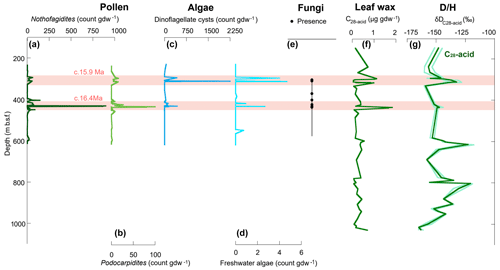
Figure 8Geochemical and palynological results from AND-2A core. (a) Nothofagidites pollen concentration (cc). (b) Podocarpidites pollen cc. (c) Dinoflagellate cyst cc. (d) Freshwater algae cc. (e) Presence of Apiosporaceae fungal spores from this study (note that each dot represents several non-barren samples). (f) Leaf wax abundance. (g) δD, mean (dark green), 1 standard deviation (light green). Pink shading highlights two peaks identified in abundance data (modified after Feakins et al., 2012).
6.2 CREST
The CREST analysis provides a maximum mean annual temperature of 10.3 ∘C and a mean annual precipitation of 1147 mm yr−1 for the warmest intervals of the MCO (Table 2 and Fig. 9). The reconstructed MCO climate of ANDRILL-2A is considerably warmer and wetter than the modern climate of the nearby McMurdo Station. The modern polar-desert conditions at McMurdo have an average annual temperature of −17.6 ∘C with summer averages of −5.0 ∘C and winter averages of −25.6 ∘C. Compared to the modern values, the reconstructed MCO estimates represent a 27.9 ∘C increase in annual average temperature, a 21.4 ∘C increase in summer temperatures, and a 10.5 ∘C increase in winter temperatures. Modern average annual precipitation at McMurdo is low at 302 mm yr−1 and precipitation seasonality is 61.0 (CoV × 100), meaning the MCO reconstruction is 279 % wetter and approximately half as seasonal.
The CREST reconstruction aligns with the interpretation of a warmer, wetter, less seasonal world during the MCO (Steinthorsdottir et al., 2021) and is supported by independent proxy evidence from the Ross Sea and penecontemporaneous records from across Antarctica. Northern high-latitude areas were potentially 14 ∘C warmer than preindustrial times during the MCO (Pound et al., 2012) and it has been suggested that southern high latitudes were similarly warmed (Pagani et al., 2011; Steinthorsdottir et al., 2021). The reconstruction presented here suggests the southern high latitudes experienced enhanced warming with average summer temperatures reconstructed over 20 ∘C warmer and winter temperatures 10 ∘C warmer than preindustrial times. Considered in the Köppen–Geiger climate classification scheme, the MCO is reconstructed as a Cfb climate class (temperate, no dry season, warm summer), as opposed to the EF climate class (polar, frost) of present-day SMS (Beck et al., 2018). The nearest present-day location to SMS with this Köppen–Geiger climate classification is the Snares Islands of New Zealand at 48∘ S (Beck et al., 2018).
The relative warming reconstructed by CREST is supported by various proxy records from the ANDRILL-2A core and beyond. The encroachment of plant taxa associated with lower latitudes today, specifically Nothofagites, has been repeatedly linked to warming during the Neogene (e.g., Lewis et al., 2008; Warny et al., 2009; Anderson et al., 2011; Sangiorgi et al., 2018). The taxonomic composition of terrestrial palynomorphs from the ANDRILL-2A record, including the large increase in the relative abundance of Podocarpidites (podocarp conifer) and Nothofagidites (southern beech) suggests austral summer temperatures reached at least 10 ∘C during the MCO (Warny et al., 2009), similar to average annual temperature estimated from pollen assemblages from ANDRILL-2A of 0–10 ∘C (Feakins et al., 2012). Dinoflagellate species from ANDRILL-2A show a replacement of sea ice indicator taxa by temperate taxa, also indicating similar temperatures within the range of 0 to 11.5 ∘C in the Ross Sea (Warny et al., 2009). These temperatures are close to the CREST reconstructed MAT of 10.3 ∘C. Marine sediments from ANDRILL-2A have yielded plant wax δD that suggest MCO summer temperatures of 7 ∘C on land (Feakins et al., 2012). These summer temperatures are lower than MTWQ (summer) reconstructed by CREST (16.4 ∘C (9.3–25.3 ∘C)) and more comparable to MAT (10.3 ∘C (2.0–20.2 ∘C)).
CREST temperature reconstructions align well with sea surface temperature (SST) reconstructions from the Ross Sea and surrounding regions. SSTs from peak warming during the MCO (ca. 15.7 Ma) suggest a warming of 6–10 ∘C in the Ross Sea (Levy et al., 2016). This is well within the 27.9 ∘C warming reconstructed by CREST for MAT relative to preindustrial times but more comparable with the 10.5 ∘C increase for MTCQ (winter). In addition, TEX86 from ANDRILL-2A from the Ross Sea (Levy et al., 2016) recalibrated with BAYSPAR yields SST estimates of ∼ 11 ∘C (6–26 ∘C) spanning from 20 to 11 Ma, which are within the range of the 95 % confidence interval for CREST MAT and MTWQ (summer) reconstructions.
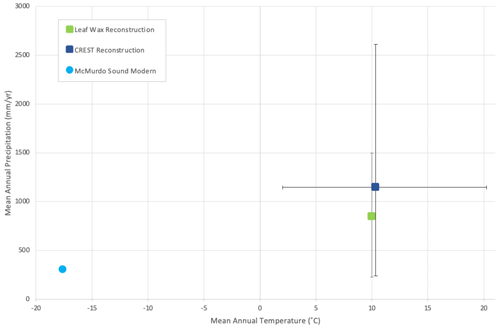
Figure 9Mean annual temperature vs. mean annual precipitation of southern McMurdo Sound, Antarctica. Error bars represent the 95 % (2σ) confidence interval. Squares indicate Miocene climate reconstructed by CREST (this study, dark blue) and leaf wax (Feakins et al., 2012, green), and the circle indicates modern-day climate in southern McMurdo Sound, Antarctica.
Slightly further afield in the Dry Valleys of the Transantarctic Mountains, cypridoidean ostracods and lathridiid beetle fossils yield summer temperatures of 5 ∘C immediately following the Mid-Miocene Climate Transition (MMCT) (14 Ma) (Lewis et al., 2008) more comparable with CREST reconstructed MTCQ (winter) and indicative of post MCO cooling. TEX86 from DSDP Site 269 reveals warm ocean temperatures of up to 14 ∘C persisted off Wilkes Land, East Antarctica (Evangelinos et al., 2020), and at IODP Site U1356A (∼ 60∘ S paleolatitude), the Methylation of Branched Tetraether (MBT)/Cyclization of Branched Tetraether (CBT) method has estimated Miocene Wilkes Land MAT as 12 ∘C for 16.3 to 13.8 Ma (Sangiorgi et al., 2018). These are comparable with the CREST MTWQ (summer) reconstruction.
Penecontemporary evidence from elsewhere in Antarctica provides similar temperature reconstructions. Branched glycerol dialkyl glycerol tetraethers from soil bacteria suggest air temperatures of 12 ∘C ± 1 ∘C for months above freezing for the Miocene with TEX86 Miocene SSTs ranging from 14.3 to 3.9 ∘C (Tibbett et al., 2022), which are similar to CREST reconstructions of mean MTWQ (summer) and MTCQ (winter), respectively. TEX86 BAYSPAR SSTs from IODP Site U1356 (60∘ S) in the Weddell Sea indicate SSTs of 12 ∘C ± 3 ∘C (13.8–10.8 Ma) (Hartman et al., 2018), which is within the 95 % confidence intervals of CREST MAT, MTWQ (summer), and MTCQ (winter). SSTs have also been reconstructed at IODP Site 1171 (48∘30′ S, 149∘6′ E) based on clumped isotopes measured on planktonic foraminiferal calcite yielding 12.7 ∘C ± 1.1 ∘C (Leutert et al., 2020), which are within the 95 % uncertainties for CREST MAT, MTWQ, and MTCQ. The warmest temperatures are reconstructed from offshore ODP Site 1171 (55∘ S) on the South Tasman Rise where TEX86 BAYSPAR indicates SSTs of 22.6 ∘C ± 2.5 ∘C (15.3–12.0 Ma) (Leutert et al., 2020), which is within the 95 % confidence interval for CREST MTWQ (summer).
The goal of this study is to provide a new climate reconstruction using plant-based fossils and to evaluate fungal composition during the MCO in Antarctica. New plant-based paleoclimate reconstructions performed using CREST indicate a warmer and wetter than present paleoclimate that agrees with previous studies completed using various geochemical proxies, with mean annual temperature and precipitation reconstructed at 10.3 ∘C and 1145 mm yr−1, respectively. The plant-based reconstructions define a temperate, no dry season with a warm summer (Cfb) Köppen–Geiger climate classification during the MCO for southern McMurdo Sound. In the present study, the MCO in the Ross Sea region is reconstructed 279 % wetter than modern conditions, representing a relative 843 mm yr−1 increase in precipitation. This increase agrees with precipitation reconstructed from leaf wax hydrogen isotope data from the ANDRILL-2A core (Feakins et al., 2012).
The 44 samples analyzed from the ANDRILL-2A core in southern McMurdo Sound ranged in age from approximately 17 to 15 Ma, but fungal spores were only present from ∼ 16.4 to 15.6 Ma. Results described herein show a poor recovery of fungal spores with a maximum concentration per gram of dried sediment ranging up to ∼ 200, as opposed to a concentration of up to ∼ 900 for pollen, and up to ∼ 2250 for dinoflagellates. Only 36 % of samples analyzed yielded fungal spores or fungal remains, all from a single family, the Apiosporaceae. The presence of this single type, a saprotrophic cosmopolitan family that inhabits substrates rich in both woody and herbaceous plants, some of which are associated with freshwater habitats, supports the assumption that these fungi were able to recolonize some areas of Antarctica during this brief interval of warming and increased moisture availability. Unfortunately, the limited fossil fungi record recovered prevents the use of fungi for fungal-based paleoclimatic reconstruction.
The code used within this work is publicly available here https://cran.r-project.org/web/packages/crestr/index.html (last access: 28 October 2022) and here https://github.com/mchevalier2/crestr (last access: 28 October 2022). An older format is also available at https://doi.org/10.5281/zenodo.6458405 (Chevalier, 2022b).
Data sets used in this study are available upon request.
MP conducted the fungal analyses, MEG conducted all CREST analyses, SW conducted the pollen and spore analyses and advised MP during her MS thesis. NBNO and ICR provided guidance and training on fungal taxonomy. MJP and JMKO are the lead PIs on the FiaWW project and provided guidance to MP and MEG. All authors contributed in various ways to the writing of the paper.
The contact author has declared that none of the authors has any competing interests.
Publisher's note: Copernicus Publications remains neutral with regard to jurisdictional claims made in the text, published maps, institutional affiliations, or any other geographical representation in this paper. While Copernicus Publications makes every effort to include appropriate place names, the final responsibility lies with the authors.
This article is part of the special issue “Advances in Antarctic chronology, paleoenvironment, and paleoclimate using microfossils: Results from recent coring campaigns”. It is not associated with a conference.
This research was supported by a graduate research assistantship from the “Fungi in a Warmer World” (FiaWW) project, jointly funded by NSF/Geo (award no. 2015813) and NERC (award no. NE/V01501X/1) to Jen O'Keefe and Matthew Pound. Samples acquired by the ANDRILL SMS project were provided by the Antarctic Marine Geology Research, which is sponsored by the National Science Foundation. Processing of all palynological samples was funded by a US NSF CAREER grant (award no. ANT-1048343) to Sophie Warny. Additional funding was received from the New Orleans Geological Society Graduate Student Scholarship, LSU CENEX funds, and an LSU AAPG Scholarship.
This research has been supported by the National Science Foundation (grant nos. GEO-2015813 and ANT-1048343) and NERC (grant no NE/V01501X/1).
This paper was edited by Francesca Sangiorgi and reviewed by two anonymous referees.
Acton, G., Crampton, J., Di Vincenzo, G., Fielding, C. R., Florindo, F., Hannah, M., Harwood, D. M., Ishman, S., Johnson, K., Jovane, L., and Levy, R.: Preliminary integrated chronostratigraphy of the AND-2A core, ANDRILL Southern McMurdo Sound project, Terra Antartica 2008–2009, 15, 211–220, 2008.
Anderson, J. B., Warny, S., Askin, R. A., Wellner, J. S., Bohaty, S. M., Kirshner, A. E., Livsey, D. N., Simms, A. R., Smith, T. R., Ehrmann, W., and Lawver, L. A.: Progressive Cenozoic cooling and the demise of Antarctica's last refugium, P. Natl. Acad. Sci. USA, 108, 11356–11360, 2011.
Askin, R. A.: Late Cretaceous–early Tertiary Antarctic outcrop evidence for past vegetation and climates, Antar. Res. S., 56, 61–74, 1992.
Askin, R. A.: Spores and pollen from the McMurdo Sound erratics, Antarctica, Paleobiology and Paleoenvironments of Eocene Rocks: McMurdo Sound, East Antarctica, 76, 161–181, 2000.
Askin, R. A. and Raine, J. I.: Oligocene and Early Miocene terrestrial palynology of the Cape Roberts Drillhole CRP-2/2A, Victoria Land Basin, Antarctica, Terra Antartica, 7, 493–501, 2000.
Askin, R. A. and Ricci, C. A.: Eocene? earliest Oligocene terrestrial palynology of Seymour Island, Antarctica, The Antarctic Region: Geological Evolution and Processes, 993, p. 7, 1997.
Beck, H. E., Zimmermann, N. E., McVicar, T. R., Vergopolan, N., Berg, A., and Wood, E. F.: Present and future Köppen-Geiger climate classification maps at 1-km resolution, Sci. Data, 5, 1–12, 2018.
Benninghoff, W. S.: Calculation of pollen and spore density in sediments by addition of exotic pollen in known quantities, Pollen et Spores, 4, 332–333, 1962.
Birks, H. J. B.: Contributions of Quaternary botany to modern ecology and biogeography, Plant Ecol. Divers., 12, 189–385, 2019.
Chevalier, M.: crestr: an R package to perform probabilistic climate reconstructions from palaeoecological datasets, Clim. Past, 18, 821–844, https://doi.org/10.5194/cp-18-821-2022, 2022a.
Chevalier, M.: mchevalier2/crestr: v1.0.1, Zenodo [code], https://doi.org/10.5281/zenodo.6458405, 2022.
Chevalier, M., Cheddadi, R., and Chase, B. M.: CREST (Climate REconstruction SofTware): a probability density function (PDF)-based quantitative climate reconstruction method, Clim. Past, 10, 2081–2098, https://doi.org/10.5194/cp-10-2081-2014, 2014.
Chevalier, M., Davis, B. A., Heiri, O., Seppä, H., Chase, B.M., Gajewski, K., Lacourse, T., Telford, R. J., Finsinger, W., Guiot, J., and Kühl, N.: Pollen-based climate reconstruction techniques for late Quaternary studies, Earth-Sci. Rev., 210, 103384, https://doi.org/10.1016/j.earscirev.2020.103384, 2020.
Clarke, C.: Differential recovery of fungal and algal palynomorphs versus embryophyte pollen and spores by three processing techniques, Aspects of archaeological palynology: methodology and applications, 29, 53–62, 1994.
DeConto, R. M. and Pollard, D. Rapid Cenozoic glaciation of Antarctica induced by declining atmospheric CO2, Nature, 421, 245–249, 2003.
DeConto, R. M. and Pollard, D.: Contribution of Antarctica to past and future sea-level rise, Nature, 531, 591–597, 2016.
Dix, N. J. and Webster, J.: Fungi of extreme environments, Fungal Ecol., 322–340, https://doi.org/10.1007/978-94-011-0693-1_12, 1995.
Dowsett, H., Dolan, A., Rowley, D., Moucha, R., Forte, A. M., Mitrovica, J. X., Pound, M., Salzmann, U., Robinson, M., Chandler, M., Foley, K., and Haywood, A.: The PRISM4 (mid-Piacenzian) paleoenvironmental reconstruction, Clim. Past, 12, 1519–1538, https://doi.org/10.5194/cp-12-1519-2016, 2016.
Elsik, W. C.: Fungi, in: Palynology: principles and applications, edited by: Jansonius, J. and McGregor, D. C., American Association of Stratigraphic Palynologists Foundation, 1, 293–305, 1996.
Elsik, W. C. and AASP Workgroup on Fossil Fungal Palynologists: Annotated glossary of fungal palynomorphs, AASP Contrib. Ser., 11, 1–35, 1983.
Evangelinos, D., Escutia, C., Etourneau, J., Hoem, F., Bijl, P., Boterblom, W., vaan de Flierdt, T., Valero, L., Flores, J. A., Rodrigues-Tovar, F. J., and Jimenez-Espejo, F. J.: Late Oligocene-Miocene proto-Antarctic circumpolar current dynamics off the Wilkes Land margin, East Antarctica, Glob. Planet. Change, 191, 103221, https://doi.org/10.1016/j.gloplacha.2020.103221, 2020.
Feakins, S. J., Warny, S., and Lee, J. E.: Hydrologic cycling over Antarctica during the Middle Miocene warming, Nat. Geosci., 5, 557–560, 2012.
Fick, S. E. and Hijmans, R. J.: WorldClim 2: New 1-km spatial resolution climate surfaces for global land areas, Int. J. Climatol., 37, 4302–4315, 2017.
Florindo, F., Harwood, D., Levy, R., and SMS Project Science Team: ANDRILL's success during the 4th International Polar Year, Sci. Drill., 6, 29–31, 2008.
Flower, B. P. and Kennett, J. P.: The Middle Miocene climatic transition: East Antarctic ice sheet development, deep ocean circulation and global carbon cycling, Palaeogeogr. Palaeocl., 108, 537–555, 1994.
Fretwell, P., Pritchard, H. D., Vaughan, D. G., Bamber, J. L., Barrand, N. E., Bell, R., Bianchi, C., Bingham, R. G., Blankenship, D. D., Casassa, G., Catania, G., Callens, D., Conway, H., Cook, A. J., Corr, H. F. J., Damaske, D., Damm, V., Ferraccioli, F., Forsberg, R., Fujita, S., Gim, Y., Gogineni, P., Griggs, J. A., Hindmarsh, R. C. A., Holmlund, P., Holt, J. W., Jacobel, R. W., Jenkins, A., Jokat, W., Jordan, T., King, E. C., Kohler, J., Krabill, W., Riger-Kusk, M., Langley, K. A., Leitchenkov, G., Leuschen, C., Luyendyk, B. P., Matsuoka, K., Mouginot, J., Nitsche, F. O., Nogi, Y., Nost, O. A., Popov, S. V., Rignot, E., Rippin, D. M., Rivera, A., Roberts, J., Ross, N., Siegert, M. J., Smith, A. M., Steinhage, D., Studinger, M., Sun, B., Tinto, B. K., Welch, B. C., Wilson, D., Young, D. A., Xiangbin, C., and Zirizzotti, A.: Bedmap2: improved ice bed, surface and thickness datasets for Antarctica, The Cryosphere, 7, 375–393, https://doi.org/10.5194/tc-7-375-2013, 2013.
Gibson, M. E., McCoy, J., O'Keefe, J. M., Nuñez Otaño, N. B., Warny, S., and Pound, M. J.: Reconstructing Terrestrial Paleoclimates: A Comparison of the Co-Existence Approach, Bayesian and Probability Reconstruction Techniques Using the UK Neogene, Paleoceanogr. Paleocl., 37, e2021PA004358, https://doi.org/10.1029/2021PA004358, 2022.
Griener, K. W., Warny, S., Askin, R., and Acton, G.: Early to Middle Miocene vegetation history of Antarctica supports eccentricity-paced warming intervals during the Antarctic icehouse phase, Glob. Planet. Change, 127, 67–78, 2015.
Guarro, J.: Atlas of soil ascomycetes, CBS-KNAW Fungal Biodiversity Centre, 2012.
Guimarães, J. T. F., Nogueira, A. C. R., Bandeira Cavalcante Da Silva Jr., J., Lima Soares, J., and Silveira, R.: Fossil fungi from Miocene sedimentary rocks of the central and coastal Amazon region, North Brazil, J. Paleontol., 87, 484–492, 2013.
Gulick, S. P., Shevenell, A. E., Montelli, A., Fernandez, R., Smith, C., Warny, S., Bohaty, S. M., Sjunneskog, C., Leventer A., Frederick, B., and Blankenship, D. D.: Initiation and long-term instability of the East Antarctic Ice Sheet, Nature, 552, 225–229, 2017.
Gulis, V., Marvanová, L., and Descals, E.: An illustrated key to the common temperate species of aquatic hyphomycetes, Methods to study litter decomposition: a practical guide, 223–239, 2020.
Harper, C. J., Bomfleur, B., Decombeix, A. L., Taylor, E. L., Taylor, T. N., and Krings, M.: Tylosis formation and fungal interactions in an Early Jurassic conifer from northern Victoria Land, Antarctica, Rev. Palaeobot. Palynol., 175, 25–31, 2012.
Harper, C., Taylor, T., Krings, M., and Taylor, E.: Structurally preserved fungi from Antarctica: Diversity and interactions in late Palaeozoic and Mesozoic polar forest ecosystems, Antarct. Sci., 28, 153–173, https://doi.org/10.1017/S0954102016000018, 2016.
Hartman, J. D., Sangiorgi, F., Salabarnada, A., Peterse, F., Houben, A. J. P., Schouten, S., Brinkhuis, H., Escutia, C., and Bijl, P. K.: Paleoceanography and ice sheet variability offshore Wilkes Land, Antarctica – Part 3: Insights from Oligocene–Miocene TEX86-based sea surface temperature reconstructions, Clim. Past, 14, 1275–1297, https://doi.org/10.5194/cp-14-1275-2018, 2018.
Harwood, D., Florindo, F., Talarico, F., Levy, R., Kuhn, G., Naish, T., Niessen, F., Powell, R., Pyne, A., and Wilson, G.: Antarctic drilling recovers stratigraphic records from the continental margin, Eos, 90, 90–91, 2009.
Holbourn, A., Kuhnt, W., Schulz, M., Flores, J. A., and Andersen, N.: Orbitally-paced climate evolution during the Middle Miocene Monterey carbon-isotope excursion, Earth Planet. Sc. Lett., 261, 534–550, 2007.
Holbourn, A., Kuhnt, W., Regenberg, M., Schulz, M., Mix, A., and Andersen, N.: Does Antarctic glaciation force migration of the tropical rain belt?, Geology, 38, 783–786, 2010.
Holbourn, A., Kuhnt, W., Kochhann, K. G., Matsuzaki, K. M., and Andersen, N.: Middle Miocene climate–carbon cycle dynamics: Keys for understanding future trends on a warmer Earth?, in: Understanding the Monterey Formation and Similar Biosiliceous Units across Space and Time, edited by: Aiello, I. W., Barron, J. A., and Ravelo, A. C., Special paper of the Geological Society of America, Vol. 556, 96–111, https://doi.org/10.1130/2022.2556(05), 2022.
Hollis, C. J., Dunkley Jones, T., Anagnostou, E., Bijl, P. K., Cramwinckel, M. J., Cui, Y., Dickens, G. R., Edgar, K. M., Eley, Y., Evans, D., Foster, G. L., Frieling, J., Inglis, G. N., Kennedy, E. M., Kozdon, R., Lauretano, V., Lear, C. H., Littler, K., Lourens, L., Meckler, A. N., Naafs, B. D. A., Pälike, H., Pancost, R. D., Pearson, P. N., Röhl, U., Royer, D. L., Salzmann, U., Schubert, B. A., Seebeck, H., Sluijs, A., Speijer, R. P., Stassen, P., Tierney, J., Tripati, A., Wade, B., Westerhold, T., Witkowski, C., Zachos, J. C., Zhang, Y. G., Huber, M., and Lunt, D. J.: The DeepMIP contribution to PMIP4: methodologies for selection, compilation and analysis of latest Paleocene and early Eocene climate proxy data, incorporating version 0.1 of the DeepMIP database, Geosci. Model Dev., 12, 3149–3206, https://doi.org/10.5194/gmd-12-3149-2019, 2019.
Hughes, S. J.: Conidiophores, conidia, and classification, Can. J. Bot., 31, 577–659, 1953.
Intergovernmental Panel on Climate Change (IPCC): Climate Change 2014: Synthesis Report, in: Contribution of Working Groups I, II and III to the Fifth Assessment Report of the Intergovernmental Panel on Climate Change, edited by: Pachauri, R. K. and Meyer, L. A., Geneva, 151, 2014.
Intergovernmental Panel on Climate Change (IPCC): Climate Change 2021: The Physical Science Basis, in: Contribution of Working Group I to the Sixth Assessment Report of the Intergovernmental Panel on Climate Change, edited by: Zhai, V. P., Pirani, A., Connors, S. L., Péan, C., Berger, S., and Caud, N., Cambridge University Press, 2021.
Intergovernmental Panel on Climate Change (IPCC): Climate Change 2022: Impacts, Adaptation, and Vulnerability, in: Contribution of Working Group II to the Sixth Assessment Report of the Intergovernmental Panel on Climate Change, edited by: Portner, H. O., Roberts, D. C., Tignor, M., Poloczanska, E. S., Mintenbeck, K., Alegría, A., Craig, M., Langsdorf, S., Loschke, S., Moller, V., Okem, A., and Rama, B., Cambridge University Press, 2022.
Intergovernmental Panel on Climate Change (IPCC): Climate Change 2023: Climate Change 2023: Synthesis Report. A Report of the Intergovernmental Panel on Climate Change in: Contribution of Working Groups I, II and III to the Sixth Assessment Report of the Intergovernmental Panel on Climate Change, edited by: Core Writing Team, Lee, H., and Romero, J., IPCC, Geneva, Switzerland, in press, https://doi.org/10.59327/IPCC/AR6-9789291691647.001, 2023.
Jansonius, J. and Kalgutkar, R. M.: Redescription of some fossil fungal spores, Palynology, 24, 37–47, 2000.
Lear, C. H., Elderfield, H., and Wilson, P. A.: Cenozoic deep-sea temperatures and global ice volumes from in benthic foraminiferal calcit, Science, 287, 269–272, 2000.
Leutert, T. J., Auderset, A., Martínez-García, A., Modestou, S., and Meckler, A. N.: Coupled Southern Ocean cooling and Antarctic ice sheet expansion during the Middle Miocene, Nat. Geosci., 13, 634–639, 2020.
Levy, R. H., Harwood, D. M., Florindo, F., DeConto, R., von Eynatten, H., Fielding, C., Field, B., Gasson, G., Golledge, N., Kuhn, G., McKay, R., Naish, T., Olney, M., Pollard, D., Sangiorgi, F., Schouten, S., Warny, S., Willimott, V., and SMS Science Team: Threshold response of Antarctic Ice Sheets to atmospheric CO2 variations during the Early to mid-Miocene, P. Natl. Acad. Sci. USA, 113, 3453–3458, 2016.
Lewis, A. R., Marchant, D. R., Ashworth, A. C., Hedenäs, L., Hemming, S. R., Johnson, J. V., Leng, M. J., Machlus, M. L., Newton, A. E., Raine, J. I., and Willenbring, J. K.: Mid-Miocene cooling and the extinction of tundra in continental Antarctica, P. Natl. Acad. Sci. USA, 105, 10676–10680, 2008.
Li, Z., Zhang, Y. G., Torres, M., and Mills, B. J.: Neogene burial of organic carbon in the global ocean, Nature, 613, 90–95, 2023.
Musotto, L. L., Borromei, A. M., Bianchinotti, M. V., Coronato, A., Menounos, B., Osborn, G., and Marr, R.: Postglacial environments in the southern coast of Lago Fagnano, central Tierra del Fuego, Argentina, based on pollen and fungal microfossils analyses, Rev. Palaeobot. Palynol., 238, 43–54, 2017.
Nuñez Otaño, N. B., Bianchinotti, M. V., and Saparrat, M. C.: Palaeomycology: a modern mycological view of fungal palynomorphs, Geol. Soc. Lond. Spec. Publ., 511, 91–120, 2021.
O'Keefe, J. M.: Fungal palynomorphs from the Miocene Heath Formation, Tumbes Province, Perú, Palynology, 41, 309–326, 2017.
O'Keefe, J. M. K. and Eble, C. F.: A comparison of HF-based and non-HF-based palynology processing techniques in clay-rich lignites from the Claiborne Group, upper Mississippi Embayment, United States, Palynology, 36, 116–130, 2012.
O'Keefe, J., Romero, I., Pound, M. J., Nuñez Otano, N. B., Warny, S., Gibson, M., Pilie, M., Spears, T., McCoy, J., Faairchild, C. J., and Jones, S.: Fungi in a Warmer World: Development and Application of a New Paleoecological and Paleoclimatological Proxy, Geol. Soc. Am. Abstr. Prog., AGU Fall Meeting 2021, New Orleans, LA, 13–17 December 2021, ID: B55L-1332, 2021.
Pagani, M., Huber, M., Liu, Z., Bohaty, S. M., Henderiks, J., Sijp, W., Krishnan, S., and DeConto, R. M.: The role of carbon dioxide during the onset of Antarctic glaciation, Science, 334, 1261–1264, 2011.
Perrotti, A. G., Ramiadantsoa, T., O'Keefe, J., and Nuñez Otaño, N. B.: Uncertainty in coprophilous fungal spore concentration estimates, Front. Ecol. Evol., 10, 1238, https://doi.org/10.3389/fevo.2022.1086109, 2022.
Pirozynski, K. A. and Weresub, L. K.: Classification and nomenclature of fossil fungi, Whole fungus; the sexual-asexual synthesis, in: Whole Fungus: the Sexual-Asexual Synthesis, Proceedings of th 2nd International Mycological Conference, edited by: Kendrick, B., University of Calgary, Alberta. Published by the National Museum of Natural Sciences, National Museums of Canada and the Kananaskis Foundation), 653–688, 1979.
Pollard, D. and DeConto, R. M.: Modelling West Antarctic ice sheet growth and collapse through the past five million years, Nature, 458, 329–332, 2009.
Põlme, S., Abarenkov, K., Henrik Nilsson, R., et al.: FungalTraits: a user-friendly traits database of fungi and fungus-like stramenopiles, Fungal Divers., 105, 1–16, https://doi.org/10.1007/s13225-020-00466-2, 2020.
Pound, M. J. and Salzmann, U.: Heterogeneity in global vegetation and terrestrial climate change during the late Eocene to early Oligocene transition, Sci. Rep., 7, 1–12, 2017.
Pound, M. J., Haywood, A. M., Salzmann, U., and Riding, J. B.: Global vegetation dynamics and latitudinal temperature gradients during the Mid to Late Miocene (15.97–5.33 Ma), Earth-Sci. Rev., 112, 1–22, 2012.
Pound, M. J., O'Keefe, J. M., Otaño, N. B. N., and Riding, J. B.: Three new Miocene fungal palynomorphs from the Brassington Formation, Derbyshire, UK, Palynology, 43, 596–607, 2019.
Pound, M. J., O'Keefe, J. M., and Marret, F.: An overview of techniques applied to the extraction of non-pollen palynomorphs, their known taphonomic issues and recommendations to maximize recovery, Geol. Soc. Lond. Spec. Publ., 511, 63–76, 2021.
Pound, M. J., Nuñez Otaño, N. B., Romero, I. C., Lim, M., Riding, J. B., and O'Keefe, J. M.: The fungal ecology of the Brassington Formation (Middle Miocene) of Derbyshire, United Kingdom, and a new method for palaeoclimate reconstruction, Front. Ecol. Evol., 711, 947623, https://doi.org/10.3389/fevo.2022.947623, 2022.
Quattrocchio, M. E., Olivera, D. E., Martínez, M. A., Ponce, J. J., and Carmona, N. B.: Palynofacies associated to hyperpycnite deposits of the Miocene, Cabo Viamonte Beds, Austral Basin, Argentina, Facies, 64, 22, https://doi.org/10.1007/s10347-018-0535-2, 2018.
RStudio Team: RStudio: Integrated Development Environment for R. RStudio, PBC, Boston, http://www.rstudio.com/ (last access: 28 October 2022), 2022.
Raine, J. I.: Terrestrial palynomorphs from Cape Roberts Project drillhole CRP-1, Ross Sea, Antarctica, Terra Ant. Reports, 5, 539–548, 1998.
Rivero de Dibi, L. R., de Sarmiento, M. R., and de Cabrera, J. D.: Esporas de hongos de la Formación Anta (Grupo Orán), Mioceno Medio, provincia de Salta, Argentina, Acta geológica lilloana, 257–264, 2001.
Robinson, C. H.: Cold adaptation in Arctic and Antarctic fungi, New Phytol., 15, 341–353, https://doi.org/10.1046/j.1469-8137.2001.00177.x, 2001.
Romero, I. C., Nuñez Otaño, N. B., Gibson, M. E., Spears, T. M., Fairchild, C. J., Tarlton, L., Jones, S., Belkin, H. E., Warny, S., Pound, M. J., and O'Keefe, J. M.: First record of fungal diversity in the tropical and warm-temperate Middle Miocene Climate Optimum forests of Eurasia, Front. Forest. Glob. Change, 4, 768405, https://doi.org/10.3389/ffgc.2021.768405, 2021.
Salzmann, U., Dolan, A. M., Haywood, A. M., Chan, W. L., Voss, J., Hill, D. J., Abe-Ouchi, A., Otto-Bliesner, B., Bragg, F. J., Chandler, M. A., and Contoux, C.: Challenges in quantifying Pliocene terrestrial warming revealed by data–model discord, Nat. Clim. Change, 3, 969–974, 2013.
Sangiorgi, F., Bijl, P. K., Passchier, S., Salzmann, U., Schouten, S., McKay, R., Cody, R. D., Pross, J., Van de Flierdt, T., Bohaty, S. M., and Levy, R.: Southern Ocean warming and Wilkes Land ice sheet retreat during the mid-Miocene, Nat. Commun., 9, 317, https://doi.org/10.1038/s41467-017-02609-7, 2018.
Seifert, K. A. and Gams, W.: The genera of Hyphomycetes–2011 update, Persoonia-Molecular Phylogeny and Evolution of Fungi, 27, 119–129, 2011.
Shackleton, N. J.: Paleotemperature history of the Cenozoic and the initiation of Antarctic glaciation: oxygen and carbon isotope analyses in DSDP Sites 277, 279, and 281, Initial Report, Deep-Sea Drill. Proj., 29, 743–755, 1975.
Shevenell, A. E., Kennett, J. P., and Lea, D. W.: Middle Miocene Southern ocean cooling and Antarctic cryosphere expansion, Science, 305, 1766–1770, 2004.
Shumilovskikh, L., O'Keefe, J. M., and Marret, F.: An overview of the taxonomic groups of non-pollen palynomorphs, Geol. Soc. Lond. Spec. Publ., 511, 13–61, 2021.
Spicer, R. A., Yang, J., Spicer, T. E., and Farnsworth, A.: Woody dicot leaf traits as a palaeoclimate proxy: 100 years of development and application, Palaeogeogr. Palaeocl., 562, 110138, https://doi.org/10.1016/j.palaeo.2020.110138, 2021.
Steinthorsdottir, M., Coxall, H. K., De Boer, A. M., Huber, M., Barbolini, N., Bradshaw, C. D., Burls, N. J., Feakins, S. J., Gasson, E., Henderiks, J., and Holbourn, A. E.: The Miocene: the future of the past, Paleoceanogr. Paleocl., 36, e2020PA004037, https://doi.org/10.1029/2020PA004037, 2021.
Stickley, C. E., Brinkhuis, H., Schellenberg, S. A., Sluijs, A., Röhl, U., Fuller, M., Grauert, M., Huber, M., Warnaar, J., and Williams, G. L.: Timing and nature of the deepening of the Tasmanian Gateway, Paleoceanography, 19, 4, https://doi.org/10.1029/2004PA001022, 2004.
Taylor, T. N., Krings, M., and Taylor, E. L.: Fossil fungi, Academic Press, 2014.
Tibbett, E. J., Warny, S., Tierney, J. E., Wellner, J. S., and Feakins, S. J.: Cenozoic Antarctic Peninsula temperatures and glacial erosion signals from a multi-proxy biomarker study, Paleoceanogr. Paleocl., 37, e2022PA004430, https://doi.org/10.1029/2022PA004430, 2022.
Utescher, T., Bruch, A. A., Erdei, B., François, L., Ivanov, D., Jacques, F. M. B., Kkern, A. K., Mosbrugger, V., and Spicer, R. A.: The coexistence approach – theoretical background and practical considerations of using plant fossils for climate quantification, Palaeogeogr. Palaeocl., 410, 58–73, 2014.
van Asperen, E. N., Kirby, J. R., and Hunt, C. O.: The effect of preparation methods on dung fungal spores: Implications for recognition of megafaunal populations, Rev. Palaeobot. Palynol., 229, 1–8, 2016.
Větrovský T., Morais D., Kohout P., Lepinay C., Algora Gallardo C., Awokunle Hollá S., Doreen Bahnmann B., Bílohnědá K., Brabcová V., D'Alò F., Human Z.R., Jomura M., Kolařík M., Kvasničková J., Lladó S., López-Mondéjar R., Martinović T., Mašínová T., Meszárošová L., Michalčíková L., Michalová T., Mundra S., Navrátilová D., Odriozola I., Piché-Choquette S., Štursová M., Švec K., Tláskal V., Urbanová M., Vlk L., Voříšková J., Žifčáková L., and Baldrian P.: GlobalFungi, a global database of fungal occurrences from high-throughput-sequencing metabarcoding studies, Sci. Data, 7, 228, https://doi.org/10.1038/s41597-020-0567-7, 2020.
Warny, S. and Askin, R. R.: Vegetation and organic-walled phytoplankton at the end of the Antarctic greenhouse world: Latest Eocene cooling events, in: Tectonic, climatic, and cryospheric evolution of the Antarctic peninsula, 63, 193–210, 2011a.
Warny, S. and Askin, R.: Last remnants of Cenozoic vegetation and organic-walled phytoplankton in the Antarctic Peninsula's icehouse world, in: Tectonic, climatic, and cryospheric evolution of the Antarctic peninsula, 63, 167–192, 2011b.
Warny, S., Askin, R. A., Hannah, M. J., Mohr, B. A., Raine, J. I., Harwood, D. M., Florindo, F., and SMS Science Team: Palynomorphs from a sediment core reveal a sudden remarkably warm Antarctica during the Middle Miocene, Geology, 37, 955–958, 2009.
Warny, S., Kymes, C. M., Askin, R., Krajewski, K. P., and Tatur, A.: Terrestrial and marine floral response to latest Eocene and Oligocene events on the Antarctic Peninsula, Palynology, 43, 4–21, 2019.
Worobiec, G., Worobiec, E., Gedl, P., Kasinski, J. R., Peryt, D., and Widera, M.: Terrestrial-aquatic wood-inhabiting ascomycete Potamomyces from the Miocene of Poland, Acta Palaeontol. Pol., 67, 737–744, https://doi.org/10.4202/app.00976.2022, 2022.
You, Y., Huber, M., Müller, R. D., Poulsen, C. J., and Ribbe, J.: Simulation of the Middle Miocene Climate Optimum, Geophys. Res. Lett., 36, L04702, https://doi.org/10.1029/2008GL036571, 2009.
Zachos, J. C., Dickens, G. R., and Zeebe, R. E.: An early Cenozoic perspective on greenhouse warming and carbon-cycle dynamics, Nature, 451, 279–283, 2008.
Zachos, J., Pagani, M., Sloan, L., Thomas, E., and Billups, K.: Trends, rhythms, and aberrations in global climate 65 Ma to present, Science, 292, 686–693, 2001.
Zanne, A. E., Abarenkov, K., Afkhami, M. E., Aguilar-Trigueros, C. A., Bates, S., Bhatnagar, J. M., Busby, P. E., Christian, N., Cornwell, W. K., Crowther, T. W., and Flores-Moreno, H.: Fungal functional ecology: bringing a trait-based approach to plant-associated fungi, Biol. Rev., 95, 409–433, 2020.
- Abstract
- Background: the Antarctic Miocene Climatic Optimum record
- The status of Miocene fungal records
- Materials
- Methods
- Results
- Discussion
- Conclusions
- Code availability
- Data availability
- Author contributions
- Competing interests
- Disclaimer
- Special issue statement
- Acknowledgements
- Financial support
- Review statement
- References
- Abstract
- Background: the Antarctic Miocene Climatic Optimum record
- The status of Miocene fungal records
- Materials
- Methods
- Results
- Discussion
- Conclusions
- Code availability
- Data availability
- Author contributions
- Competing interests
- Disclaimer
- Special issue statement
- Acknowledgements
- Financial support
- Review statement
- References