the Creative Commons Attribution 4.0 License.
the Creative Commons Attribution 4.0 License.
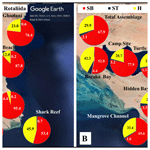
Benthic foraminifers in coastal habitats of Ras Mohamed Nature Reserve, southern Sinai, Red Sea, Egypt
Ahmed M. BadrElDin
The Red Sea is globally recognized for its exceptional diversity of marine environments despite relatively high salinities (39–41). Unfortunately, as elsewhere, anthropogenic activities are degrading coastal environments, including coral reefs. We examined foraminiferal distributions to assess the ecological status of coral reef environments in the coastal area of the Ras Mohamed Nature Reserve (RMNR) in the southern Sinai coastal region. Sediment samples were collected in mangrove, shallow-lagoon, nearshore, and coral reef habitats. Overall, 95 species were recorded. Five benthic foraminiferal species that host algal symbionts dominated the assemblages, representing ∼60 % of the assemblages, along with one porcelaneous heterotrophic species (Quinqueloculina seminulum; ∼8 %). The symbiont-bearing species were three porcelaneous forms (Amphisorus hemprichii, Peneroplis pertusus, and P. planatus) and two hyaline taxa (Amphistegina lobifera and A. lessonii). Peneroplis and Amphisorus dominated shallow-bay and mangrove channel assemblages, where carbonate sediments predominated, whereas Amphistegina were most abundant in reef sediments. The results of diversity and heterogeneity indices, including the Shannon–Wiener index (H′), the Simpson index (D), and the evenness index (E), were remarkably consistent. The foraminiferal assemblages are characterized by low diversity (H′), low evenness, and often high dominance (D). Values of the FoRAM Index consistently exceeded four, indicating water quality suitable for carbonate production and accretion. Relatively high percentages of foraminifers were collected live (stained), with up to 18 % of the total assemblage in some localities. The low α-Fisher indices and commonly observed “abnormal” peneroplid and soritid specimens reflect that these metrics must be used with caution when assessing tropical–subtropical shallow-water assemblages in hypersaline environments. Benthic species that host algal symbionts represent a tiny fraction of total numbers of benthic species, yet photosynthesis by the algal symbionts allows the dominance of those few in clear, shallow, reef-associated waters where particulate food is limited. In addition, morphological variability is extremely common in peneroplids and soritids for reasons not fully understood.
- Article
(9288 KB) - Full-text XML
- BibTeX
- EndNote
The Government of Egypt is committed to conserving its marine resources for sustainable development. Egypt is 1 of the 22 early-starter countries presenting national reviews in the first High-Level Political Forum (HLPF) since the adoption of Agenda 2030 and the Sustainable Developments Goals (SDGs), championing the course for the future. Sustainable Development Goal 14 aims to conserve and sustainably use the oceans, seas, and marine resources for future development (National Voluntary Review, 2016).
Coral reefs are among the most valuable ecosystems on Earth, thanks to their remarkable biological diversity, in addition to economic and environmental services provided to millions of people. Anthropogenic activities along coastal areas are stressing coral reef ecosystems worldwide through pollution, coastline development, sedimentation, overfishing, and other activities (e.g., Bryant et al., 1998; Pandolfi et al., 2003; Eddy et al., 2021).
The Red Sea coastal areas are experiencing rapid urbanization associated with petroleum production and other industries, including tourism, which is a major component of the economy of surrounding countries (Schwartz, 2005; Jobbins, 2006). Coral reefs near urban and industrial centers have been damaged by many activities in watersheds and coastal zones, including clearing of coastal vegetation (e.g., mangroves), landfilling and dredging, port activities, and discharge of sewage and other pollutants, in addition to tourism (e.g., Wilkinson, 2008; Hulver et al., 2022). Tourism provides Egypt with its main source of foreign revenue. Hundreds of thousands of jobs are related directly or indirectly to touristic activities, including along the Red Sea coastal area. Degradation of the marine and coastal environments is the direct effect of environmental disturbance (Naumann et al., 2015), which reduces the potential for sustainable development (PERSGA, 2010).
The Sinai coastal area is noted for its fabulous coral reefs, particularly in the Ras Mohamed Nature Reserve (RMNR). As tourism and related human activities continue to increase in this area, efforts to protect the reefs remain a top environmental concern for the government of Egypt. Moreover, the coral reefs of RMNR may act as stepping stones for gene flow, connecting populations in the Red Sea more generally (Turak et al., 2007). This area provides essential sources of coral recruits for the recovery of coastal and nearshore coral populations that have been damaged in recent decades. To promote sustainable management, additional approaches are essential to evaluate the status and sustainability of Egypt's coral reef ecosystems.
The phylum Foraminifera represents the most abundant shelled organisms in marine and brackish water habitats worldwide and are important components of marine sediments (e.g., Murray, 1973, 2006, 2014). Reef-dwelling and other coastal benthic foraminifers are easy to collect, and their shells are generally sufficiently abundant to provide an adequate statistical base, even in small-volume samples. Foraminifers have relatively short life cycles, are sensitive to environmental changes, and react quickly to environmental stresses (Hallock et al., 2003; Donnici et al., 2012; Bergamin et al., 2019). Benthic foraminifers have long been used in paleoenvironmental studies and are widely used for ecological assessment, providing early-warning indicators for environmental hazards worldwide (e.g., Schönfeld et al., 2012; Prazeres et al., 2020). In recent decades, studies of foraminiferal assemblages have contributed to understanding of environmental problems in coastal marine ecosystems (e.g., Koukousioura et al., 2011; Sabbatini et al., 2014). With developments in biotic indices, studies of benthic foraminifers are increasingly applied in assessments of ecological quality in marine and transitional waters (O'Brien et al., 2021, and references therein).
Hallock et al. (2003) developed a numerical index for relating water quality to coral reef health known as the Foraminifera in Reef Assessment and Monitoring Index (FoRAM Index). This index quantifies the suitability of an environment to support calcifying organisms that host algal endosymbionts (i.e., many coral and larger benthic foraminiferal taxa) and, thus, the accretion of carbonate sediments including those that form coral reefs. Larger benthic foraminifers (LBFs) contain algal endosymbionts whose photosynthetic activity aids in calcification and organic carbon production for foraminifers in the same way that Symbiodiniaceae are beneficial for coral (Hallock, 1999). The endosymbionts limit the occurrence of algal symbiont-bearing foraminifers and coral to the photic zone, where they thrive in clear, nutrient-poor waters (Hallock et al., 2003). Therefore, the abundance of symbiont-bearing foraminifers should parallel coral abundance if water quality is a major environmental control. The FoRAM Index has been applied successfully in many coral reef environmental studies, including Australia (Uthicke and Nobes, 2008; Uthicke et al., 2010), Florida, USA (Williams, 2009), Puerto Rico (Oliver et al., 2014), Brazil (Kelmo and Hallock, 2013), Indonesia (Gitaputri et al., 2013), the Maldives (Pisapia et al., 2017), and elsewhere (see Prazeres et al., 2020, and references therein). Moreover, the FoRAM Index has been applied in non-reefal environments such as subtropical estuaries (Carnahan et al., 2009; Narayan and Pandolfi, 2010) and the eastern Mediterranean Sea (Koukousioura et al., 2011).
This study explores three primary questions. (1) What benthic foraminiferal taxa thrive in coastal habitats of RMNR? (2) Do foraminiferal assemblages indicate that water quality supports the predominance of algal symbiont-bearing taxa? (3) How does the FoRAM Index compare with common diversity indices when working in coral reef ecosystems? This study is focused on distribution, density, and diversity of benthic foraminiferal assemblages associated with reef-associated ecosystems of Egyptian Red Sea coastal area as part of a more extensive project comparing foraminiferal assemblages in the southern Red Sea (Al Bawdi island, Yemen; BadrElDin et al., 2022), northern Red Sea (Ras Mohamed Nature Reserve, Egypt; the current work), and southeastern Mediterranean Sea (Abu Qir coastal area, Egypt; BadrElDin and Hallock, 2022, 2023).
1.1 Regional setting
The Red Sea is a “baby ocean” that was born as a rift basin during the Late Oligocene, with an age of ∼25 Ma (Bosworth, 2015). Created by slow seafloor spreading and flooding during the middle Miocene (Taviani, 1998), the western boundary of the Arabian plate includes the Red Sea and the Gulf of Aqaba–Dead Sea transform fault, with a northeasterly direction of movement (Rasul et al., 2015). The length of the Red Sea is ∼2000 km, with an average width of ∼280 km (Parker et al., 2012). The waterbody bifurcates into the Gulf of Aqaba in the east and the Gulf of Suez in the west. In the north, the Red Sea was detached from the Mediterranean Sea during the Pleistocene and then was reconnected in 1869 by the opening of the Suez Canal. The Red Sea is connected to the Gulf of Aden in the south through Bab-el-Mandeb strait (Siddall et al., 2004).
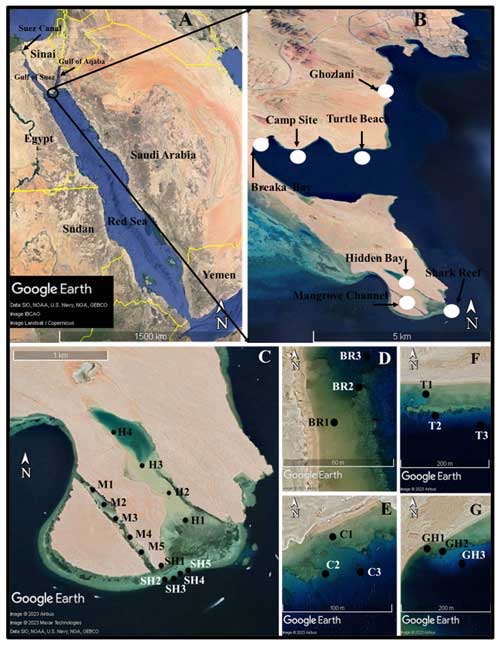
Figure 1Maps (© Google) showing the geographical location of Ras Mohamed Nature Reserve coastal area (a, b) and sampling sites (c–g).
The southern Sinai is characterized by an arid climate with negligible precipitation. Air temperatures range from 15–35 °C in winter and from 20–45 °C in summer (Parker et al., 2012). Water temperatures fluctuate between 20 and 27 °C, and the evaporation rate is about 2000 mm per year, resulting in relatively high salinities (39–41 psu). The tidal regime is micro-tidal, with 0.6 m tides in the gulfs of Aqaba and Suez (Parker et al., 2012). The current phase of coral reef growth in the Red Sea is believed to have begun about 6000 years ago on the Sudanese coast (Braithwaite, 1982).
The Ras Mohamed Nature Reserve (RMNR) is located at the southern tip of the Sinai Peninsula, where the Red Sea bifurcates into the gulfs of Aqaba and Suez (Fig. 1a–b). Declared a protected national nature reserve (by law 120, 1983), the reserve includes a mainland sector dominated by siliciclastic sedimentation and an elongated peninsula of mixed siliciclastic and carbonate sediments (Nasr et al., 1997). The peninsula serves as an eastern border to an extensive reefal shoal. Diverse coastal marine habitats, including mangrove forests, hypersaline lagoons, sandbanks, coral reef ecosystems, and other geomorphological features, make RMNR a unique area for ecological and environmental studies. Ecosystems within the reserve are threatened by anthropogenic influences including (i) the dramatic increase in the international trade through Red Sea and Suez Canal; (ii) the continuous increase in tourism activity; (iii) the progressive urbanization of coastal areas along the gulfs of Aqaba and Suez; and (iv) exploration, extraction, and transport of oil and gas. Global climate change adds further risk for RMNR ecosystems.
2.1 Sampling
Samples were collected in December 2021 and included 26 sets of surface sediment samples collected along seven transects in the coastal area of RMNR (Fig. 1b–g). Sampling was conducted during low tide to determine whether the shallow stations were subject to aerial exposure. Four transects were sampled along the southeastern coast of the mainland area (Fig. 1b), where three sets of samples were collected each from Breaka Bay (BR), Camp Site (C), Turtle Beach (T), and Ghozlani (GH). These transects extended roughly perpendicular to the coastline, starting from nearshore (0.4 m) toward open water to a maximum depth of 12.3 m. Three transects were located on the peninsula (Fig. 1c), including five sets of samples along the length of Mangrove Channel (M) and four sets from the channel to within Hidden Bay (H). At Shark Reef (SH), five sets of samples were collected, with one set from 0.5 m and the others from 4.2–9.5 m depth. In general, the bottom topography and coral distribution governed the selection of coastal sample locations. The samples were georeferenced by a portable global positioning system (Magellan GPS). Sediment samples from the Mangrove Channel, Hidden Bay, and shallow-nearshore locations were collected using short cores, whereas the deeper samples were collected by scuba diving. Four replicates were collected in each sampling site, with one to assess geochemical characteristics of sediments. The remaining three sets were preserved in 70 % ethyl alcohol and rose Bengal solution (1 g L−1) to identify foraminifers that were alive when collected (Walton, 1952). Depth was measured directly; pH, salinity, and temperature were determined in situ using a YSI 556 multimeter. The samples are stored at the Marine Geology Laboratory, Oceanography Department, Faculty of Science, Alexandria University, Egypt.
2.2 Sediment analyses
Each sample for grain size and geochemical analyses (total carbonate and organic carbon) was divided into two subsamples. Those for grain size analysis were air-dried and then disaggregated. Sand-sized sediments (>0.063 mm) dominated the samples, so only mechanical sieving was applied (Folk, 1980). About 20 g of dried sediments were sieved, using standard mesh sizes of 2, 1, 0.5, 0.25, 0.125, and 0.063 mm mounted on an electric shaker machine for 10 min. The fractions were weighed, and the weight percentage of each fraction was calculated and reported as percentages of gravel (G %), sand (S %), or mud (M %). Mean phi size (Mz Φ) and median phi (Φ50) also were calculated according to Folk and Ward (1957).
The second subsample from each site was rewashed with ultrapure distilled water several times through a sintered glass funnel, then dried at 105 °C, and pulverized to pass a 0.125 mm sieve. Subsamples were kept in clean, dry, and labeled polyethylene containers until analyses for total carbonate (TCO3 %) and total organic carbon (TOC %).
Pre-weighed sediment subsamples were treated with 10 % HCl to remove carbonate minerals. The HCl was added in excess, and samples were agitated to ensure a full reaction until no more effervescence was observed. The samples were rinsed with reverse-osmosis water several times until approaching neutral values and then dried and weighed. The percentage of total carbonate (TCO3 %) was approximated as the following: TCO3 %= [(mpre−mpost)/mpre] ×100, where m is mass of the sample pre-acid treatment and post-acid treatment, as indicated by the subscripts.
The residual solid remaining after the acid treatment was comprised of acid-insoluble minerals and organic matter. Percent organic matter was determined using an Elementar PYRO cube elemental analyzer in carbon–nitrogen mode. Standards of known elemental composition were run every 10 samples to track the calibration and drift of the instrument. We report the results as the percent organic carbon per sample mass based on pretreatment mass: %TOC , where f is the percentage of organic matter in the sample residual post-acid treatment, and masses m are either post- or pre-acid treatment, as indicated by the subscript.
2.3 Foraminiferal analyses
The rose Bengal-stained samples were washed over 0.063 mm mesh sieve, oven-dried at 65°, and then stored in clean, labeled polyethylene bags for further processing. Approximately 300 specimens were picked from each replicate and identified to species level. The results of each sample presented herein are the averages of the three replicates. Species counts in each sample were converted to percent abundance (relative abundance). The number of living (stained) specimens of each species were counted, and living percentages were calculated. Species dominance is the tendency of any species to comprise a major part of the assemblage and is usually evaluated based on the percentages of species in the assemblage and stated herein as Dom %. Absolute abundance is referred to as the number of foraminiferal tests in a given weight of dry sediments sample (Murray, 2014) and is reported as the number of foraminiferal tests (t) present in one gram of dry sediment (g) and expressed as t g−1.
Genus-level classification followed essentially that of Loeblich and Tappan (1988). The name of each species was checked and revised, if necessary, in accordance with the online database WoRMS (World Register of Marine Species; Hayward et al., 2020). All the specimens were stored in micropaleontological slides, recorded with a voucher number, and archived. Images of selected species were photographed using a Hitachi S-3500N scanning electron microscope.
2.3.1 Diversity analyses
Species diversity is commonly expressed in several ways. Species richness is the simplest method and is reported here as the average number of species present in 300 picked specimens. The α-Fisher index (Fisher et al., 1943) relates the number of species and the number of individuals in an assemblage and is commonly used in foraminiferal research (Murray, 1973, 2006, 2014). Murray considered α=5 as a boundary separating normal marine from stressed environments, where α>5 characterizes normal shelf seas and normal marine lagoons, and α<5 typically distinguishes hypersaline and hyposaline marshes, lagoons, or hypersaline shelf seas.
Heterogeneity indices are diversity measures that consider the proportions of species in a sample (Peet, 1974). The Shannon–Wiener index (H′), the Simpson index (D), and the evenness index (E) are commonly used to indicate heterogeneity (e.g., Hayek and Buzas, 2010). The Shannon–Wiener index approaches zero as one species becomes increasingly dominant. The Simpson index approaches zero as species richness increases and so is also commonly reported. The evenness index indicates whether species found in the assemblage are relatively evenly distributed or are dominated by one or a few species (Zubaida et al., 2020). Diversity indices were calculated with PAST software (Paleontological Statistics, v. 4.03). The base values that correspond to the terminologies used to describe the diversity indices (α-Fisher index, Shannon–Wiener index (H′), Simpson index (D), and evenness (E)) are presented in Table 1.
2.3.2 FoRAM Index (FI)
The Foraminifera in Reef Assessment and Monitoring (FoRAM) Index (FI) is a single-metric bioindicator that utilizes benthic foraminiferal assemblages from surface sediments (Hallock et al., 2003). The FoRAM Index is based upon three ecological groupings of foraminifers: (i) the larger foraminifers that host algal symbionts, (ii) smaller foraminifers that thrive when food resources are fairly abundant but organic matter does not exceed the availability of oxygen, and (iii) stress-tolerant foraminifers that prevail in stressed environments such as where oxygen intermittently becomes limiting or where chemical pollutants are prevalent (Hallock et al., 2003; modified by Carnahan et al., 2009).
The FoRAM Index is calculated using the following equation: FI , where Psb, Pst, and Ph represent the proportion of symbiont-bearing, stress-tolerant, and other heterotrophic taxa, respectively (Hallock et al., 2003; Carnahan et al., 2009). The base values that correspond to the terminologies used to describe the FoRAM Index are presented in Table 1.
2.3.3 Statistical analyses
For statistical purposes, the data matrix was standardized by converting the species counts of 300 specimens to percentages for each sample. While all foraminiferal species (benthic and planktic) were recorded, a species was only considered to be statistically significant if its abundance ≥2 % in at least two samples (after Asteman et al., 2021). The infrequently occurring taxa (<2 % relative abundance) are assumed to have insignificant effects on the formation of the major groups (Frezza and Carboni, 2009; Romano et al., 2008). Data from the geochemical analyses and foraminiferal indices were normalized using the following equation: [N= (value–mean)/standard deviation]. We carried out Pearson correlation analysis and then the Q-mode hierarchical cluster analysis was applied to cluster samples characterized by similar assemblages. The Ward's linkage method, based on Euclidean distance, which produces dendrograms with extremely well-defined clusters (Parker and Arnold, 1999), was applied. Canonical correspondence analysis (CCA) was used to identify factors that can be used to represent the relationships among sets of interrelated variables. The CCA was based on the data set including percentages of the statistically significant species and environmental factors known to be ecologically significant for foraminiferal distributions, including depth (m), pH, salinity (sal psu), mean grain size (Mz), median phi (Φ50), and percentages of gravel (G %), sand (S %), mud (M %), total organic carbon (TOC %), and total carbonate (TCO3 %). Pearson correlation was performed using the SPSS program (V. 22), whereas the Q-mode hierarchical cluster analysis and CCA utilized PAST software (Paleontological Statistics, v. 4.03).
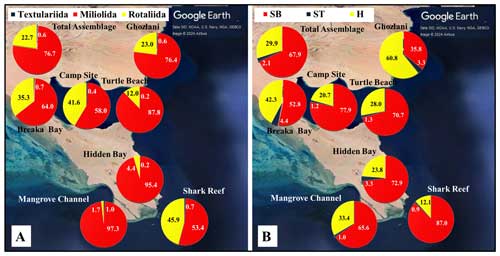
Figure 2Foraminiferal assemblages recorded in sediments of the coastal area of RMNR: (a) percentages by foraminiferal order; (b) percentages by foraminiferal functional group. SB is for symbiont bearing, ST is for stress tolerant, and H is for heterotrophic. See Fig. 1 for details regarding the © Google Earth imagery used. The base map is a high-resolution image generated in © Google Earth Pro and provided by © Airbus.
The Mangrove Channel and Hidden Bay are shallow environments with maximum depths of 0.7 and 0.6 m, respectively, while all other transects represent inshore to reef samples (Table 2). The salinity exceeded 40 everywhere and was slightly higher (>41) in the southern sites (Mangrove Channel, Hidden Bay, and Shark Reef). The pH was slightly alkaline in all sampling sites (mean 8.07), and the water temperature ranged from 18–22 °C during the sampling period in December 2021 (Table 2).
3.1 Sediments
Sand size sediments dominated (range 72 %–100 %; mean 94±8 %). Phi size ranged from 0.4 Φ (coarse sand) at Shark Reef to 3.3 Φ (very fine sand) at Turtle Beach. Shell fragments were common in coarser sediments, while fine sands were predominantly siliciclastics (Table 2). Gravel ranged from 0 %–26 % (5.8±8.4 %), with highest values in the southern sites where shell fragments were more common. Percent mud was consistently low (maximum =3.2 %; mean and SD ). Total organic carbon (TOC %) was consistently <1 % and only >0.1 % in Mangrove Channel and Hidden Bay samples. Total carbonate (TCO3 %) was bimodal (Table 2), with the southern sites (Mangrove Channel, Hidden Bay, and Shark Reef) dominated by the coarser carbonate sediments (79–96 TCO3 %), while Breaka Bay, Camp Site, Turtle Beach, and Ghozlani were dominated by fine siliciclastics (10–31 TCO3 %).
3.2 Foraminiferal assemblages
The benthic foraminiferal assemblages were dominated by porcelaneous taxa. Hyaline taxa varied from <2 % in Mangrove Channel sediments to 46 % in Shark Reef sediments (Fig. 2a). Agglutinated taxa were uncommon, and two planktic species were found. A total of 31 families were documented. Members of the order Miliolida comprised 77 % of the assemblages, represented by Peneroplidae (∼32 %), Hauerinidae (∼24 %), Soritidae (∼18 %), and Spiroloculinidae (∼3 %), with Fischerinidae, Nubeculariidae, Cribrolinoididae, Miliolidae, and Alveolinidae together making up <1 % (Fig. 3). The order Rotaliida comprised ∼23 % of the total assemblages (Fig. 2a), predominantly Amphisteginidae (∼17 %), with Calcarinidae, Rosalinidae, and Ammoniidae together comprising ∼4 % and Bolivinitidae, Buliminidae, Reussellidae, Eponididae, Discorbidae, Cibicididae, Discorbinellidae, Planorbulinidae, Cymbaloporidae, Acervulinidae, Anomalinidae, Nonionidae, Astrononionidae, Elphidiidae, and Nummulitidae combined accounting for ∼2.3 % (Fig. 3). Family Globigerinidae, representing planktic foraminifers, accounted for ∼0.1 % (Fig.3). The order Textulariida made up 0.6 % of the total assemblage, with 0.3 % for each of Textulariidae and Valvulinidae (Figs. 2, 3). The carbonate-dominated Mangrove Channel and Hidden Bay samples yielded the highest percentages of miliolids, whereas Shark Reef and the siliciclastic samples generally had higher percentages of rotaliids. An exception was the Turtle Beach samples, which were dominated by Amphisorus hemprichii, a symbiont-bearing miliolid.
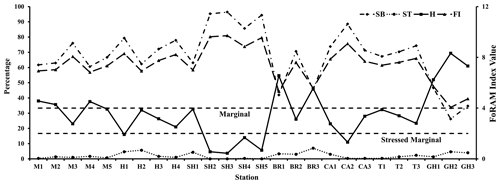
Figure 4Frequency distribution of functional group and FoRAM Index values recorded in sediments of the coastal area of RMNR (SB is for symbiont bearing, ST is for stress tolerant, H is for other heterotrophic, and FI is for FoRAM Index).
Overall, 56 genera were recorded. Symbiont-bearing genera comprised ∼68 % of the total assemblage (Table 3; Figs. 2b, 4) and were dominated by Peneroplis (28.2 %), Amphistegina (16.8 %), and Amphisorus (14.5 %), with Sorites (3 %), Coscinospira (2.6 %), Neorotalia (1.5 %), and Dendritina (1.2 %) also found. Smaller heterotrophic genera contributed about ∼30 % of the total assemblage, and the most common genus was Quinqueloculina (10.3 %), with Miliolinella, Spiroloculina, Triloculina, Pseudomassilina, Affinetrina, and Rosalina found in relative abundances of 3.1, 2.7, 2.7, 2, 1.3, and 1.1 %, respectively. The stress-tolerant group included nine genera comprising a relative abundance of ∼2 %, with Ammonia comprising more than half of this percentage (Table 3; Figs. 2b, 4). The average occurrences of the remaining 33 genera never exceeded 1 % of the total assemblage.
Table 2Locations, depth, hydrological, and sediment parameters of samples collected in the coastal area of Ras Mohamed. Station abbreviations are as follows: M is for Mangrove Channel, H is for Hidden Bay, SH is for Shark Reef, BR is for Breaka Bay, CA is for Camp Site, T is for Turtle Beach, and GH is for Ghozlani. Parameter abbreviations are as follows: mean grain size (Mz), median (Φ50), gravel percentage (G %), sand percentage (S %), mud percentage (Mud %), total organic carbon percentage (TOC %), and total carbonate percentage (TCO3 %).
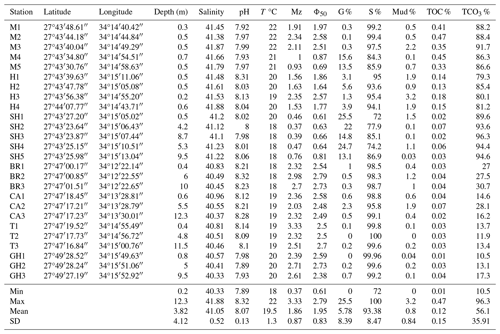
Table 3Percentages of functional groups of foraminifers used in the FoRAM Index calculation for coral reef assessments of the coastal area of Ras Mohamed Nature Reserve (after Hallock et al., 2003).
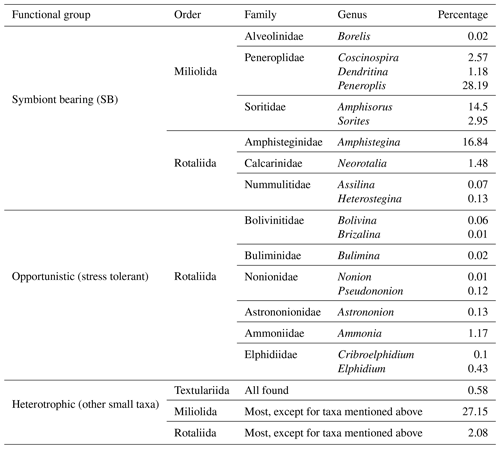
Of 95 species recorded (see Appendix A, B, and D), only 25 occurred in abundance ≥2 % in at least two samples (Table 4). Of those, 17 porcelaneous and hyaline benthic species with average occurrences exceeding 1 % of the total constituted 87 % of the total assemblages. The symbiont-bearing porcelaneous species Peneroplis pertusus, Amphisorus hemprichii, and P. planatus dominated overall at 14.7,%, 14.5,%, and 13.5 %, respectively. Commonly occurring symbiont-bearing hyaline species were Amphistegina lobifera (12.1 %) and A. lessonii (4.8 %). The commonly occurring heterotrophic porcelaneous species were Quinqueloculina seminulum, Miliolinella subrotunda, Spiroloculina antillarum, and Pseudomassilina pacificensis, in decreasing order.
The absolute abundances of tests in a gram of sediment (t g−1) varied by more than 2 orders of magnitude with between 15–55 in the siliciclastic-rich mainland samples and 2317–3180 g in carbonate-rich Mangrove Channel samples (Table 5). Hidden Bay and Shark Reef samples were intermediate, with abundances ranging from 49–249. Similarly, organic carbon (TOC %) was an order of magnitude richer in the Mangrove Channel sediments (0.33 %–0.47 %) than in the reef-related sediments (0.01 %–0.07 %), with Hidden Bay samples again intermediate (0.13 %–0.18 %).
The species richness (S) ranged from 14–57 per sample (30±10 species). The most species-rich samples were from Ghozlani (36–57), and the fewest species (14–27) were found in Shark Reef samples. The α-Fisher values ranged from 3–20.9 (8.7±3.9), with the lowest (3–4) in the Shark Reef samples (SH3, SH5) and the highest in samples from Ghozlani (GH3, 21) and Breaka Bay (BR3, 15.6). Shannon indices (H′) ranged from 1.7–3.5 (2.4±0.4), again with the lowest from Shark Reef (1.7–2.4) and the highest values recorded at Ghozlani sites (2.9–3.5) and Breaka Bay (2.6–3.0). The Simpson indices (D) ranged from 0.69–0.95 (0.86±0.06), with the lowest values in Shark Reef sediments and with the highest values at Breaka Bay and Ghozlani (>0.9) (Table 5). Evenness (E) ranged from 0.29–0.55 (0.4±0.07), with the highest values again at Ghozlani (0.5–0.55).
Table 4Mean and standard deviation (SD) for total assemblage and assemblages of each of the seven transects for the common species comprising about ≥2 % in at least two samples of the foraminiferal assemblage recorded in sediments of the coastal area of Ras Mohamed Nature Reserve (M is for Mangrove Channel, H is for Hidden Bay, SH is for Shark Reef, BR is for Breaka Bay, CA is for Camp Site, T is for Turtle Beach, and GH is for Ghozlani).
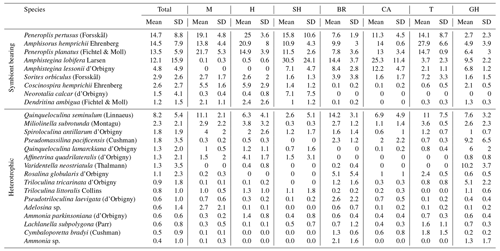
Table 5Living percentage (L %), species richness (S), α-Fisher index (α-Fisher), Shannon–Wiener index (H′), Simpson index (D), evenness (E), FoRAM Index (FI), absolute abundance (t g−1), species dominance percentage (Dom %), and dominant species (DomSp.) of the foraminiferal assemblage recorded in sediments of the coastal area of Ras Mohamed Nature Reserve (M is for Mangrove Channel, H is for Hidden Bay, SH is for Shark Reef, BR is for Breaka Bay, CA is for Camp Site, T is for Turtle Beach, and GH is for Ghozlani).
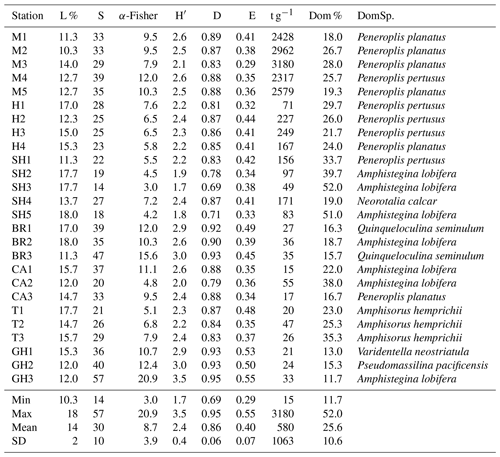
Species dominance by sample (Dom %) varied from 12 %–52 % (mean 25.6±10.6 %) (Table 5). Amphistegina lobifera dominated in 7 of 26 samples, with the highest percentages found in Shark Reef samples. Peneroplis planatus dominated six samples, and P. pertusus dominated five samples when including all the Mangrove Channel and Hidden Bay samples. Amphisorus hemprichii dominated Turtle Beach samples (T1–3) and Q. seminulum two Breaka Bay samples (BR1, 3). Neorotalia calcar, Varidentella neostriatula, and Pseudomassilina pacificensis each dominated one sample (SH4, GH1, and GH2, respectively).
A wide range of taxa were recorded living (stained) in RMNR habitats (34 species), with percentages ranging from 10 %–18 % (14±2 %) (Table 5). The most abundant species found living were P. pertusus (2.5 %), Amphisorus hemprichii (2.2 %), Amphistegina lobifera (2.2 %), P. planatus (2.1 %), and Amphistegina lessonii (∼1 %). The most abundant smaller benthic species found living was Q. seminulum (1.5 %). Of the remaining species recorded live, percentages never exceeded 0.4 % of the total and combined constituted ∼3 % of the total assemblages (Appendix B).
FoRAM Index values (FI) in all samples exceeded 4, ranging from 4.1–9.7 (mean 7.4±1.45). The percentages of the functional groups of foraminifers used in FoRAM Index calculations are presented in Appendix C. The distribution pattern of FI values reflected that of symbiont-bearing abundances (Fig. 2b), with the lowest values found at Ghozlani (mean =4.8) and highest in Shark Reef samples (mean =9.0).
3.3 Statistical analyses
Correlating diversity indices to the ecological variables can provide insight into assemblage responses. Accordingly, Pearson analysis was carried out to identify significant correlations between the determined environmental variables and calculated indices (Table 6). The strongest positive correlation was observed between foraminiferal abundance (t g−1) and TOC % (), reflecting the very high abundances in the Mangrove Channel samples. Mean Φ size (Mz), which increases as sediments become finer, positively correlated with the Shannon–Wiener index (H′) and the Simpson index (D) (r=0.55, 0.61, respectively) and negatively correlated with the FoRAM Index and species dominance ( and −0.58, respectively). These results revealed that the most diverse smaller-benthic species were found in samples with fine siliciclastic sands and low TCO3 %, while the coarser sediment samples with abundant shell fragments were mostly dominated by Peneroplis spp.
A dendrogram generated by hierarchical Q-mode cluster analysis showed two groups of samples that reflected the sediment regimes in the RMNR habitats (Fig. 5). Cluster I included the carbonate-rich samples from the southern localities grouping Mangrove Channel and Hidden Bay samples in Subcluster Ia and Shark Reef samples in Subcluster Ib. Cluster II grouped siliciclastic-rich samples from the northern localities, with samples from each site mostly grouping together. The Turtle Beach samples, which were dominated by Amphisorus hemprichii, were the most distinct of Cluster II samples.
Table 6Correlation matrix of diversity indices and environmental parameters in sediments of the coastal area of Ras Mohamed Nature Reserve. Bold text indicates a significant correlation (r>0.5). The abbreviations are as follows: mean grain size (Mz), median grain size (Φ50), gravel percentage (G %), sand percentage (S %), mud percentage (Mud %), total organic carbon percentage (TOC %), total carbonate percentage (TCO3 %), living percentage (L %), species richness (S), α-Fisher index (α-Fisher), Shannon–Wiener index (H′), Simpson index (D), evenness (E), FoRAM Index (FI), absolute abundance (t g−1), and species dominance percentage (Dom).
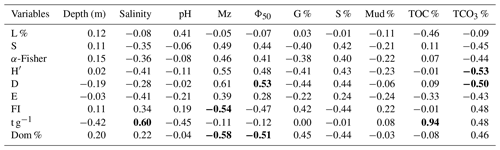
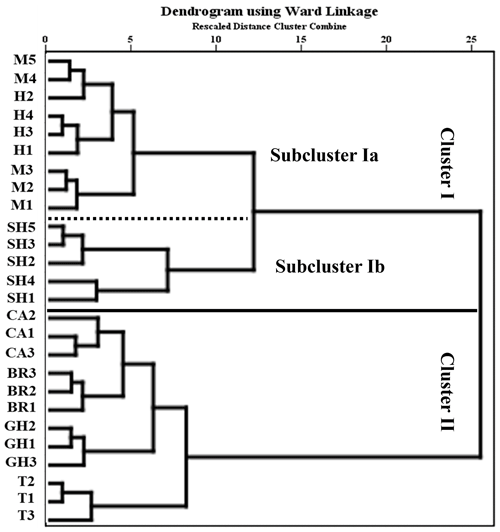
Figure 5Dendrogram from Q-mode cluster analysis using Ward's linkage method, based on foraminiferal species abundance and the environmental variables, grouping samples from the coastal area of RMNR (see Fig. 1 for locations of samples).
Canonical correspondence analysis (CCA) axes 1 and 2 yielded an ordination that explained 41 % and 30 % of the data, respectively (Appendix E). Axis 2 separates the studied stations into two main groups. The first group is on the lower side of axis 2 and includes two subgroups. Subgroup 1 is located on the right side of axis 1 and includes stations of Mangrove Channel, Hidden Bay, and SH1, whereas subgroup 2 exists on the left side of the axis and includes stations SH2, SH3, SH4, SH5, CA1, and CA2. The second group is located on the upper side of axis 2 and includes all stations of Breaka Bay, Turtle Beach, and Ghozlani, in addition to station CA3. These results greatly resemble those of Q-mode cluster analysis (Fig. 5). Larger benthic Amphistegina lessonii and A. lobifera are positively related to water depth, whereas smaller heterotrophic Quinqueloculina seminulum, Q. lamarckiana, and Miliolinella subrotunda are positively correlated with sediment texture variables (Mz, Φ50, and S %). Peneroplis planatus, Peneroplis pertusus, Amphisorus hemprichii, and the less abundant species Affinetrina quadrilateralis, Triloculina littoralis, and Ammonia parkinsoniana are more influenced by S ‰, TOC %, and Mud % (Appendix E).
Many characteristics in combination make the Red Sea a unique marine basin. As a region with an arid climate with negligible precipitation and runoff (Al-Horani et al., 2006), this semi-enclosed subtropical basin sustains rich and diverse ecosystems (Barale and Gade, 2014). With an overall negative water balance (i.e., the evaporation is greater than the precipitation) and lack of permanent rivers (Eladawy et al., 2017), the sparse fluvial transport of muddy sediments and lack of significant upwelling currents (Nasr, 2015) keep the Red Sea surface water transparency among the highest in comparison with many other tropical seas (Vine, 1985). These exceptional conditions have allowed the Red Sea to sustain the best-developed coral reefs in the western Indian Ocean region (PERSGA/GEF, 2004). Unfortunately, global climate change and socioeconomic aspects, including massive coastal urbanization (e.g., NEOM project), tourism, fishing, oil exploration, shipping and maritime activities, and organic and inorganic pollutant discharges (Nasr, 2015; Rasul and Stewart, 2015), put Red Sea ecosystems, particularly coral reefs, under increasing ecological threat. Accordingly, the commitment to monitoring these ecosystems is essential to sustaining their unique resources. Recognition of this mandate motivated this project, the goals of which are to determine the applicability of utilizing foraminiferal assemblages and, in particular, the FoRAM Index as biomonitoring tools that can be used to assess and protect these invaluable natural resources.
The two primary LBF extant orders are the porcelaneous Miliolida and the hyaline Rotaliida (Reymond et al., 2022). Porcelaneous taxa dominated the foraminiferal assemblages, with three algal symbiont-bearing porcelaneous species (Peneroplis pertusus, P. planatus, and Amphisorus hemprichii) recorded in all samples. These three species were far more abundant in the Mangrove Channel and Hidden Bay samples than the reef zone samples (Shark Reef, Breaka Bay, Camp Site, Turtle Beach, and Ghozlani). Mangrove Channel and Hidden Bay are characterized by shallow depths that never exceed 0.7 m and water transparency that allows maximum light intensity to reach those depths.
The porcelaneous LBF species in the Mangrove Channel and Hidden Bay samples attained large sizes not observed in deeper locations. In these highly stressful but survivable environmental conditions, asexual reproduction apparently can be delayed until conditions become more favorable. The algal symbionts produce sufficient photosynthate so the host can continue to add chambers and attain large sizes. But with insufficient nutrients and trace elements needed to produce amino acids and nucleic acids, reproduction can be inhibited. Hallock (1985) previously explained delayed maturation and growth to large sizes as advantageous where food resources are limited and sunlight is readily available. Hallock and Seddighi (2022) extended that interpretation to mesophotic habitats, again with emphasis on scarce food resources, with the addition of a role in the dormancy (e.g., Ross and Hallock, 2016) if light became limiting, for example, seasonally. The observation of exceptionally large porcelaneous LBFs in episodically very warm, hypersaline conditions expands the range of environmental conditions in which LBFs might experience delayed maturation and growth to large sizes where food resources are limited and sunlight is readily available.
The northern Red Sea is characterized by arid conditions, semi-diurnal tides with an ∼0.6 m tidal range, substantial temperature and salinity ranges, and dramatic diurnal fluctuations in both, especially in the summer months. Temperature plays a major role in the distribution of LBFs and is considered a controlling variable for their survival, reproduction, bleaching, and calcification (Evans et al., 2015; Hallock et al., 2006; Titelboim et al., 2019). Higher salinity is an additional stressor in Mangrove Channel and Hidden Bay due to the arid weather condition, high evaporation rate, and tidal range in these shallow coastal ecosystems.
The waters of the Ras Mohammed study area are characterized by very clear waters reflecting oligotrophic conditions and thus limited availability of food, as indicated by consistently low TOC % (<1 %). Those qualities are consistent with the dominance overall by algal symbiont-bearing species, as reflected in consistently high FoRAM Index values. In addition, the relatively high salinities (40–41 psu) favor porcelaneous taxa, especially Peneroplis and Amphisorus. Kenigsberg et al. (2022) concluded that Peneroplis spp., which host rhodophyte symbionts, can tolerate high temperatures and elevated salinities. Although the samples collected for this study were taken in winter when water temperatures were 18–22 °C, in the summer months water temperatures average 26 °C (Rasul et al., 2015) and can exceed 30 °C (Chaidez et al., 2017). Previous studies have also noted the shift in species composition from hyaline Rotaliida to porcellaneous Miliolida with increasing temperature and salinity (James et al., 1999; Dong et al., 2019).
Locally influenced features, including TCO3 %, sediment texture, water motion, and depth largely determine what assemblages of the biogeographically available taxa can occur and dominate at specific locations. In general, smaller porcelaneous taxa are found more commonly in finer sediments because of slightly higher food availability (living specimens) and concentration of dead tests by sediment sorting. At the other end of the texture and energy spectrum are coarser sediments that are rich in bioclastic material, for example, at Shark Reef where Neorotalia calcar was abundant.
The range of sample depths was limited (0.2–12.3 m), and more than half of the samples were collected from <1 m depths. Peneroplis dominated in such samples from the Mangrove Channel and Hidden Bay, while Amphistegina dominated mostly in samples from depths between 4.2 and 9.5 m. The three deepest samples (10, 11.5, and 12.3 m) were each characterized by a different dominant species. In the Breaka Bay sample, BR3 (10 m depth), Q. seminulum was the most abundant species (also in BR1), rather than a symbiont-bearing species, though dominance was relatively low (16 %). This species is common in marginal environments throughout the Red Sea coastal regions (e.g., El-Kahawy and Mabrouk, 2023; El-Menhawey et al., 2020; Youssef, 2015). At the CA3 site (12.3 m depth), Peneroplis planatus exhibited the highest relative abundance, though at only 17 %. The Turtle Beach samples were all dominated by Amphisorus hemprichii. Gabr et al. (2020a, b) reported that macroalgae and seagrass flourish in the coastal area of RMNR, providing a habitat for epiphytic taxa.
We recorded 95 species in 26 samples from depths ≤12.3 m, representing several distinct habitats in RMNR. The four mainland sites, which were all dominated by fine siliciclastic sands, produced relatively sparse (low t g−1) but diverse foraminiferal assemblages. Those sample sets produced a distinct cluster overall, though samples from each location grouped together. Amphistegina lobifera was commonly found at three of the sites, while Amphisorus dominated Turtle Beach samples, as noted above.
The Shark Reef samples were characterized by much higher TCO3 %, higher proportions of gravel (i.e., shell fragments), and ∼4 times higher abundances of foraminiferal specimens than the mainland samples. Amphistegina lobifera dominated three of the five Shark Reef samples, which as a group exhibited the lowest diversities and the highest FoRAM indices of all sites.
Table 7Data comparing indices from this study with previous foraminiferal studies in the Red Sea and Gulf of Aden. Absolute abundance (t g−1), species richness (S), α-Fisher index (α-Fisher), Shannon–Wiener index (H′), and FoRAM Index (FI) are indicated.
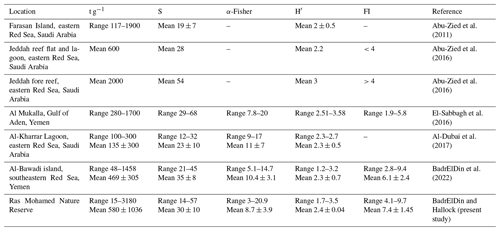
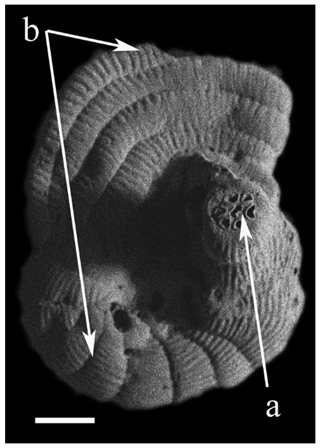
Figure 6(a) Coscinospira hemprichii Ehrenberg and (b) Peneroplis planatus (Fichtel & Moll) hybrid specimen.
The Mangrove Channel and Hidden Bay samples were very similar in all respects, except two. They were dominated by Peneroplis spp., their diversity indices were similar, and sediment texture was >84 % sand, with mostly carbonates (TCO3 % >79 %). The major difference between sites was in absolute abundance, with Mangrove Channel samples averaging nearly 2700 tests per gram, while Hidden Bay samples averaged 179 tests per gram. The other notable difference was in organic carbon, where TOC % in Mangrove Channel samples averaged 0.4 % and Hidden Bay samples averaged 0.15 %. Given that the percentages of specimens recorded as live when collected were similar, the much higher absolute abundances of live specimens in Mangrove Channel was likely a factor in its higher TOC %, combined with exceptionally large sizes of Peneroplis and Amphisorus.
The results of diversity indices from RMNR are mostly consistent with the findings from previous studies (Table 7) from Gulf of Aden and southern and east central Red Sea (Abu-Zied et al., 2011, 2016; El-Sabbagh et al., 2016; Al-Dubai et al., 2017; BadrElDin et al., 2022). The most notable observations are the contrasting results between the FoRAM Index and the diversity indices, especially in samples from stations SH2, SH3, SH5, and CA2 (Tables 5–6). These samples showed exceptionally high FoRAM Index values (range 9.1–9.7), indicating that environmental conditions support extreme dominance by calcifying organisms hosting algal endosymbionts. On the contrary, the α-Fisher index values (<4) indicate stressed conditions, according to Murray (1973).
We also observed anomalous specimens of peneroplids and soritids (∼2 %) in samples from Hidden Bay (Appendix D), likely associated with natural fluctuations in salinity and temperature. Several previous studies have attributed the presence of test anomalies to anthropogenic pollution in coastal areas of the Red Sea (e.g., El-Kahawy et al., 2018; El-Kahawy and Mabrouk, 2023). However, abnormalities in porcelaneous tests are relatively common in areas of elevated salinity or strong salinity fluctuations (e.g., Hofker, 1971; Reiss and Hottinger, 1984; Amao et al., 2018). The test anomalies observed in samples from RMNR were all found in porcelaneous specimens from Hidden Bay. A Coscinospira hemprichii–Peneroplis planatus hybrid specimen (Fig. 6) was found at Hidden Bay (<0.5 m), where salinity exceeded 41 (Table 2). Hidden Bay represents an exceptionally stressed coastal environment, particularly in a warming world. With respect to high temperature and salinity and very limited availability of organic matter, Peneroplis and Amphisorus are clearly “stress-tolerant” foraminifers but are tolerant of very different stressors than smaller rotaliids such as Ammonia.
Coastal waters of the study area in the Ras Mohamed Nature Reserve are characterized by very clear waters reflecting ultra-oligotrophic conditions and limited availability of food as indicated by low TOC % (<1 %). Those qualities are consistent with the dominance overall by algal symbiont-bearing species, as reflected in consistently high FoRAM Index values, even at sites <1 m in depth. The relatively high salinities (40–41) favor porcelaneous taxa, especially symbiont-bearing Peneroplis and Amphisorus. The low α-Fisher indices and commonly observed “abnormal” peneroplid and soritid specimens indicate that these metrics must be used with caution when assessing tropical–subtropical shallow-water assemblages in hypersaline environments. Benthic species that host algal symbionts represent a tiny fraction of total numbers of benthic species, yet photosynthesis by the algal symbionts allows the dominance of those few in clear, shallow, and reef-associated waters where particulate food is limited. In addition, morphological variability is extremely common in peneroplids and soritids in hypersaline environments. Local differences in foraminiferal assemblages reflect depth, exposure to water motion, sediment texture, and proportion of carbonate in the sediments.
Table A1Foraminiferal taxa recorded in sediments from the coastal area of RMNR (M is for Mangrove Channel, H is for Hidden Bay, and SH is for Shark Reef).
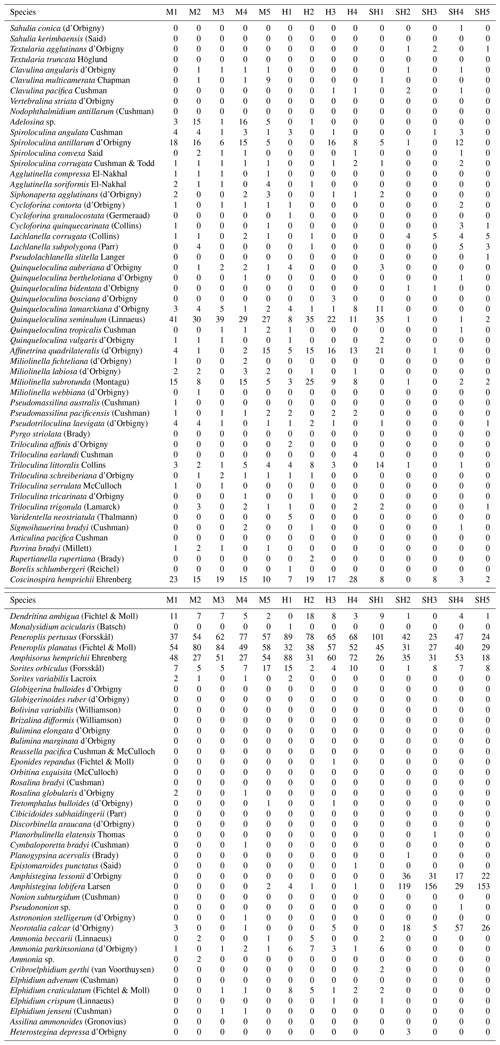
Table B1Number of the living foraminiferal taxa recorded in sediments from the coastal area of RMNR (M is for Mangrove Channel, H is for Hidden Bay, and SH is for Shark Reef).
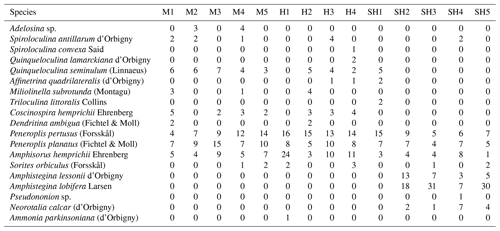
Table C1Symbiont-bearing, stress-tolerant, and other-heterotrophic taxa percentages and the FoRAM Index (FI) of the foraminiferal assemblage recorded in sediments from the coastal area o Ras Mohamed Nature Reserve. M is for Mangrove Channel, H is for Hidden Bay, SH is for Shark Reef, BR is for Breaka Bay, CA is for Camp Site, T is for Turtle Beach, and GH is for Ghozlani.
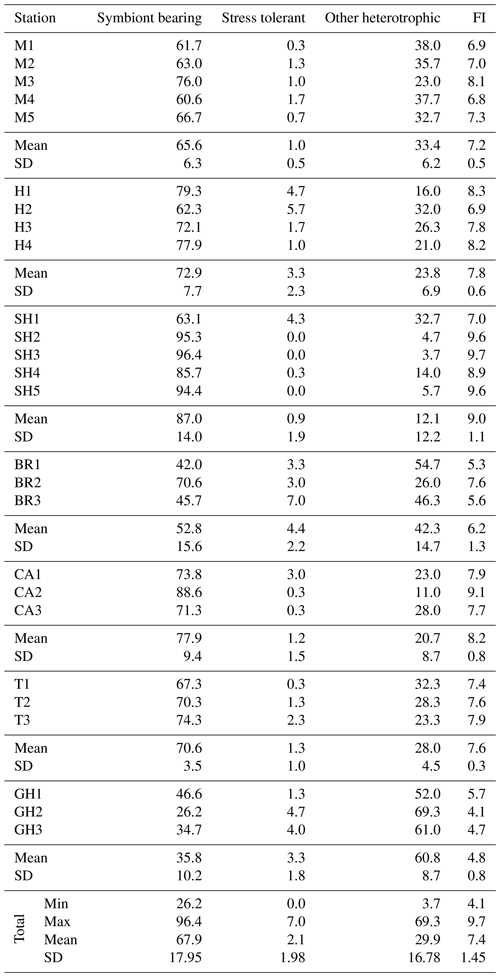
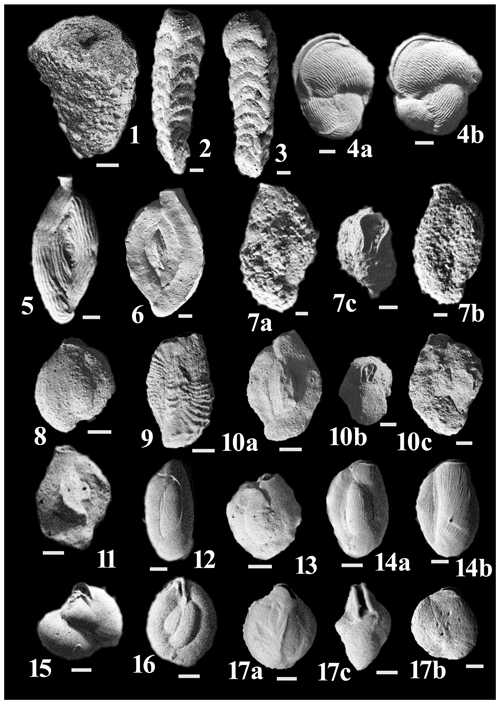
Figure C1(1) Sahulia conica (d'Orbigny). (2) Clavulina angularis d'Orbigny Höglund. (3) Clavulina pacifica Cushman. (4a–b) Vertebralina striata d'Orbigny. (5) Spiroloculina antillarum d'Orbigny. (6) Spiroloculina convexa Said. (7a–c) Agglutinella compressa El-Nakhal. (8) Siphonaperta agglutinans (d'Orbigny). (9) Lachlanella corrugata (Collins). (10a–c) Lachlanella subpolygona (Parr). (11) Quinqueloculina bidentata d'Orbigny. (12) Quinqueloculina tropicalis Cushman. (13) Quinqueloculina vulgaris d'Orbigny. (14a–b) Affinetrina quadrilateralis (d'Orbigny). (15) Miliolinella labiosa (d'Orbigny). (16) Miliolinella subrotunda (Montagu). (17a–c) Pseudomassilina australis (Cushman).
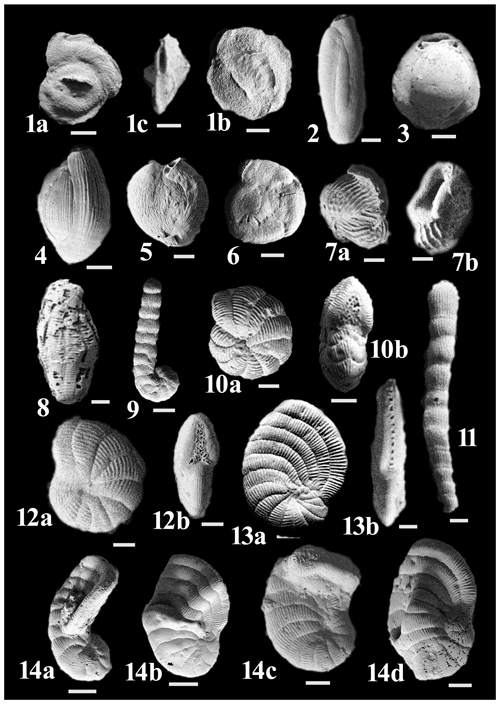
Figure C2(1a–c) Pseudomassilina pacificensis (Cushman). (2) Pseudotriloculina laevigata (d'Orbigny). (3) Pyrgo striolata (Brady). (4) Triloculina earlandi Cushman. (5) Varidentella neostriatula (Thalmann). (6) Sigmoihauerina bradyi (Cushman). (7a–b) Articulina pacifica Cushman. (8) Borelis schlumbergeri (Reichel). (9) Coscinospira hemprichii Ehrenberg. (10a–b) Dendritina ambigua (Fichtel & Moll). (11) Monalysidium acicularis (Batsch). (12a–b) Peneroplis pertusus (Forsskål). (13a–b) Peneroplis planatus (Fichtel & Moll). (14a–d) Anomalous specimens of Peneroplis planatus (Fichtel & Moll).
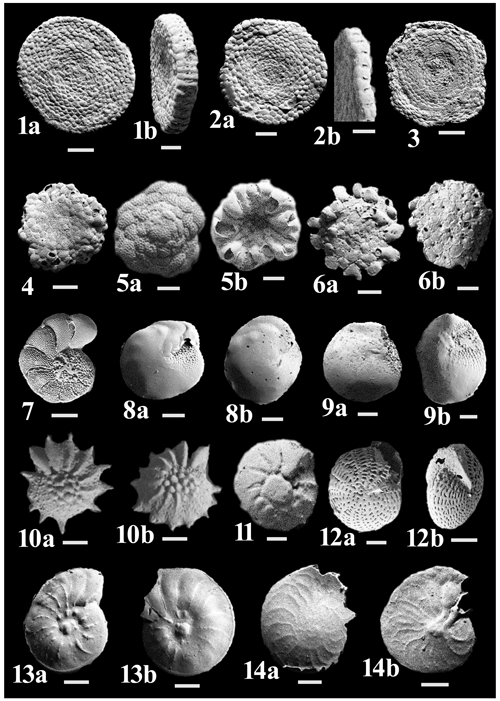
Figure C3(1a–b) Amphisorus hemprichii Ehrenberg. (2a–b) Sorites orbiculus (Forsskål). (3) Sorites variabilis Lacroix. (4) Planorbulinella elatensis Thomas. (5a–b) Cymbaloporetta bradyi (Cushman). (6a–b) Planogypsina acervalis (Brady). (7) Epistomaroides punctatus (Said). (8a–b) Amphistegina lessonii d'Orbigny. (9a–b) Amphistegina lobifera Larsen. (10) Neorotalia calcar (d'Orbigny). (11a–b) Ammonia parkinsoniana (d'Orbigny). (12a–b) Elphidium craticulatum (Fichtel & Moll). (13a–b) Assilina ammonoides (Gronovius). (14a–b) Heterostegina depressa d'Orbigny.
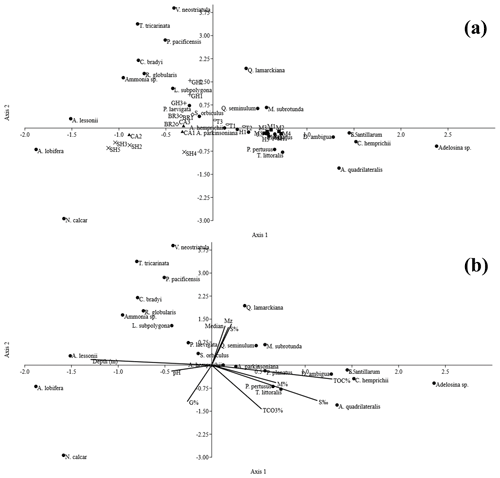
Figure C4Canonical correspondence analysis for the average values for (a) foraminiferal species abundance and (b) the environmental variables for sediments from the coastal area of RMNR, based upon PC1 (variance 29 %) and PC2 (variance 20 %). Depth, pH, salinity (sal psu), mean grain size (Mz), median (Φ50), gravel percentage (G %), sand percentage (S %), mud percentage (M %), total organic carbon percentage (TOC %), and total carbonate percentage (TCO3 %) are shown.
All data sets discussed in the present work are available in Tables 1–6 and Appendix A–C.
The samples are stored at the Marine Geology Laboratory, Oceanography Department, Faculty of Science, Alexandria University, Egypt.
Samples were collected and analyzed by AMB. Most of the statistical analyses were carried out by AMB in consultation and discussions with PH. An initial draft was written by AMB, with subsequent revisions and interpretations by PH. The submitted draft was collaboratively written by AMB and PH.
The contact author has declared that none of the authors has any competing interests.
Publisher's note: Copernicus Publications remains neutral with regard to jurisdictional claims made in the text, published maps, institutional affiliations, or any other geographical representation in this paper. While Copernicus Publications makes every effort to include appropriate place names, the final responsibility lies with the authors.
This article is part of the special issue “Advances and challenges in modern and benthic foraminifera research: a special issue dedicated to Professor John Murray”. It is not associated with a conference.
This work is dedicated to the memory of John Murray, whose pioneering work in modern benthic foraminiferal assemblages inspired generations of researchers. A fellowship from the Ministry of Higher Education and Scientific Research, Arab Republic of Egypt, and the invitation from the College of Marine Science, University of South Florida, provided the authors the opportunity to collaborate on this paper. We are thankful to Brad Rosenheim and Devon Firesinger, CMS, who assisted with geochemical analyses, and Ian Williams, CMS, who assisted with the scanning electron microscopy.
This paper was edited by Irina Polovodova Asteman and reviewed by two anonymous referees.
Abu-Zied, R. H., Bantan, R. A., Basaham, A. S., El-Mamoney, M. H., and Al-Washmi, H. A.: Composition, distribution, and taphonomy of nearshore benthic foraminifera of the Farasan Islands, Southern Red Sea, Saudi Arabia, J. Foramin. Res., 41, 349–362, 2011.
Abu-Zied, R. H., Al-Dubai, T. A. M., and Bantan, R. A.: Environmental conditions of shallow waters alongside the southern Corniche of Jeddah based on benthic foraminifera, physico-chemical parameters and heavy metals, J. Foramin. Res., 46, 149–170, https://doi.org/10.2113/gsjfr.46.2.149, 2016.
Al-Dubai, T. A. M., Abu-Zied, R. H., and Basaham, A. S.: Diversity and distribution of benthic foraminifera in the Al-Kharrar Lagoon, eastern Red Sea coast, Saudi Arabia, Micropaleontology, 63, 275–303, https://doi.org/10.47894/mpal.63.5.02, 2017.
Al-Horani, F. A., Al-Rousan, S. A., Al-Zibdeh, M., and Khalaf, M. A.: The status of coral reefs on the Jordanian coast of the Gulf of Aqaba, Red Sea, Zool. Middle East, 38, 99–110, https://doi.org/10.1080/09397140.2006.10638171, 2006.
Amao, A. O., Kaminski, M. A., and Babalola, L.: Benthic foraminifera in hypersaline Salwa Bay (Saudi Arabia): an insight into future climate change in the Gulf region?, J. Foramin. Res., 48, 29–40, https://doi.org/10.2113/gsjfr.48.1.29, 2018.
Asteman, I. P., Nieuwenhove, N., Andersen, T. J., Linders, T., and Nordberg, K.: Recent environmental change in the Kosterhavet National Park marine protected area as reflected by hydrography and sediment proxy data, Mar. Environ. Res., 166, 105265, https://doi.org/10.1016/j.marenvres.2021.105265, 2021.
BadrElDin, A. M. and Hallock, P.: Foraminifers associated with macroalgae on a wave-cut platform off Abu Qir coastal area, Egypt, Egypt. J. Aquat. Res., 48, 389–395, https://doi.org/10.1016/j.ejar.2022.08.003, 2022.
BadrElDin, A. M. and Hallock, P.: Foraminiferal assemblages from the Abu Qir coastal area (Alexandria, Egypt): Wave-cut platform versus shallow-bay sediments, Mar. Micropaleontol., 182, 102250, https://doi.org/10.1016/j.marmicro.2023.102250, 2023.
BadrElDin, A. M., Makbool, M. M. A., ElSabrouti, M. A., and Hallock, P.: Distribution and diversity of benthic foraminifera in the coastal area of Al-Bawadi Island, Southern Red Sea, J. Foramin. Res., 52, 264–277, https://doi.org/10.2113/gsjfr.52.4.264, 2022.
Barale, V. and Gade, M. (Eds.): Remote Sensing of the African Seas, Springer Netherlands, Dordrecht, XXIX, 428 pp., https://doi.org/10.1007/978-94-017-8008-7, 2014.
Bergamin, L., Di Bella, L., Ferraro, L., Frezza, V., Pierfranceschi, G., and Romano, E.: Benthic foraminifera in a coastal marine area of the eastern Ligurian Sea (Italy): Response to environmental stress, Ecol. Indic., 96, 16–31, https://doi.org/10.1016/j.ecolind.2018.08.050, 2019.
Bosworth, W.: Geological evolution of the Red Sea: Historical background, review, and synthesis, in: The Red Sea, edited by: Rasul, N. and Stewart, I., Springer, Berlin, Heidelberg, Germany, 45–78, https://doi.org/10.1007/978-3-662-45201-1_3, 2015.
Braithwaite, C. J. R.: Patterns of accretion of reefs in the Sudanese Red Sea, Mar. Geol., 45, 297–325, https://doi.org/10.1016/0025-3227(82)90116-5, 1982.
Bryant, D., Burke, L., McManus, J., and Spalding, M.: Reefs at Risk – A map-based indicator of threats to the world's coral reefs, World Resources Institute (WRI), International Center for Living Aquatic Resources Management (ICLARM), World Conservation Monitoring Centre (WCMC), United Nations Environment Programme (UNEP), Washington DC, USA, 56 pp., https://doi.org/10.1007/978-90-481-2639-2_274, 1998.
Carnahan, E. A., Hoare, A. M., Hallock, P. M., Lidz, B. H., and Reich, C. D.: Foraminiferal assemblages in Biscayne Bay, Florida, USA: Responses to urban and agricultural influence in a subtropical estuary, Mar. Pollut. Bull., 59, 221–233, https://doi.org/10.1016/j.marpolbul.2009.08.008, 2009.
Chaidez, V., Dreano, D., Agusti, S., Duarte, C. M., and Hoteit, I.: Decadal trends in Red Sea maximum surface temperature, Sci. Rep., 7, 8144, https://doi.org/10.1038/s41598-017-08146-z, 2017.
Dong, S., Lei, Y., Li, T., and Jian, Z.: Responses of benthic foraminifera to changes of temperature and salinity: Results from a laboratory culture experiment, Sci. China Earth Sci., 62, 459–472, https://doi.org/10.1007/s11430-017-9269-3, 2019.
Donnici, S., Serandrei-Barbero, R., Bonardi, M., and Sperle, M.: Benthic foraminifera as proxies of pollution: The case of Guanabara Bay (Brazil), Mar. Pollut. Bull., 64, 2015–2028, https://doi.org/10.1016/j.marpolbul.2012.06.024, 2012.
Eddy, T. D., Lam, V. W. Y., Reygondeau, G., Cisneros-Montemayor, A. M., Greer, K., Palomares, M. L. D., Bruno, J. F., Ota, Y., and Cheung, W. W. L. Global decline in capacity of coral reefs to provide ecosystem services, One Earth, 4, 1278–1285, https://10.1016/j.oneear.2021.08.016, 2021.
Eladawy, A., Nadaoka, K., Negm, A., Abdel-Fattah, S., Hanafy, M., and Shaltout, M.: Characterization of the northern Red Sea's oceanic features with remote sensing data and outputs from a global circulation model, Oceanologia, 59, 213–237, https://doi.org/10.1016/j.oceano.2017.01.002, 2017.
El-Kahawy, R., El-Shafeiy, M., Helal, S. A., Aboul-Ela, N., and El-Wahab, M. A.: Morphological deformities of benthic foraminifera in response to nearshore pollution of the Red Sea, Egypt, Environ. Monit. Assess., 190, 312, https://doi.org/10.1007/s10661-018-6695-2, 2018.
El-Kahawy, R. M. and Mabrouk, M. S.: Benthic foraminifera as bioindicators for the heavy metals in the severely polluted Hurghada Bay, Red Sea coast, Egypt, Environ. Sci. Pollut. Res., 30, 70437–70457, https://doi.org/10.1007/s11356-023-27242-4, 2023.
El-Menhawey, W., Kholeif, S. E. A., Elshanawany, R., and Ibrahim, M. I. A.: Benthic foraminifera as bio-indicator of Mangrove ecosystem quality: A case study from Abu Ghoson area, Red Sea Coast, Egypt, Reg. Stud. Mar. Sci., 40, 101506, https://doi.org/10.1016/j.rsma.2020.101506, 2020.
El-Sabbagh, A. M., Ibrahim, M. I., Mostafa, A. R., Al-Habshi, N. O., and Abdel Kireem, M. R.: Benthic foraminiferal proxies for pollution monitoring in Al-Mukalla coastal area, Hadramout governate, Republic of Yemen, J. Foramin. Res., 46, 369–392, https://doi.org/10.2113/gsjfr.46.4.369, 2016.
Evans, D., Erez, J., Oron, S., and Müller, W.: Mg/Ca-temperature and seawater-test chemistry relationships in the shallow-dwelling large benthic foraminifera Operculinaammonoides, Geochim. Cosmochim. Ac., 148, 325–342, https://doi.org/10.1016/j.gca.2014.09.039, 2015.
Fisher, R. A., Corbet, A. S., and Williams, C. B.: The relation between the number of species and the number of individuals in a random sample of an animal population, J. Anim. Ecol., 12, 42–58, 1943.
Folk, R. L.: Petrology of Sedimentary Rocks, Hemphill Publishing Company, Austin, 184 pp., http://hdl.handle.net/2152/22930, 1980.
Folk, R. L. and Ward, W. C.: Brazos River Bar: A study in the significance of grain size parameters, J. Sediment. Res., 27, 3–26, https://doi.org/10.1306/74D70646-2B21-11D7-8648000102C1865D, 1957.
Frezza, V. and Carboni, M. G.: Distribution of recent foraminiferal assemblages near the Ombrone River mouth (Northern Tyrrhenian Sea, Italy), Rev. Micropaléontologie, 52, 43–66, https://doi.org/10.1016/J.REVMIC.2007.08.007, 2009.
Gabr, M. K., Ziena, A. F., and Ahmed, M.: Abundance and diversity of amphipod species associated with macro-algae at Ras-Mohamed, Aqaba Gulf, Red Sea, Egypt, Egypt. J. Aquat. Biol. Fish., 24, 1–15, https://doi.org/10.21608/ejabf.2020.87108, 2020a.
Gabr, M. K., Zeina, A. F., and Hellal, A. M.: Influence of algal architecture and shore exposure on population dynamics of marine amphipods at Ras Mohamed Protectorate, Egypt, Egypt. J. Aquat. Biol. Fish., 24, 59–72, https://doi.org/10.21608/ejabf.2020.109900, 2020b.
Gitaputri, K., Kasmara, H., Erawan, T. S., and M., S.: Benthic foraminifera as bioindicator of coral reef environmental condition based on FoRAM index in Natuna Islands, province of Riau Islands, J. Ilmu dan Teknol. Kelaut. Trop., 5, 26–35, 2013.
Hallock, P.: Why are larger foraminifera large?, Paleobiology, 11, 195–208, 1985.
Hallock, P.: Symbiont-bearing Foraminifera, in: Modern Foraminifera, edited by: Sen Gupta, B. K., Springer Netherlands, Dordrecht, 123–139, https://doi.org/10.1007/0-306-48104-9_8, 1999.
Hallock, P. and Seddighi, M.: Why did some larger benthic foraminifera become so large and flat?, Sedimentology, 69, 74–87, https://doi.org/10.1111/sed.12837, 2022.
Hallock, P., Williams, D. E., Fisher, E. M., and Ayoub, L. M.: Bleaching in foraminifera with algal symbionts: implications for reef monitoring and risk assessment, Anuário do Inst. Geociências, 29, 172–173, https://doi.org/10.11137/2006_1_172-173, 2006.
Hallock, P. M., Lidz, B. H., Cockey-Burkhard, E. M., and Donnelly, K. B.: Foraminifera as bioindicators in coral reef assessment and monitoring: The FoRAM Index, Environ. Monit. Assess., 811, 221–238, https://doi.org/10.1023/A:1021337310386, 2003.
Hayek, L. C. and Buzas, M. A.: Surveying Natural Populations, 2nd ed., Columbia University Press, 590 pp., https://doi.org/10.7312/haye14620, 2010.
Hayward, B. W., Le Coze, F., Vandepitte, L., and Vanhoorne, B.: Foraminifera in the World Register of Marine Species (Worms) Taxonomic Database, J. Foramin. Res., 50, 291–300, https://doi.org/10.2113/gsjfr.50.3.291, 2020.
Hofker, J.: The foraminifera of Piscadera Bay, Curaçao, Stud. Fauna Curacao other Caribb. Islands, 35, 1–62, 1971.
Hulver, A. M., Steckbauer, A., Ellis, J. I., Aylagas, E., Roth, F., Kharbatia, N., Thomson, T., Carvalho, S., Jones, B. H., and Berumen, M. L.:Interaction effects of crude oil and nutrient exposure on settlement of coral reef benthos, Mar. Pollut. Bull., 185, 114352, https://doi.org/10.1016/j.marpolbul.2022.114352, 2022.
James, N. P., Collins, L. B., Bone, Y., and Hallock, P.: Rottnest Shelf to Ningaloo Reef: cool-water to warm-water carbonate transition on the continental shelf of Western Australia, J. Sediment. Res., 69, 1297–1321, 1999.
Jobbins, G.: Tourism and coral-reef-based conservation: can they coexist?, in: Coral Reef Conservation, edited by: Cote, I. M. and Reynolds, J. D., Conservation Biology Series, Cambridge University Press, 13, 237–263, https://doi.org/10.1017/CBO9780511804472, 2006.
Kelmo, F. and Hallock, P.: Responses of foraminiferal assemblages to ENSO climate patterns on bank reefs of northern Bahia, Brazil: A 17-year record, Ecol. Indic., 30, 148–157, https://doi.org/10.1016/j.ecolind.2013.02.009, 2013.
Kenigsberg, C., Titelboim, D., Ashckenazi-Polivoda, S., Herut, B., Kucera, M., Zukerman, Y., Hyams-Kaphzan, O., Almogi-Labin, A., and Abramovich, S.: The combined effects of rising temperature and salinity may halt the future proliferation of symbiont-bearing foraminifera as ecosystem engineers, Sci. Total Environ., 806, 150581, https://doi.org/10.1016/j.scitotenv.2021.150581, 2022.
Koukousioura, O., Dimiza, M. D., Triantaphyllou, M. V., and Hallock, P. M.: Living benthic foraminifera as an environmental proxy in coastal ecosystems: A case study from the Aegean Sea (Greece, NE Mediterranean), J. Mar. Syst., 88, 489–501, https://doi.org/10.1016/j.jmarsys.2011.06.004, 2011.
Loeblich, A. R. and Tappan, H.: Foraminiferal Genera and Their Classification, Springer US, Boston, MA, https://doi.org/10.1007/978-1-4899-5760-3, 1988.
Murray, J. W.: Distribution and ecology of living benthic foraminiferids, Crane Russak & Co., New York, 274 pp., https://doi.org/10.4319/lo.1973.18.6.1011a, 1973.
Murray, J. W.: Ecology and applications of benthic foraminifera, Cambridge University Press, Cambridge, 426 pp., https://doi.org/10.1017/CBO9780511535529, 2006.
Murray, J. W.: Ecology and paleoecology of benthic foraminifera, Routledge, New York, 425 pp., https://doi.org/10.4324/9781315846101, 2014.
Narayan, Y. R. and Pandolfi, J. M.: Benthic foraminiferal assemblages from Moreton Bay, South-East Queensland, Australia: Applications in monitoring water and substrate quality in subtropical estuarine environments, Mar. Pollut. Bull., 60, 2062–2078, https://doi.org/10.1016/j.marpolbul.2010.07.012, 2010.
Nasr, D.: Coral Reefs of the Red Sea with Special Reference to the Sudanese Coastal Area, in: The Red Sea, 453–469, https://doi.org/10.1007/978-3-662-45201-1_26, 2015.
Nasr, S., Abdel-Kader, A. F., El-Gamilyt, H. I., and El-Raey, M.: Coastal zone geomorphology of Ras-Mohammed area, Red Sea, Egypt, J. Coast. Res., 13, 134–140, 1997.
National Voluntary Review: Sustainable development goals, Ministry of International Cooperation, Arab Republic of Egypt, 105 pp., https://sustainabledevelopment.un.org/content/documents/10443egypt.pdf (last accessed: 5 July 2024), 2016.
Naumann, M. S., Bednarz, V. N., Ferse, S. C. A., Niggl, W., and Wild, C.: Monitoring of coastal coral reefs near Dahab (Gulf of Aqaba, Red Sea) indicates local eutrophication as potential cause for change in benthic communities, Environ. Monit. Assess., 187, 44, https://doi.org/10.1007/s10661-014-4257-9, 2015.
O'Brien, P. A. J., PolovodovaAsteman, I., and Bouchet, V. M. P.: Benthic Foraminiferal Indices and Environmental Quality Assessment of Transitional Waters: A Review of Current Challenges and Future Research Perspectives, Water, 13, 1898, https://doi.org/10.3390/w13141898, 2021.
Oliver, L. M., Fisher, W. S., Dittmar, J., Hallock, P., Campbell, J., Quarles, R. L., Harris, P., and LoBue, C.: Contrasting responses of coral reef fauna and foraminiferal assemblages to human influence in La Parguera, Puerto Rico, Mar. Environ. Res., 99, 95–105, https://doi.org/10.1016/j.marenvres.2014.04.005, 2014.
Pandolfi, J. M., Bradbury, R. H., Sala, E., Hughes, T. P., Bjorndal, K. A., Cooke, R. G., McArdle, D., McClenachan, L., Newman, M. J. H., Paredes, G., Warner, R. R., and Jackson, J. B. C.: Global trajectories of the long-term decline of coral reef ecosystems, Science, 301, 955–958, https://10.1126/science.1085706, 2003.
Parker, J. H., Gischler, E., and Eisenhauer, A.: Biodiversity of foraminifera from Late Pleistocene to Holocene coral reefs, South Sinai, Egypt, Mar. Micropaleontol., 86–87, 59–75, https://doi.org/10.1016/j.marmicro.2012.02.002, 2012.
Parker, W. C. and Arnold, A. J.: Quantitative methods of data analysis in foraminiferal ecology, in: Modern foraminifera, edited by: Sen Gupta, B. K., Springer, Dordrecht, 71–89, 1999.
Peet, R. K.: The Measurement of Species Diversity, Annu. Rev. Ecol. Syst., 5, 285–307, https://doi.org/10.1146/annurev.es.05.110174.001441, 1974.
PERSGA/GEF: Standard survey methods for key habitats and key species in the Red Sea and Gulf of Aden, PERSGA, Jeddah, 310 pp., https://www.informea.org/sites/default/files/imported-documents/TS10_SSM_for_Key_Habitat.pdf (last accessed: 5 July 2024), 2004.
PERSGA: The Status of Coral Reefs in the Red Sea and Gulf of Aden: 2009. PERSGA Technical Series No. 16, https://www.informea.org/sites/default/files/imported-documents/Coral_Reef_Status_Report_2009.pdf (last accessed: 5 July 2024), 2010.
Pisapia, C., El Kateb, A., Hallock, P., and Spezzaferri, S.: Assessing coral reef health in the North Ari Atoll (Maldives) using the FoRAM Index, Mar. Micropaleontol., 133, 50–57, https://doi.org/10.1016/j.marmicro.2017.06.001, 2017.
Prazeres, M., Martínez-Colón, M., and Hallock, P.: Foraminifera as bioindicators of water quality: The FoRAM Index revisited, Environ. Pollut., 257, 113612, https://doi.org/10.1016/j.envpol.2019.113612, 2020.
Rasul, N. M. A. and Stewart, I. C. F. (Eds.): The Red Sea, Springer Berlin Heidelberg, Berlin, Heidelberg, https://doi.org/10.1007/978-3-662-45201-1, 2015.
Rasul, N. M. A., Stewart, I. C. F., and Nawab, Z. A.: Introduction to the Red Sea: Its Origin, Structure, and Environment, in: The Red Sea The Formation, Morphology, Oceanography and Environment of a Young Ocean Basin, edited by: Rasul, N. M. A. and Stewart, I. C. F., Springer-Verlag GmbH Berlin Heidelberg, 1–28, https://doi.org/10.1007/978-3-662-45201-1_1, 2015.
Reiss, Z. and Hottinger, L.: The Gulf of Aqaba-Ecological Micropaleontology studies, Springer-Verlag, Berlin, Heidelberg, 354 pp., https://doi.org/10.1007/978-3-642-69787-6, 1984.
Reymond, C. E., Hallock, P., and Westphal, H.: Preface for “Tropical Large Benthic Foraminifera: Adaption, Extinction, and Radiation”, J. Earth Sci., 33, 1339–1347, https://doi.org/10.1007/s12583-021-1590-0, 2022.
Romano, E., Bergamin, L., Finoia, M. G., Carboni, M. G., Ausili, A., and Gabellini, M.: Industrial pollution at Bagnoli (Naples, Italy): Benthic foraminifera as a tool in integrated programs of environmental characterisation, Mar. Pollut. Bull., 56, 439–457, https://doi.org/10.1016/j.marpolbul.2007.11.003, 2008.
Ross, B. J. and Hallock, P.: Dormancy in the foraminifera: A review, J. Foramin. Res., 46, 358–368, https://doi.org/10.2113/gsjfr.46.4.358, 2016.
Sabbatini, A., Morigi, C., Nardelli, M. P., and Negri, A.: Foraminifera, in: The Mediterranean Sea, Springer Netherlands, Dordrecht, 237–256, https://doi.org/10.1007/978-94-007-6704-1_13, 2014.
Schönfeld, J., Alve, E., Geslin, E., Jorissen, F., Korsun, S., and Spezzaferri, S.: The FOBIMO (FOraminiferalBIo-MOnitoring) initiative-Towards a standardised protocol for soft-bottom benthic foraminiferal monitoring studies, Mar. Micropaleontol., 94–95, 1–13, https://doi.org/10.1016/j.marmicro.2012.06.001, 2012.
Schwartz, M. L. (Ed.): Encyclopedia of Coastal Science, Springer Netherlands, Dordrecht, https://doi.org/10.1007/1-4020-3880-1, 2005.
Siddall, M., Smeed, D. A., Hemleben, C., Rohling, E., Schmelzer, I., and Peltier, W. R.: Understanding the Red Sea response to sea level, Earth Planet. Sc. Lett., 225, 421–434, https://doi.org/10.1016/j.epsl.2004.06.008, 2004.
Taviani, M.: Post-Miocene reef faunas of the Red Sea: glacio-eustatic controls, in: Sedimentation and Tectonics in Rift Basins, Red Sea- Gulf of Aden, Springer Netherlands, Dordrecht, 574–582, https://doi.org/10.1007/978-94-011-4930-3_30, 1998.
Titelboim, D., Almogi-Labin, A., Herut, B., Kucera, M., Asckenazi-Polivoda, S., and Abramovich, S.: Thermal tolerance and range expansion of invasive foraminifera under climate changes, Sci. Rep., 9, 4198, https://doi.org/10.1038/s41598-019-40944-5, 2019.
Turak, E., Brodie, J., and DeVantier, L.: Reef-building corals and coral communities of the Yemen Red Sea, Fauna Arab., 23, 1–40, 2007.
Uthicke, S. and Nobes, K.: Benthic foraminifera as ecological indicators for water quality on the Great Barrier Reef, Estuar. Coast. Shelf Sci., 78, 763–773, https://doi.org/10.1016/j.ecss.2008.02.014, 2008.
Uthicke, S., Thompson, A., and Schaffelke, B.: Effectiveness of benthic foraminiferal and coral assemblages as water quality indicators on inshore reefs of the Great Barrier Reef, Australia, Coral Reefs, 29, 209–225, https://doi.org/10.1007/s00338-009-0574-9, 2010.
Vine, P. J.: The Red Sea, Immel Publishing, London and Jeddah, 128 pp., ISBN 13 9780907151104, 1985.
Walton, W. R.: Techniques for recognition of living foraminifera, Contrib. Cushman Found. Foraminifer. Res., 3, 56–60, 1952.
Wilkinson, C.: Status of coral reefs of the world: 2008, Global Coral Reef Monitoring Network and Reef and Rainforest Research Centre, Townsville, Australia, 296 pp., https://icriforum.org/wp-content/uploads/2019/12/GCRMN_Status_Coral_Reefs_2008.pdf (last access: 5 July 2024), 2008.
Williams, R. A.: Comparing reef bioindicators on benthic environments off southeast Florida, College of Marine Science, University of South Florida, 60 pp., https://digitalcommons.usf.edu/etd/87 (last access: 5 July 2024), 2009.
Youssef, M.: Heavy metals contamination and distribution of benthic foraminifera from the Red Sea coastal area, Jeddah, Saudi Arabia, Oceanologia, 57, 236–250, https://doi.org/10.1016/j.oceano.2015.04.002, 2015.
Zubaida, V., Sartimbul, A., and Natsir, S. M.: Study of benthic foraminifera and its connection with environmental condition in Bidadari Island, KepualauanSeribu, IOP Conf. Ser. Earth Environ. Sci., 429, 012007, https://doi.org/10.1088/1755-1315/429/1/012007, 2020.