the Creative Commons Attribution 4.0 License.
the Creative Commons Attribution 4.0 License.
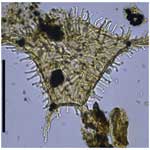
High Arctic late Paleocene and early Eocene dinoflagellate cysts
Henk Brinkhuis
Palynomorphs, notably sporomorphs and organic-walled dinoflagellate cysts, or “dinocysts”, are the only abundant microfossils consistently present in the sole available central Arctic upper Paleocene to lower Eocene sedimentary succession recovered at the central Lomonosov Ridge by the Integrated Ocean Drilling Program (IODP) Expedition 302 (or the Arctic Coring Expedition, ACEX) in 2004, close to the North Pole. While the analysis and interpretation of a part of these assemblages have so far guided many major stratigraphic, climatological, and paleoenvironmental findings from ACEX, intrinsic details, notably of the dinocyst taxa and assemblages, have not yet been addressed. Here, we present new ACEX dinocyst data for the interval spanning the latest Paleocene to the earliest Eocene (∼56.5–53.8 Ma; cores 32X–27X) and integrate these with previous results. We develop a pragmatic taxonomic framework, document critical biostratigraphic events, and propose two new genera (Guersteinia and Sangiorgia) and seven new species (Batiacasphaera obohikuenobeae, Chaenosphaerula sliwinskae, Heterolaucacysta pramparoae, Pyxidinopsis iakovlevae, Sangiorgia pospelovae, Sangiorgia marretiae, and Spiniferella crouchiae). In addition, we interpret trends and aberrations in dinocyst assemblages in terms of variability in regional temperature, hydrology, and tectonism across the long-term and the Paleocene–Eocene Thermal Maximum (PETM) and Eocene Thermal Maximum 2 (ETM2) global warming phases.
- Article
(15830 KB) - Full-text XML
-
Supplement
(30576 KB) - BibTeX
- EndNote
The partially recovered sedimentary record from the central Lomonosov Ridge, Arctic Ocean (Integrated Ocean Drilling Program (IODP) Expedition 302, or Arctic Coring Expedition (ACEX); Fig. 1) yielded a wealth of new information concerning early Paleogene Arctic paleoclimates, environments, and biogeochemical cycling (Backman et al., 2006). In lower Paleogene sediments overlying an upper Cretaceous unconformity, early work identified the Paleocene–Eocene Thermal Maximum (PETM; ∼56 Ma) (Sluijs et al., 2006), the Eocene Thermal Maximum 2 (ETM2; 54 Ma) (Stein et al., 2006; Sluijs et al., 2008b), and the Azolla phase (48 Ma; Brinkhuis et al., 2006). These, and many follow-up contributions, revealed that the Arctic was very warm and wet during the early Paleogene, particularly during the PETM and ETM2 (e.g., Willard et al., 2019; Sluijs et al., 2020). Salinities were low throughout, and significant freshening occurred during the PETM, ETM2, and the Azolla phase (e.g., Brinkhuis et al., 2006; Pagani et al., 2006; Waddell and Moore, 2008; Sluijs et al., 2009; Speelman et al., 2010; Krishnan et al., 2014). Various papers have also addressed the strong orbital forcing on Arctic temperature and hydrology (Sangiorgi et al., 2008; Sluijs et al., 2008b; Barke et al., 2011; Fokkema et al., 2024b). During the early Paleogene the drill site was apparently located very close to shore, given the large proportions of terrestrial organic matter throughout the record, likely derived from then-exposed parts of the Lomonosov Ridge and Svalbard (e.g., Sluijs et al., 2008b). Often, low-oxygen, euxinic conditions prevailed at the seafloor, reaching into the lower photic zone during the PETM and ETM2 (e.g., Sluijs et al., 2006, 2009; Stein et al., 2006; März et al., 2011).
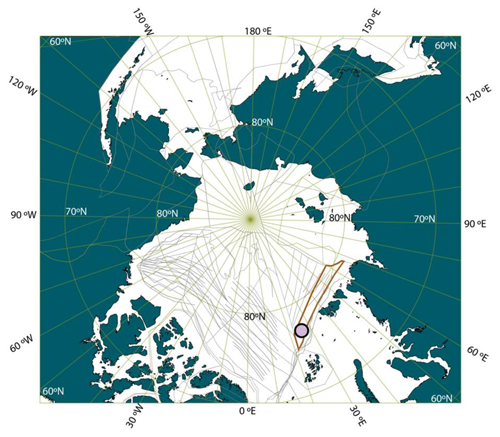
Figure 1Location of ACEX Hole M0004A on a paleogeographic reconstruction of the Arctic region at the time of the PETM. The reconstruction was made using GPlates (Müller et al., 2018), with the tectonic reconstruction of Seton et al. (2012; the red shape is the Lomonosov Ridge in this reconstruction, and the gray lines are structural features, including spreading ridges) and the paleomagnetic reference frame of Torsvik et al. (2012), and the modern coastlines are indicated.
Calcareous and siliceous microfossils are typically scarce to absent in this interval, while, in contrast, palynomorphs are abundant and often well preserved (Backman et al., 2006). These particularly comprise pollen and spores derived from terrestrial higher plants and organic-walled dinocysts, besides a suite of other algal remains, organic linings of benthic foraminifera, and acritarchs (Backman et al., 2006). The analysis and interpretation of a part of these assemblages has guided many of the above major stratigraphic, climatological, and paleoenvironmental conclusions. Recent work has assembled and interpreted the pollen and spore record from the latest Paleocene to just above ETM2 with decimeter-scale resolution (cores 32X–27X; Willard et al., 2019). Here, also to aid future Arctic work, we assemble and integrate the unique ACEX dinocyst data for that same interval, integrating new and existing qualitative and quantitative information from analyses carried out previously (Backman et al., 2006, 2008; Sluijs et al., 2006, 2008b, 2009; Fokkema et al., 2024b).
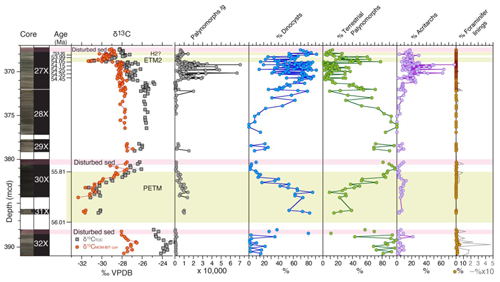
Figure 2Abundances of major palynological groups across the uppermost Paleocene and lowermost Eocene at ACEX Hole 4A. Core photos and codes (Backman et al., 2006); ages and assignments of PETM, ETM, and H2? (Table S1); and stable carbon isotope records (δ13C) of total organic carbon (TOC), corrected to marine organic matter (MOM) by excluding terrestrial organic matter contributions using the BIT index (see Sluijs and Dickens, 2012), are from cited previous work. Pink bars indicate disturbed sediments, caved from overlying strata during coring. Note the double axis in the column showing the percentage of foraminiferal linings to visualize low abundances.
The interval represented by IODP 302 cores 33X to 27X of Hole M0004A straddles the interval from just below the PETM (∼56 Ma) to across the ETM2 and possibly the H2 event (54 Ma; Stein et al., 2006; Sluijs et al., 2008b, 2009) (Fig. 2). Recently, Fokkema et al. (2024b) identified eccentricity cycles in the interval immediately below ETM2 and tuned them to two orbital solutions, thereby refining the age model (see Table S1 for age model tie points).
Here, we compile and integrate all existing and new “raw” palynological data underlying the records published so far from cores 33X to 27X of Hole M0004A (Sluijs et al., 2006, 2008b, 2009; Fokkema et al., 2024b) and present them using an existing composite depth scale (Sluijs et al., 2009). All samples were prepared according to the same, typical palynological processing techniques as described in the papers mentioned above. Briefly, after freeze-drying and precision weighing, this involved processing with HCl and HF treatments, followed by sieving residues over a 15 µm mesh sieve. Samples were spiked with a known amount of Lycopodium clavatum spores to allow absolute quantitative counts (Stockmarr, 1972). Palynomorphs were counted to a minimum of 200 identifiable dinocysts per sample where possible and to a minimum of 100 identifiable dinoflagellate cysts for the high-resolution palynological investigation in the interval immediately below ETM2. For dinocyst taxonomy, we employ the taxonomy cited in Fensome et al. (2019), except for wetzelielloid taxa, where we follow the recommendations of Bijl et al. (2016). Various consistency checks between the data generated by the two authors (HB and AS), including extensive microscope sessions together and re-counting each other's slides, indicated that counts are consistent at the taxonomical level presented, within an uncertainty of 5 %–10 %. We include a taxonomic section (Sect. 6) that describes selected new taxa and provides an annotated species list. All raw data are provided in the Supplement and on Zenodo (https://doi.org/10.5281/zenodo.10998746, version 4) (Sluijs and Brinkhuis, 2024). A high-resolution version of the Supplement photoplates (38 plates with light microscope photos and 3 plates with scanning electron microscope photos) is also available at Zenodo. All materials are stored in the collection of the Laboratory of Palaeobotany and Palynology, Department of Earth Sciences, Utrecht University, the Netherlands.
Palynomorph preservation varies across the section, ranging from (most often) good to poor. The dominant palynological groups throughout the Paleogene ACEX record are dinocysts and the pollen and spores of terrestrial higher plants (Fig. 2). We also recovered many other aquatic algal taxa and acritarchs which were locally quite abundant, sometimes representing up to 30 % of the aquatic palynomorphs. These include Pterospermella spp., Leiosphaera spp., Paleotetradinium spp., Tasmanites spp., Tritonites spinosus, and Botryococcus spp., besides numerous very small (<20 µm) psilate and skolochorate cysts (viz. “acritarchs”). Organic linings of benthic foraminifera and their fragments are relatively abundant in sediments stratigraphically above and below the PETM (Sluijs et al., 2006), with decreasing abundance up-section. Fungal spores were occasionally encountered as well. In terms of palynofacies, several intervals are dominated by amorphous organic matter (AOM), particularly the upper Paleocene, up to the onset of the PETM, the interval directly overlying the PETM (∼381–371 mcd) and the ETM2 interval. Other samples are characterized by a mix of terrestrial plant debris and associated elements. We also regularly encountered pyritized remains of diatoms, Radiolaria, silicoflagellates, and ebridians (Fig. 2).
3.1 Qualitative dinocyst distribution
3.1.1 Peridinioids
Taxonomic issues
Peridinioid cysts with a six-sided (“hexa”) second anterior intercalary (para)plate that is involved in archeopyle formation are a major constituent of the assemblages. However, many specimens could not be assigned to a specific or even generic level, either because diagnostic features have not been described, could not be observed, vary from the features described for existing taxa, or could be interpreted as more than one taxon. Specimens present a continuum of morphological characteristics between the descriptions of Senegalinium, Lentinia, Spinidinium, Cerodinium, and Deflandrea. For example, specimens may range from being “classical” peridinioid-shaped, strongly denticulate with wall striations with steno/isodeltaform 2a archeopyles typical for Cerodinium, to faintly striate or smooth with large isodeltaform 2a archeopyles, adhering more to the definition of Lentinia and ranging into latideltaform 2a archeopyles as in Deflandrea. Other peridinioids may range from being rather small, brown to unpigmented, smooth to granulate cysts without processes, assignable to Senegalinium, to specimens with few to numerous spines typical for Spinidinium. We noted numerous intermediate morphotypes between these taxonomical entities (see Supplement Plates S8–S13, S34–S36). Preservation is suboptimal in several intervals, which also impacts taxonomical assignments. Future detailed taxonomic work on this group could possibly clarify and complement this work, but we surmise that any evolving concepts will remain challenging to apply. The issues assigning specimens to described taxa primarily arise due to the less detailed taxonomic descriptions that were produced prior to the 1980s.
Taxonomic groupings
Despite the taxonomic ambiguity encountered within the peridinioids with hexa-2a plates, the two authors were in reasonable agreement on the generic level, so counts of Cerodinium, Deflandrea, Lentinia, Phthanoperidinium, and Senegalinium reflect reliable results. However, we suggest taking abundance percentages of these genera with care and estimate the uncertainty in absolute percentages to be a maximum of 10 %. We decided to group these genera into two broad “complexes” (cpx). We place the recorded variability in the locally highly abundant “morphological continuum” Cerodinium/Deflandrea/Lentinia spp. in the Cerodinium complex and the Senegalinium/Spinidinium/Phthanoperidinium spp. continuum in the Senegalinium complex (See Plates S8–S13, S34–S36).
Furthermore, in terms of paleoenvironmental signals, we place both these complexes in a “low-salinity-tolerant group” following previous work (Brinkhuis et al., 2006; Sluijs et al., 2006; Frieling and Sluijs, 2018), in which we also include the few occurring (non-cavate) protoperidinioid dinocysts, such as Lejeunecysta spp. and Selenopemphix spp. These cysts are known to be derived from heterotrophic species that tolerate low-salinity waters in modern oceans and also indicate regions of high primary production (e.g., Zonneveld et al., 2013). Some taxa within this large category, notably those assignable to the genus Phthanoperidinium, are known to be tolerant to lower salinities relative to others (e.g., Liengjarern et al., 1980; Barke et al., 2011), but these are not abundant in this part of the ACEX record. Quadra-2A peridinioids (wetzelielloids) such as Apectodinium spp. are not included in this group. These taxa appear more indicative of high surface water temperatures on top of a strong tolerance for variable salinities (Bujak and Brinkhuis, 1998; Crouch et al., 2001; Frieling et al., 2014; Frieling and Sluijs, 2018).
3.1.2 Gonyaulacoids
Taxonomic issues
We recorded many gonyaulacoid species that are relatively stable in their morphology and unequivocally assignable to many existing taxa, so that they appear useful for intra-regional to even global correlations with chronostratigraphic value. We also recorded various undescribed taxa with potential paleoecological or stratigraphical value, which were selected for formal description. These include taxa broadly adhering to the “standard building plan” of the cysts of the modern Gonyaulax spinifera group, viz. “spiniferitioid” cysts, i.e., sexiform gonyaulacoids with an S-type sulcus, tri- and bifurcated gonal and intergonal processes, that are either isolated or distally connected, like in family member Nematosphaeropsis, but differ in having only a part of the processes interconnecting distally with quite pronounced trabeculae. We place these in a new genus, Sangiorgia. Based on the size, nature, and shape of the processes, we distinguish two species within this genus, which we name S. pospelovae and S. marretiae.
Furthermore, we note that some species placed in the genus Thalassiphora up to now are morphologically so distinct that there is a need to erect the new genus Guersteinia to accommodate standard sexiform gonyaulacoid camocavate cysts with a dorso-ventrally compressed periphragm. We designate the holotype of Thalassiphora delicata as the type species of the new genus. Importantly, we note that, by definition, the genus Thalassiphora constitutes (typically large) cavate, fibroid gonyaulacoid cysts characterized by dextral torsion and a lack of (para)tabulation. We consider these to be members of the cribroperidinioid lineage of gonyaulacoids that typically exert dextral torsion of the hypocyst relative to the epicyst, which is not the case in T. delicata (see also Mudie et al., 2020 for an overview of Thalassiphora species and similar deliberations).
In addition, we record an extremely small and psilate new species of Batiacasphaera, which we name B. obohikuenobeae. Furthermore, we encountered a new taxon lacking an endophragm, assignable to Chaenosphaerula, with characteristic, somewhat irregular, tagliatelle-shaped parasutural “bands”, which we name C. sliwinskae. We also encountered a rather thick-walled species with a microreticulate surface with small irregular outgrowths of low relief assignable to Pyxidinopsis, which we name P. iakovlevae.
A well-known and long-ranging taxon encountered is Spiniferella cornuta. However, these Arctic types are extremely large (consistently >125 µm in overall length), with the characteristic long, slightly curved apical horn, but are further distinguished here by their rather long gonal processes, which terminate in trifurcate ends that may individually split again into secondary bifurcations or even trifurcations. We describe this as the new species Spiniferella crouchiae.
Taxonomic groupings
Despite the relatively large number of morphologically stable taxa discussed above, a major portion of the gonyaulacoids also include morphologically intimately related groups that display formidable intra- and interspecific variation. Following Evitt (1985), these gonyaulacoid representatives within our material include forms assignable to his concepts of the partiforms, the insert and exsert quinqueforms (e.g., goniodomids) and sexiforms and the dorso-ventrally compressed (Gv) insert sexiform (areoligeracean) types, besides those assignable to his categories Gx and Gi (typically including small multi-spined skolochorates).
One of the characteristic features of this interval is the ubiquitous presence of representatives of Hystrichosphaeridium, mostly assignable to (subspecies of) H. tubiferum. Previous work (e.g., Marheinecke, 1992; Lentin and Williams, 1993; Fauconnier and Masure, 2004; Niechwedowicz, 2022) has also described the enormous morphological variability in this taxon, ranging in shape from “standard” spherical or oval forms to strongly elongated ones (see Plates S20, S21). Additionally, their processes may vary strongly in width and length and in the nature of the distal terminations, where some species form platform-like extensions that can be either perorate or entire. We will refer to this group as the Hystrichosphaeridium tubiferum complex.
Morphological variation is also large for the areoligeraceans abundantly recorded here, which represent the dorso-ventrally compressed (Gv) gonyaulacoids with an apical archeopyle, like Areoligera and Glaphyrocysta spp. (cf. Evitt, 1985). Such taxa are typically identified on their process expressions and arrangements, from intra- or penitabular unconnected to complexly interconnected distally, e.g., from Areoligera coronata or A. medusettiformis types via Glaphyrocysta ordinata to G. exuberans types. In this material, these morphotypes (“taxa”) form a single, fluid complex (see Plates S5–S7) and have therefore been assigned to a single group (the Areoligera complex). In effect, our Areoligera cpx is identical to that of previous work (Sluijs and Brinkhuis, 2009; Frieling and Sluijs, 2018) and at this location is most often dominated by representatives of Glaphyrocysta, notably G. ordinata. Among the gonyaulacoids, this group is considered to be characteristic of relatively high-energy, near-shore settings (Brinkhuis, 1994; Sluijs and Brinkhuis, 2009; Harding et al., 2011; Frieling and Sluijs, 2018). However, in the assemblages encountered here, they particularly contrast the large abundances of peridinioids with hexa-2A plates, together with a few other gonyaulacoid groups. As such, together with the other gonyaulacoids, in the present setting, they reflect a normal marine shelf environment, as opposed to a setting with very strong freshwater forcing as indicated by the peridinioids with hexa-2A plates.
We have assigned forms to the (poorly established) genus Membranosphaera in previous publications concerning ACEX material (Sluijs et al., 2008b, 2009, 2011; Sluijs and Brinkhuis, 2009) for (mostly) non-tabular holocavate gonyaulacoid cysts with an apical archeopyle. Here we note that the generic name Elytrocysta is more suitable for such specimens (Brinkhuis et al., 2003; Williams et al., 2004; Williams et al., 2017) and assign them here accordingly. Morphotypes in this group regularly show vague to clear expression of the paratabulation in their ornamentation. In addition, partly because preservation is not optimal in all samples, it is not always clear if specimens are holocavate or simply consist of an autophragm with numerous (non-)tabular processes. This occasionally abundant category is principally assignable to the partiforms (Evitt, 1985), from Microdinium or Cladopyxidium types to the morphologically strongly related Elytrocysta and Histiocysta or Graptodinium spp. Consequently, following Sluijs et al. (2008b), the Elytrocysta complex consists here of the genera Elytrocysta, Histiocysta/Graptodinium, and Microdinium and intermediates. Elytrocysta is by far the dominant genus. The paleoecological significance of the Elytrocysta cpx remains rather enigmatic, but cysts in this complex are thought to be derived from open marine, neritic taxa (Frieling and Sluijs, 2018).
Finally, for Spiniferites and associated genera, i.e., sexiform gonyaulacoid, S-type sulcus, with gonal processes with typically trifurcate distal ends and possibly intergonal processes with bifurcate distal ends that distally might connect, we use the Spiniferites complex, following previous work (e.g., Sluijs et al., 2008a; Sluijs and Brinkhuis, 2009; Harding et al., 2011; Frieling and Sluijs, 2018). In all our samples, the bulk of this group represent Spiniferites spp. sensu stricto. This group is typically interpreted to represent marine generalists occurring along the entire inshore-to-offshore transect, relatively abundant in warm open marine settings in the modern period (Wall et al., 1977; Thöle et al., 2023) and in the Eocene (Brinkhuis, 1994; Frieling and Sluijs, 2018).
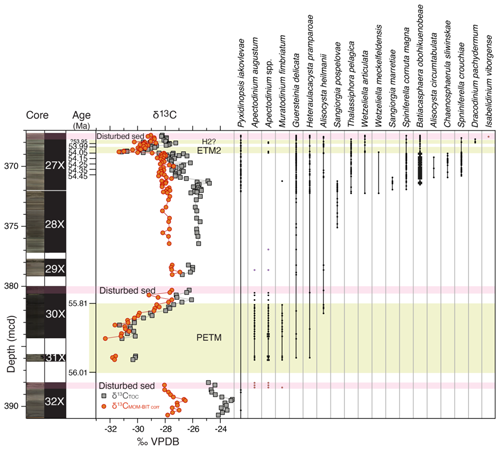
Figure 3Range chart of (potentially) stratigraphically useful first and last (consistent) dinocyst occurrences and the newly described taxa across the uppermost Paleocene and lowermost Eocene at ACEX Hole 4A. Core photos and codes (Backman et al., 2006); ages and assignments of PETM, ETM and H2? (Table S1); and stable carbon isotope records (δ13C) of total organic carbon (TOC), corrected to marine organic matter (MOM) by excluding terrestrial organic matter contributions using the BIT index (see Sluijs and Dickens, 2012), are from cited previous work. Pink bars indicate disturbed sediments, caved from overlying strata during coring. Occurrences shown in orange in these intervals are assumed to reflect downcore contamination due to caving (see text).
Table 1Stratigraphic constraints on recognized dinocyst events (Fig. 3) and their calculated ages. Ages are determined using simple linear interpolations between the tie points of our age model using the ages of 56.0 and 54.0 for the onsets of the PETM and ETM2, respectively, and tuned eccentricity maxima (see Table S1). LO: last occurrence. FO: first occurrence. LCO: last common occurrence.
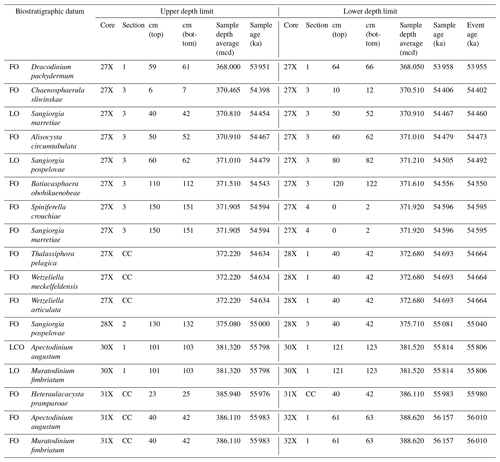
Biostratigraphy
Among both existing and new species, we recorded several empirically established stratigraphically important taxa that can be used for interregional correlations, besides the potentially useful first occurrences (FOs) among the new species (Fig. 3). We treat them chronologically in this section. The calculated ages of these events, using simple linear interpolations between the tie points of our age model using ages of 56.0 and 54.0 for the onsets of the PETM and ETM2, respectively, are presented in Table 1.
Cerodinium wardenense occurs throughout the record and is therefore not shown in Fig. 3. The last occurrence of C. wardenense in the North Sea closely coincides with the top of the H. tubiferum zone of Bujak and Mudge (1994), which is close to calcareous nannofossil zone boundary NP10–NP11. Based on this, the presence of C. wardenense within the carbon isotope excursion (CIE) at ∼369 mcd, first identified by Stein et al. (2006), confirmed this CIE to represent ETM2 (Sluijs et al., 2008b). The new species Pyxidinopsis iakovlevae ranges throughout the studied interval but notably in the upper Paleocene and the interval below ETM2.
Apectodinium augustum, the total range of which is diagnostic for the PETM interval, first occurs in the uppermost 0.5 m of Core 32X, but this interval represents caved sediments from overlying strata. The first in situ occurrence is hence in Core 31X, representing a first occurrence in the core gap at 387.36 (±1.26) mcd, consistent with the onset of the PETM and its carbon isotope excursion (Sluijs et al., 2006). The last consistent occurrence is at 381.41 (±0.1) mcd, which marks the top of the CIE. Isolated specimens recorded above this level might be reworked due to sea level regression after the PETM (Sluijs et al., 2008a, b). The range of A. augustum is approximately consistent with the oldest Apectodinium acme, which is recorded globally during the PETM and – in combination – typically restricted to the CIE (Crouch et al., 2001; Frieling and Sluijs, 2018; Denison, 2021), although it leads the CIE in several records by millennia (Sluijs et al., 2007; Jin et al., 2022). The expression of the Apectodinium acme at the central Lomonosov Ridge is less extreme than in other records around the globe (Bujak and Brinkhuis, 1998; Crouch et al., 2001; Sluijs and Brinkhuis, 2009; Sluijs et al., 2011; Kender et al., 2012; Denison, 2021), with maximum percentages of ∼20. However, these percentages are still diagnostic of the PETM in this section. The drill site seems outside the geographic (or temperature?) range of Apectodinium spp. for the remainder of the studied interval, except for a brief return in two samples at the onset of ETM2 (368.84–368.72 ±0.02 mcd, not A. augustum), corroborating its poleward spread during global warming phases (Bujak and Brinkhuis, 1998; Sluijs et al., 2009). Given the dominance of low-salinity-tolerant dinocysts, we surmise that representatives of Apectodinium were outcompeted due to low salinity and lower temperatures, but it is possible that the genus was more abundant in the Arctic in less proximal/more saline settings during the PETM and ETM2. Interestingly, some specimens of multiple Apectodinium species are remarkably small.
Partly consistent with the stratigraphic distribution of Apectodinium spp. is the occurrence of Muratodinium fimbriatum (Fig. 3). The effective range of this species is restricted to the PETM, apart from a single specimen at 371.20 rm b.s.f. This FO seems consistent with that in various Nordic Sea locations, including the Labrador Sea (Nøhr-Hansen et al., 2016), the North Sea (Powell et al., 1996), and the Turgay Strait (Iakovleva et al., 2001), but this taxon ranges slightly deeper down into the Paleocene at lower-latitude sites, such as the New Jersey shelf (Sluijs and Brinkhuis, 2009) and South Carolina (Edwards, 1998) in the USA, various locations along Tethys margins (Henk Brinkhuis, personal observations, 1985–2025), the eastern equatorial Atlantic Ocean (Frieling et al., 2018b), and India (Prasad et al., 2020), and was originally described from strata close to the PETM (Frieling et al., 2018a) by Cookson and Eisenack (1967). It seems that this species is one of many that shows poleward migration during the PETM (see overview of Frieling and Sluijs, 2018). We do note the conspicuous absence of the morphologically related species, Ifecysta pachyderma, which, at low and mid-latitudes, is typically found in tandem with M. fimbriatum. Empirical evidence suggests that the former was essentially a true tropical variety of this cribroperidinioid group.
Alisocysta heilmannii and the new species Heteraulacacysta pramparoae first occur within PETM strata, but their presence is sporadic. Given the rarity of dinocysts below the PETM, we cannot exclude that these taxa were already present in the region during the Paleocene. The species range throughout the entire studied interval. For A. heilmanii, this is consistent with its range in the North Sea (Heilmann-Clausen, 1985; Casas-Gallego et al., 2021).
The FO of the genus Sangiorgia is represented by the FO of S. pospelovae at 375.40 (±0.31) rm b.s.f., but we have recorded several specimens in the underlying samples that resemble this species. The last occurrence is at 371.11 (±0.05) rm b.s.f.
Several FOs are concentrated near the base of Core 27X, likely implying an FO in the core gap between the top of undisturbed sediments in Core 28X (372.68 rm b.s.f.) and the bottom of Core 27X (372.22 rm b.s.f.). This includes the FO of Thalassiphora pelagica. This interval is also the base of the very short range of Sangiorgia marretiae between 371.97 (±0.05) and 370.86 (±0.05) rm b.s.f. The FOs of Wetzeliella articulata and W. meckelfeldensis are also in this interval, and another useful event among the wetzelielloids includes the FO of Dracodinium pachydermum in Core 27X at 360.02 (±0.03) rm b.s.f. The FOs of these wetzelielloids appear internally consistent with the succession as recorded in the North Sea, Shetland, and Norwegian basins (e.g., Bujak and Mudge, 1994).
Slightly higher up, we detect the FO of Batiacasphaera obohikuenobeae at 371.56 (±0.05) rm b.s.f., after which it rapidly becomes an abundant constituent and dominates assemblages up to the onset of ETM2. The species reappears after ETM2 and ranges up to the top of the studied section, including the upper part that represents caved material, suggesting its range exceeds the studied time interval. In this interval, we also record sporadic Alisocysta circumtabulata, much later than in the North Sea (e.g., Powell et al., 1996). At the same level, we record the first indisputable occurrence of Chaenosphaerula sliwinskae at 369.88 rm b.s.f., but a few cysts resembling this new species were recorded down to 370.9 rm b.s.f. It is never an abundant species, and its occurrence is somewhat scarce up to its LO at 368.89 (±0.01) rm b.s.f. Closely above this FO, we record the FO of the new species Spiniferella crouchiae, which ranges at least to the top of the study interval.
In addition, and somewhat remarkably, we have recorded a single specimen of Isabelidinium viborgense in the top interval of Core 27X, which likely represents caved material. This is a stratigraphic index taxon for the Selandian (e.g., Heilmann-Clausen, 1985; Vieira et al., 2020), which is not present in the ACEX material due to a significant hiatus between the Cretaceous and the late Paleocene (Backman et al., 2006). We therefore conclude that the Selandian was once deposited close to, and presumably at, the drill site, eroded during the early Eocene leading to its reworking, and was subsequently redeposited in caved sediments on top of Core 27X. We surmise it is present in the poorly recovered ∼60 m overlying the studied interval.
3.2 Quantitative dinocyst distribution patterns
3.2.1 Dinocyst paleoecological framework
As indicated above, for our quantitative description of the assemblages, we make effective taxonomic and ecological dinocyst groups derived from modern distributions (e.g., Zonneveld et al., 2013; Thöle et al., 2023) and empirically based paleoecological information of Paleogene dinocysts following previous work (e.g., Brinkhuis, 1994; Powell et al., 1996; Brinkhuis et al., 1998; Pross and Schmiedl, 2002; Crouch and Brinkhuis, 2005; Pross and Brinkhuis, 2005; Sluijs et al., 2005; Eldrett and Harding, 2009; Harding et al., 2011; Frieling and Sluijs, 2018; Vieira and Jolley, 2020; Bijl et al., 2021a; Iakovleva and Heilmann-Clausen, 2021; Jolley et al., 2023). Moreover, we generally follow the quantitative empirical framework of Sluijs and Brinkhuis (2009) and Frieling and Sluijs (2018). Assignment of species to ecogroups is specified in Sect. 6.2.
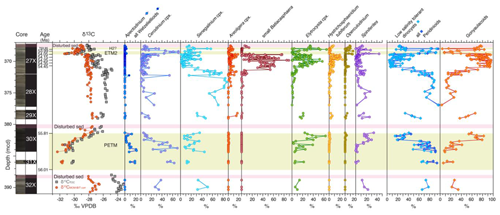
Figure 4Dinocyst (group) abundances across the uppermost Paleocene and lowermost Eocene at ACEX Hole 4A. Core photos and codes (Backman et al., 2006); ages and assignments of PETM, ETM, and H2? (Table S1); and stable carbon isotope records (δ13C) of total organic carbon (TOC), corrected to marine organic matter (MOM) by excluding terrestrial organic matter contributions using the BIT index (see Sluijs and Dickens, 2012), are from cited previous work. Pink bars indicate disturbed sediments, caved from overlying strata during coring.
3.2.2 Dinocyst distribution patterns
Dinocyst assemblages in the uppermost Paleocene (Core 32X), several intervals between the PETM and ETM2, notably the uppermost 1 m of Core 30X, parts of Core 29X, and the lowermost 2 m of Core 28X, are very low in diversity and are dominated by representatives of the hexa-2A peridinioid Senegalinium cpx and Cerodinium cpx with minor contributions from the gonyaulacoid Spiniferites spp. and the Elytrocysta cpx (Fig. 4). Palynological assemblages in these intervals are overwhelmingly dominated by terrestrial palynomorphs (Fig. 2). Dominant amorphous organic matter (AOM) is considered to be of terrestrial origin given the dominance of other terrestrial components such as cuticles and pollen. Marine components are so rare that dinocyst assemblages are unquantifiable in many samples. During both the PETM and ETM2, the Cerodinium cpx dominates assemblages, also with abundant Apectodinium spp. in the PETM interval. Dinocyst taxonomic diversity is also much greater during the PETM.
We find much more diversity among gonyaulacoids, notably B. obohikuenobeae, the Elytrocysta cpx, the Areoligera cpx, and the Hystrichosphaeridium tubiferum cpx, besides Operculodinium spp. and Spiniferites spp., in the interval between 371.35 (±0.05) mcd and the onset of ETM2. This interval represents the time during which the drill site was located most distally from the coast in the Paleogene section of the ACEX record, suggesting a relation between abundance and relative sea level (Sluijs et al., 2009; Fokkema et al., 2024b). Indeed, small Batiacasphaera cysts, such as B. obohikuenobeae, are typically characteristic of open-ocean conditions throughout the Cenozoic (e.g., Schreck et al., 2013). Together with the other gonyaulacoid species, we therefore consider their paleoecology as “open marine”, thereby contrasting them to the low-salinity-tolerant group.
Although members of the Cordosphaeridium fibrospinosum complex (Brinkhuis and Schiøler, 1996; Sluijs and Brinkhuis, 2009; Frieling and Sluijs, 2018) are present in the studied material, they never dominate assemblages. Similarly, we encounter some Goniodomidae with an epicystal archeopyle, notably Eocladopyxis, Heteraulacacysta, and Polysphaeridium, but never as a significant component of the assemblage. This contrasts with records from time-equivalent locations globally (e.g., Crouch and Brinkhuis, 2005; Sluijs and Brinkhuis, 2009; Sluijs et al., 2011; Frieling et al., 2018b) and suggests that environmental conditions, perhaps temperature, light regime, and salinity, were not optimal for these groups.
4.1 Hydrological change, sea level rise, and eutrophication during the PETM
Previous work has already described (1) the terrestrially dominated latest Paleocene with a dominance of cysts derived from low-salinity-tolerant dinoflagellates, (2) the change to a more open marine palynological assemblage but with the persistence of the low-salinity-tolerant taxa during the PETM, and (3) the first abundances of various “open marine” gonyaulacoid taxa in its aftermath (Sluijs et al., 2006, 2008b). These three phases imply a change from a very proximal position of the drill site relative to the coast with limited marine influence during the late Paleocene to a more offshore position during the PETM due to sea level rise but with persistently low salinities due to the invigorated hydrological cycle. The incursion of “open marine” gonyaulacoid taxa during the recovery of the PETM suggests an increase in salinity but a still higher relative sea level. Above the PETM, palynological assemblages are again dominated by terrestrial material, suggesting a sea level return to the late Paleocene situation.
We note that dominant Senegalinium cpx prior to the PETM is replaced by dominant Cerodinium cpx during the PETM (Fig. 4), notably by Cerodinium spp. Both of these genera and complexes are considered to be produced by dinoflagellates that were tolerant to low salinities and preferred relatively nutrient-rich environments, and they are typically treated as a single paleoecological group termed hexa-2A peridinioids or Senegalinium complex (Frieling and Sluijs, 2018, and references therein). Previously, a distinction within this larger group was made regarding the degree of tolerance to low salinities, with species of Phthanoperidinium being more tolerant than Senegalinium based on patterns recorded in the Azolla phase at the Lomonosov Ridge, when the sea surface became (intermittently) fresh (Barke et al., 2011). Analogously, it is possible that a shift towards lower salinities benefited representatives of Cerodinium more than Senegalinium spp., but, given the abundances of Senegalinium spp. during the Azolla interval (Barke et al., 2011) and much lower abundances of specimens of Cerodinium, this seems unlikely. Similarly, given the cosmopolitan distribution of both taxa, we do not surmise a strong influence of PETM warming on their relative abundances. Rather, we surmise that representatives of Cerodinium were able to benefit more than their Senegalinium counterparts from sea surface eutrophication following more intense food and nutrient supply from land during the PETM (e.g., Sluijs et al., 2008b), or the two groups were targeting other food sources or types.
Supporting evidence for these hydrological and productivity changes during the PETM comes from hydrogen isotope records of higher-plant n-alkanes (Pagani et al., 2006), a vast increase in sediment accumulation rates (Sluijs et al., 2008b), TOC enrichments (Sluijs et al., 2006; Stein et al., 2006), and ample evidence for water-column and seafloor deoxygenation resulting from this hydrological change, warming, and eutrophication (Sluijs et al., 2006, 2008b; März et al., 2010).
4.2 Sea level change between the PETM and ETM2
In strata overlying the PETM, palynological associations are dominated by terrestrial material and palynomorphs, and dinocysts are often too diluted to quantify assemblages, particularly in the upper part of Core 30X and the lower part of Core 28X (Fig. 2). The dominance of Cerodinium cpx and Senegalinium cpx (Fig. 4), cysts derived from low-salinity-tolerant dinoflagellates, confirms a setting proximal to the coastline. The drill site was located somewhat more distally during the deposition of sediments recovered in Core 29X.
In the lower 1 m of Core 27X, the interval between ∼54.7 and 54.5 Ma, gonyaulacoid taxa become increasingly abundant (Fig. 4) and the dominance of terrestrial palynomorphs is gradually replaced by marine palynomorphs (Fig. 2), suggesting an increasingly distal position of the drill site relative to the coast. This is consistent with a previously recorded rise in sea level (Fokkema et al., 2024b). While sediments below this transgressive surface were likely deposited just below wave base, given the lack of marine indicators, the overlying interval represents the most distal setting of the study interval and biomarker assemblages suggest water depths of significantly more than 200 m (Fokkema et al., 2024b). Transgression is also supported by the high glauconite content of the sediments. Glauconite formation requires formation of thousands of years at the sediment–water interface (Prothero and Schwab, 2004). The associated sediment starvation is interpreted to reflect shoreward migration of sedimentation.
4.3 Cosmopolitanism among early Eocene dinocyst taxa
The relatively diverse, open marine assemblages between 371–369 mcd (54.5–54.0 Ma) are not only unique for the upper Paleocene–lower Eocene section, but also in the broader context of Paleogene dinocyst assemblages in the ACEX record, which are typically dominated by hexa-2A peridinioids (Sangiorgi et al., 2008; Sluijs et al., 2008b; Barke et al., 2011). The latter are still abundant in a few samples but typically comprise between 5 % and 20 % of the assemblage, with higher values in only a few samples. Gonyaulacoid taxa, typically B. obohikuenobeae and sometimes Elytrocysta spp., usually dominate assemblages. Moreover, Spiniferites spp., Glaphyrocysta ornata (Areoligera cpx.), and Hystrichosphaeridium tubiferum cpx are common in almost all samples, as are regular abundances of Operculodinium spp.
Abundances of Spiniferites spp., Areoligera cpx, Elytrocysta spp., and Hystrichosphaeridium tubiferum cpx are common in numerous early Eocene mid- to high-latitude sites of the Northern (Powell et al., 1996; Iakovleva et al., 2001; Eldrett and Harding, 2009; Sluijs and Brinkhuis, 2009; Rush et al., 2023) and Southern hemispheres (Crouch et al., 2020; Bijl et al., 2021a, b; Premaor et al., 2023). Many of these aspects, notably representatives of Spiniferites cpx, Operculodinium spp., and Areoligera cpx, are common to abundant constituents in low-latitude regions as well (Gregory and Hart, 1995; Crouch et al., 2003; Frieling et al., 2018b; Steeman et al., 2020). This confirms that the taxa recorded in this section, including the hexa-2A peridinioids, were truly cosmopolitan, inhabiting shallow marine settings from the Antarctic shelf to the Lomonosov Ridge. The occurrence of truly global distributions in any dinocyst in geological time is remarkable given the strong meridional biogeographic separation of dinocyst taxa in the modern period (Zonneveld et al., 2013). The global occurrence of so many taxa that often dominate assemblages worldwide during the early Eocene implies that the extremely low temperature gradients in this time period (Huber and Caballero, 2011; Cramwinckel et al., 2018; Hollis et al., 2019; Fokkema et al., 2024) were likely associated with low global meridional marine biodiversity gradients and that temperature may not have been the dominant forcing of marine biogeographic patterns, in contrast to the present day.
4.4 ETM2
Previous work on ETM2 already indicated a vast drop in sea surface salinity, as indicated by the loss of normal marine taxa, the dominance of the low-salinity-tolerant group, and an increase in the supply of terrestrial pollen and spores through increased run-off (Fig. 3, Sluijs et al., 2009). The recent insight that the water depth of the drill site significantly exceeded 200 m during this phase (Fokkema et al., 2024b), which must have also increased the distance of the site to the coastline, makes this response even more remarkable. It not only suggests that run-off increased in coastal areas, but also that larger areas of the surface ocean, even those located somewhat more distally, were insufficiently salty for normal marine dinoflagellates to thrive.
Like the PETM, the largest abundance increase during ETM2 was in numbers of representatives of the Cerodinium cpx. Analogous to the PETM (see Sect. 4.1), this may have resulted from an increased supply of food and nutrients from land through intensified run-off. The abundance of amorphous organic matter and TOC enrichments within ETM2 is consistent with this interpretation, although this is likely a combined effect of supply and enhanced preservation due to the development of anoxic conditions at the seafloor and in the water column (Stein et al., 2006; Sluijs et al., 2009).
We develop a pragmatic taxonomy and paleoecological grouping of late Paleocene–early Eocene dinoflagellate cysts encountered in sediments recovered during the IODP ACEX expedition on the Lomonosov Ridge, Arctic Ocean, representing the first and only (academic) Arctic record for this time interval. We describe two new dinocyst genera and six new species. We record several first occurrences of species, including several new ones, which may have value for regional or global biostratigraphic correlations.
Strong variations in the relative abundance of terrestrial and marine palynomorphs show evidence of sea level variability, notably related to eustatic rise during the PETM at ∼56 Ma, and a likely much larger, likely tectonic-forced sea level rise at ∼54.5 Ma. Both during the PETM and ETM2, we record the influx of thermophilic species into the Arctic region, notably Apectodinium, Muratodinium fimbriatum, and also several other wetzelielloids during ETM2. The events are further marked by strong freshwater forcing, as indicated by freshwater-tolerant taxa peridinioid dinoflagellates. We suggest that the notable increase in Cerodinium spp. within this freshwater-tolerant group may be related to eutrophication.
We also note that components of the dinocyst assemblages are identical to those at the adjacent Nordic seas, the mid-latitudes, and the tropics all the way to the Southern Ocean. The global occurrence of so many taxa that often dominate assemblages worldwide during the early Eocene implies that the extremely low meridional temperature gradients during this time period were likely associated with low global meridional marine biodiversity gradients. In contrast to the modern period, temperature may not have been the dominant forcing of marine biogeographic patterns.
We formally describe the new genera and species in Sect. 6.1, of which photographs of holotypes and paratypes are presented in Sect. 6.2. Section 6.3 includes a complete species list with remarks and taxonomical and ecological grouping, supported by an extensive collection of photographs in the Supplement (low-resolution version; high resolution available at Zenodo (Sluijs and Brinkhuis, 2024, version 4: https://doi.org/10.5281/zenodo.10998746).
6.1 Systematic paleontology
-
Division Dinoflagellata (Bütschli, 1885; Fensome et al., 1993)
-
Class Dinophyceae (Pascher, 1914)
-
Subclass Peridiniphycidae (Fensome et al., 1993)
-
Order Gonyaulacales (Taylor, 1980)
-
Suborder uncertain
-
Family uncertain
-
Genus Batiacasphaera (Drugg, 1970; emend. Morgan, 1975)
-
Batiacasphaera obohikuenobeae sp. nov.
Plates 1, S3, S4, S39
Derivation of name. Named for Francisca Oboh-Ikuenobe, in recognition of her achievements in marine palynology, Missouri State University of Science and Technology, Rolla, Missouri, USA.
Diagnosis. A small, spherical, and thin-walled gonyaulacoid cyst with an autophragm only. The cyst surface appears completely smooth to psilate, to incidentally microgranulate under light microscopy. SEM analyses show a micrometer-scale reticulate surface (see Plate S39). Tabulation is only apparent from the archeopyle, which is formed by the release of all the apical paraplates (tA, compound) and is distinctly angular. The paraplates reflecting 2' and 3' are much larger than 1' and 4'. The operculum is usually detached, but sometimes plate 1' remains attached to the anterior sulcal plate.
Holotype. Plate 1, A–C
Paratypes. Plate 1, D–F, G–H
Material. IODP 302 Hole M0004A, Lomonosov Ridge, Arctic Ocean
Type locality and horizon. IODP 302 Hole M0004A, Lomonosov Ridge, Arctic Ocean, lower Eocene Core 302-0004A-27X-03-04 cm.
Age. Early Eocene
Description.
-
Shape: (sub)spherical.
-
Wall relationships: autophragm only.
-
Wall features: appears completely smooth to psilate, to incidentally microgranulate under light microscopy. SEM analyses show a micrometer-scale reticulate surface.
-
Processes: absent.
-
Paratabulation: indicated by archeopyle only.
-
Archeopyle: formed by the release of all the apical paraplates (tA, compound); distinctly angular. The paraplates reflecting 2' and 3' are much larger than 1' and 4'. The operculum is usually detached, but sometimes plate 1' remains attached to the anterior sulcal plate.
-
Paracingulum: indeterminable.
-
Parasulcus: indeterminable.
Dimensions. Diameter 18–25 µm (n=10).
Holotype. 22 µm.
Paratypes. 23 µm.
Stratigraphic range/occurrence. Early Eocene
Remarks. In our material, we locally encountered numerous small, round, thin-walled, colorless, psilate to microgranulate gonyaulacoid cysts with an apical archeopyle, which must be included in the genus Batiacasphaera. Using light microscopy, the apical archeopyle is often folded in such a way that it strongly resembled a Type 3I, but SEM micrography confirmed type TA. The species resembles representatives of Batiacasphaera often encountered in Neogene open -ocean sediments, such as B. micropapillata, B. minuta, and B. sphaerica, but it differs in its extremely small size and its general lack of ornamentation when viewed using light microscopy.
-
Genus Pyxidinopsis (Habib, 1975; emend. Stover and Evitt, 1978)
-
Pyxidinopsis iakovlevae sp. nov.
Plates 5, 6, S25, 26, 27
Derivation of name. Named for Alina I. Iakovleva, in recognition of her achievements in marine palynology, at the Geological Institute, Russian Academy of Sciences, Moscow, Russian Federation
Diagnosis. A species of Pyxidinopsis, intermediate in size, with a relatively thick, punctate to most often microreticulate surface, characterized by frequent small, solid, irregularly shaped and distributed rod-like features. No other surface ornamentation present. Archeopyle relatively large, precingular, type P (3′′ only); operculum free. Paratabulation indicated by archeopyle only. No traces of paracingulum or parasulcus.
Holotype. Plate 6, A–C
Paratypes. Plate 5, A–C, D–E, F–H; Plate 6, D–E, F–G
Material. IODP 302 Hole M0004A, Lomonosov Ridge, Arctic Ocean
Type locality and horizon. IODP 302 Hole M0004A, Lomonosov Ridge, Arctic Ocean, upper Eocene, sample 302-4A-27X-3-20/22
Age. Late Paleocene–early Eocene
Description.
-
Shape: subspherical, almost circular to ovoid in outline. Wall relationships: autophragm only.
-
Wall features: a relatively thick autophragm, with a punctate to microreticulate surface, with frequently occurring small, irregularly distributed solid, rod-like features and a maximum height of ∼3 µm. These features may be simple and straight to distally T-shaped.
-
Processes: absent.
-
Paratabulation: indicated by archeopyle only.
-
Archeopyle: relatively large, precingular, type P (3′′ only); operculum free.
-
Paracingulum: indeterminable.
-
Parasulcus: indeterminable.
Dimensions. Range: overall diameter 38(45)54 µm (n=5).
Holotype. 42 µm.
Paratypes. 45 µm.
Stratigraphic range/occurrence. Upper Paleocene–lower Eocene.
Remarks. The original description of Pyxidinopsis does not include any kind of projections, spines, or other possible features on the periphragm (Habib, 1975). Stover and Evitt (1978) noted that the type species, and others in the genus, most often have (micro)reticulated surfaces. Furthermore, several taxa subsequently attributed to Pyxidinopsis, even the type species, P. fairhavenensis (cf. De Verteuil and Norris, 1996), have additional wall features like coni or small projections, but none of them are like those displayed by P. iakovlevae. These features would not support assignment to Operculodinium, since the rod-like extensions are too few and of very low relief compared to the typically longer spines in taxa of the latter. Attribution to Xenicodinium (Klement, 1960) is refuted, since that genus is even less well defined than Pyxidinopsis (e.g., with an uncertain archeopyle).
-
Suborder Goniodomineae (Fensome et al., 1993)
-
Family Goniodomaceae (Lindemann, 1928)
-
Subfamily Goniodomoideae (autonym)
-
Genus Heteraulacacysta (Drugg and Loeblich, 1967; emend. Bujak in Bujak et al., 1980)
-
Heteraulacacysta pramparoae sp. nov.
Plates 3, 4, S18, S19
Derivation of name. Named for Mercedes Beatriz Pramparo, in recognition of her achievements in marine palynology, at IANIGLA, CCT-CONICET, Mendoza, Argentina.
Diagnosis. A species of Heteraulacacysta characterized by very thin wall layers, with isolated small rods or pillars, or rows of small “pillars”, supporting the periphragm, notably around the paracingulum. Archeopyle epicystal, Type tAtP. The periphragm often appears wrinkled, but on some specimens this may faintly indicate paratabulation, overall supporting assignment to the Goniodomidae.
Holotype. Plate 3, A–H
Paratypes. Plate 4, A–C, E–H
Material. IODP 302 Hole M0004A, Lomonosov Ridge, Arctic Ocean
Type locality and horizon. IODP 302 Hole M0004A, Lomonosov Ridge, Arctic Ocean, lower Eocene sample 302-4A-27X-3-90/92 cm
Age. Early Eocene
Description.
-
Shape: (sub)spherical; outline circular to oval in apical/antapical view; combination epicystal archeopyle, Type tAtP.
-
Wall relationships: two wall layers are apparent; a thin endophragm and a periphragm are closely appressed over large parts of the cysts (“autophragm”, as per Bujak in Bujak et al., 1980). The periphragm is occasionally supported by isolated small rod-like features, or small “pillars”, or even rows of these, most notably around the paracingulum.
-
Wall features: both wall layers appear completely smooth to psilate, to incidentally microgranulate under light microscopy, except for the rod-like or pillar-like features of low relief that may occur in rows connecting the two wall layers, most notably around the paracingulum.
-
Processes: absent.
-
Paratabulation: indicated by the tAtP archeopyle, sometimes by conspicuous folds of the periphragm, and potentially in combination with the rows of small “pillars”, which may also reflect sutural growth bands.
-
Archeopyle: formed by the release of all the apical and precingular paraplates (tAtP, compound).
-
Paracingulum: clearly featured by septa formed by the periphragm, supported by rows of small pillars connecting to the endophragm.
-
Parasulcus: indicated by a small incursion in the paracingular septae only.
Dimensions. Length/width 58×85 µm (n=10).
Holotype. 61×77 µm.
Paratypes. 62×75 µm.
Stratigraphic range/occurrence. Early Eocene
Remarks. Wall morphologies, ultrastructure, and wall relationships in Heteraulacacysta and in the very similar genus Dinopterygium (Deflandre, 1935). Stover and Evitt (1978) are still unclear on most species of this group of Goniodomidae. SEM/TEM analyses will be required to fully resolve such issues. The “pillars”, and notably the rows of them, including the connections supporting the paracingular rim, separate H. pramparoae from all other described Heteraulacacysta species.
-
Suborder Gonyaulacineae
-
Family Gonyaulacaceae
-
Subfamily Gonyaulacoideae
-
Genus Chaenosphaerula (Damassa, 1997)
-
Chaenosphaerula sliwinskae sp. nov.
Plate 2, S15, S16
Derivation of name. Named for Kasia K. Sliwinska in recognition of her achievements in marine palynology and organic geochemistry at the Geological Survey of Denmark and Greenland (GEUS), Copenhagen, Denmark.
Diagnosis. Gonyaulacoid cysts that are solely comprised of a periphragmal parasutural network; a central body (or endophragm) is not present. These match the criteria for attribution to Evittosphaerula (Manum, 1979) or Chaenosphaerula (Damassa, 1997). The network in this case is characterized by relatively broad, irregularly shaped bands, much like the Italian pasta, tagliatelle, typical for Chaenosphaerula. Occasionally, fragments of intratabular areas, attached to the parasutural “ribbons”, may be present as well. Reflected paratabulation appears gonyaulacean, matching that surmised for Chaenosphaerula. Archeopyle is uncertain due to the absence of all plates but most likely involves 3”.
Holotype. Plate 2, A–C
Paratypes. Plate 2, D–F, G–H
Material. IODP 302 Hole M0004A, Lomonosov Ridge, Arctic Ocean
Type locality and horizon. IODP 302 Hole M0004A, Lomonosov Ridge, Arctic Ocean, lower Eocene, Core 302-0004A-27X-2-0/1
Age. Early Eocene
Description.
-
Shape: subspherical.
-
Wall relationships: solely comprise a parasutural network; central body is not present.
-
Wall features: parasutural network comprising relatively broad bands, reminiscent of the pasta type, tagliatelle, delineating a standard gonyaulacoid tabulation. Parts of intratabular areas may be represented by smooth and thin wall material.
-
Processes: absent.
-
Paratabulation: indicated by parasutural network representing the periphragm, delineating a standard gonyaulacoid tabulation.
-
Archeopyle: unclear because only parasutural strands are represented, but, given the presence of all parasutures, the archeopyle may be a single-plate, presumably precingular, Type P (3” only).
-
Paracingulum: indicated by parasutural strands.
-
Parasulcus: indicated by parasutural strands.
Dimensions. Diameter 65(73)85 µm (n=5).
Holotype. 80 µm.
Paratypes. 75 µm, 65 µm.
Stratigraphic range/occurrence. Early Eocene
Remarks. The species is distinct from Chaenosphaerula magnifica by its much broader and more irregularly shaped parasutural strands.
-
Guersteinia gen. nov.
Type species.
-
Guersteinia delicata comb. nov. Williams and Downie (1966), p. 235, Pl. 26, Fig. 8
Basionyms.
-
Thalassiphora delicata (Williams and Downie, 1966)
-
Disphaeria delicata (Norvick, 1973)
-
Thalassiphora delicata (Eaton, 1976)
-
Thalassiphora delicata (Lentin and Williams, 1977)
-
Thalassiphora delicata (Bujak in Bujak et al., 1980)
Derivation of name. Named for G. Raquel Guerstein in recognition of her achievements in marine palynology, at the Instituto Geológico del Sur (INGEOSUR), Departamento de Geología, Bahia Blanca, Argentina.
Diagnosis. Guersteinia accommodates intermediate to large, thin-walled, camocavate, non-cribroperidinioid sexiform gonyaulacoid cysts. The small endocyst is subspherical to ellipsoidal, and the dorso-ventrally compressed, often partially tabulated periphragm is connected to the endocyst dorsally but strongly separated ventrally. It forms an umbrella-like structure. Archeopyle is precingular, formed by the detachment of paraplate 3”. Operculum free.
Description.
-
Shape: central body (endophragm) and periphragm appear subspherical from a dorsal and ventral view, which is how it is typically oriented in slides for light microscopy.
-
Wall relationships: cysts camocavate. Endophragm and periphragm are appressed in the mid-dorsal area but strongly separated ventrally so that the dorso-ventrally compressed periphragm forms an umbrella-like structure.
-
Wall features: endophragm typically thin and smooth, occasionally scabrate. Parasutural features (e.g., traces or low ridges) are often present on the periphragm. Major paraplate boundaries appear to be at least partially reflected, indicating a standard symmetric gonyaulacoid pattern, i.e., not cribroperidinioid displaying dextral torsion.
-
Processes: absent.
-
Paratabulation: often at least partially indicated by parasutural septa and/or low ridges on the periphragm, delineating a standard symmetric sexiform gonyaulacoid tabulation.
-
Archeopyle: type P (3” only), detached.
-
Paracingulum: often indicated by parasutural features on the periphragm.
-
Parasulcus: typically indicated by parasutural ridges on the periphragm.
-
Size: intermediate to very large.
Re-attributed species.
-
Guersteinia delicata gen et comb. nov. Plate S17, S41
-
Guersteinia lacunata (Vieira et al., 2018) comb. nov.
-
Guersteinia succincta (Morgenroth, 1966) comb. nov.
Remarks. In a recent paper, Mudie et al. (2020) discussed wall relationships within species of Thalassiphora at length while describing T. subreticulata and T. balcaniensis. They provide a detailed overview of all “accepted” species included in the index of Fensome et al. (2019), noting differences in wall structure, size range, type location, and age (see their Table 2, p. 260). Importantly, in that study, the authors follow Fensome et al. (1993), who regard Thalassiphora as a part of the subfamily Cribroperidinioideae of the family Gonyaulacaceae. This subfamily is mainly characterized by having an “offset” hypocyst, reflecting “dextral torsion” relative to the “symmetric” sexiform gonyaulacoids. However, Mudie et al. (2020, p. 257) also note that, on some specimens, where visible, T. balcaniensis may be in fact be characterized as reflecting standard gonyaulacean organization rather than being cribroperidinioid. Moreover, they note that, within typical Thalassiphora, i.e., as in the type species T. pelagica and allies, the wall structures are typically thick, fibrous, and without clear reflection of paratabulation. In addition, these taxa often also show an antapical protrusion of some kind.
In the ACEX material, specimens assignable to T. delicata are numerous. While we can mention large variability in overall size, all these specimens may be characterized by having a distinctly thin endophragm and periphragm, with the latter showing clear paratabulation (Plate S17 and SEM image Plate S41, g). The reflected pattern is somewhat difficult to interpret because of the massive “inflation” of the periphragm. However, the typical sexiform organization of the sulcal plates and 1”” may often be discerned, and, from there, it follows that epicystal major plate sutures are reflected as well. Importantly, this taxon does not display the dextral torsion of the hypocyst relative to the epicyst that is characteristic of the cribroperidinioid lineage but rather a standard gonyaulacoid. Furthermore, its (thin) wall, the absence of an antapical protrusion, and the well-reflected paratabulation set it apart from Thalassiphora and its type species T. pelagica (Eisenack and Gocht, 1960) and from the many morphologically related genera such as Muratodinium, Cordosphaeridium, Ifecysta, and Damassadinium. Hence, based on the standard sexiform gonyaulacoid paratabulation reflected on the periphragm of the many recorded specimens of T. delicata and on the holotype (Williams and Downie, 1966, p. 235, Pl. 26, Fig. 8, refigured in Bujak et al., 1980, Pl. 8, Fig. 10) and its typical transparent, thin walls, we conclude that these aspects do not match the definition of the genus Thalassiphora. We therefore erect Guersteinia to accommodate this widely recorded taxon.
Recently, Vieira et al. (2018) described Thalassiphora lacunata from the Paleocene of NW Europe, a taxon they already considered to be morphologically closely related to delicata. Indeed, we concur, and we attribute that species here to Guersteinia.
Differential diagnosis. Guersteinia differs from Thalassiphora in its standard sexiform plate organization rather than the dextral torsion as recorded in all taxa within the cribroperidinioid lineage. The periphragm of Guersteinia is typically larger than its endocyst and is dorso-ventrally compressed, which sets it apart from Lophocysta (Manum, 1979). It differs from Invertocysta (Edwards, 1984) by the dorsally appressed endophragm and periphragm, rather than vice versa.
There are more thin-walled cavate gonyaulacoid taxa currently assigned to Thalassiphora, like T. rota (Schiøler, 2005), that clearly lack the typical overall features manifest for the former genus. Other superficially similar cavate gonyaulacoid taxa have been placed in other genera (either formally or in “open nomenclature”), e.g., Invertocysta, Gelatia, Saturnodinium, Dalella, and Lophocysta and perhaps even Aiora and likely others. For these, further studies should confirm a more precise allocation.
-
Sangiorgia gen. nov.
Type species. Sangiorgia pospelovae gen. et sp. nov. (Plates 9, 10, S30, S31, S32, 33)
Derivation of name. Named for Francesca Sangiorgi in recognition of her achievements in marine palynology, at the Laboratory of Palaeobotany and Palynology, Department of Earth Sciences, Utrecht University, the Netherlands.
Diagnosis. Cysts proximochorate to skolochorate; intermediate to large in size, body subspherical, bearing isolated gonal, relatively large, solid processes, of which many, but typically not all, distally interconnect. Such processes are distally interconnected with ribbon-like features, sometimes evolved into “tramlines” typical for Nematosphaeropsis and sometime single connections such as in Cannosphaeropsis, and these connections may be locally clustered on larger portions of the cyst. Distally, the essentially “gonal” locations may be marked by trifurcate projections. Proximal markings between process bases absent or limited to faint parasutural lines; paratabulation standard sexiform gonyaulacacean; archeopyle precingular, Type P, operculum free.
Description.
-
Shape: body subspherical.
-
Wall relationships: autophragm only, or endophragm and periphragm appressed between processes.
-
Wall features: autophragm smooth or faintly ornamented. Parasutural features between process bases faint (e.g., traces of low ridges) or absent altogether; features may be present over entire cyst or only locally.
-
Processes: gonal only or gonal and intergonal; tips of unconnected processes may distally be trifurcate (gonal) or bifurcate (intergonal). A variable portion of the processes distally link to form trabeculae that connect several processes, sometimes leading to a partial and incomplete parasutural network. Connections between processes may comprise one (as in Cannosphaeropsis) or two (as in Nematosphaeropsis) trabeculae.
-
Paratabulation: gonal processes and faint sutural ornamentation indicate standard sexiform gonyaulacacean tabulation.
-
Archeopyle: precingular, Type P (3” only); operculum free.
-
Paracingulum: indicated, if at all, by parasutural lines and position of gonal processes.
-
Parasulcus: indicated, if at all, by parasutural lines.
-
Size: medium.
Type species.
-
Sangiorgia pospelovae sp. nov. (this paper)
Other species.
-
Sangiorgia marretiae sp. nov. (this paper)
Remarks. Sangiorgia is a genus with the basic building plan of Spiniferites and allied genera. Systematically, it resembles Achomosphaera because of the sparse parasutural ornamentation but differs in having distal connections of only a portion of the gonal processes. In Nematosphaeropsis and Cannosphaeropsis, all processes are distally connected (simple, or by “tramlines”) to form a complete network around the central body, whereas only part of the processes are interconnected in Sangiorgia.
-
Sangiorgia pospelovae gen. et sp. nov.
Plates 9, 10, S30, S31, S32, 33
Derivation of name. Named for Vera Pospelova in recognition of her achievements in marine palynology, at the Department of Earth and Environmental Sciences, University of Minnesota, Minneapolis, USA.
Diagnosis. Cysts skolochorate; intermediate to large; body subspherical; bearing isolated essentially gonal; relatively large, solid, processes, of which many, but typically not all, distally interconnect. The distal connections are formed by ribbon-like features, typical for Cannosphaeropsis, sometimes evolved into “tramlines” typical for Nematosphaeropsis. The distally interconnected processes typically form clusters, giving the cysts an irregular outline. Proximal markings between process bases are absent or limited to faint parasutural lines; paratabulation standard sexiform gonyaulacacean; archeopyle precingular, Type P, operculum free.
Holotype. Plate 9, A–D
Paratypes. Plate 9, E–H, I–L; Plate 10, E–H
Material. IODP 302 Hole M0004A, Lomonosov Ridge, Arctic Ocean
Type locality and horizon. IODP 302 Hole M0004A, Lomonosov Ridge, Arctic Ocean, lower Eocene, cores 302-0004A-28X-2-130/132
Age. Early Eocene
Description.
-
Shape: central body subspherical, outline skolochorate, but irregular in consequence of sets of groups of clustered, distally partially connected processes, combined with occasional single, distally not connected gonal processes.
-
Wall relationships: autophragm only, or endophragm and periphragm “appressed” between processes.
-
Wall features: autophragm smooth or faintly ornamented. Parasutural features between process bases faint (e.g., traces of low ridges) or absent altogether; features may be present over entire cyst or only locally.
-
Processes: gonal only, or gonal and intergonal; tips of unconnected processes may distally be broadly trifurcate (gonal) or bifurcate (intergonal). A variable portion (“sets”) of processes distally link to form rather thick trabeculae that connect several processes, sometimes leading to a partial and incomplete distal parasutural network. Connections between (sets of) processes may comprise one (as in Cannosphaeropsis) or two (as in Nematosphaeropsis) trabeculae.
-
Paratabulation: positions of gonal processes and faint sutural ornamentation indicate standard sexiform gonyaulacacean tabulation.
-
Archeopyle: precingular, Type P (3” only); operculum free.
-
Paracingulum: indicated, if at all, by parasutural lines and position of gonal processes.
-
Parasulcus: indicated, if at all, by parasutural lines.
Dimensions. central body 35(40)42 (L) ×30(38)40 (W) (n=10); total dimensions 60(70)77 (L) ×63(68)75 (W)
Holotype. Central body/total: 40/70 µm (L) µm (W).
Paratypes. Central body/total: 35/77 (L) (W) µm, central body/total: 40/70 (L) µm (W) µm, central body/total: 42/68 (L) µm (W) µm
Stratigraphic range/occurrence. Early Eocene
Remarks. Sangiorgia pospelovae somewhat resembles Achomosphaera alcicornu in overall character but has distinctly distally connected (sets of) processes. It differs from S. marretiae in being more robust and larger, having rather thick trabeculae, but foremost in the distinct lack of tri- or bifurcated processes positioned distally on top of the connecting trabeculae, “barbed wire style”.
-
Sangiorgia marretiae sp. nov.
Plates 8, S28, S29
Etymology. Named for Fabienne Marret-Davies in recognition of her achievements in marine palynology, at Liverpool University, United Kingdom.
Diagnosis. Cysts proximochorate; small to intermediate in size, body subspherical, bearing gonal and intergonal, solid processes, of which many, but not all, distally interconnect. The connections are formed by single, ribbon-like trabecular features, which sometimes split into two strands, like the “tramlines” typical for Nematosphaeropsis. The gonal locations are typically distally marked by somewhat broad, trifurcate projections, typical for Spiniferites species. However, since these tri- and occasionally bifurcations occur distally on top of the connecting trabeculae, the overall habitus is also reminiscent of species of Cannosphaeropsis, notably C. utinensis(e.g., “barbed wire style” distal trabeculae). Proximal markings between process bases are limited to faint parasutural lines; paratabulation standard sexiform gonyaulacacean, like all members of the spiniferitioids; archeopyle precingular, Type P, operculum free.
Holotype. Plate 8, E–H
Paratypes. Plate 8, A–D, I–L
Material. IODP 302 Hole M0004A, Lomonosov Ridge, Arctic Ocean
Type locality and horizon. IODP 302 Hole M0004A, Lomonosov Ridge, Arctic Ocean, lower Eocene, Core 302-0004A-27X-3-80/82
Age. Early Eocene
Description.
-
Shape: body subspherical.
-
Wall relationships: autophragm only, or endophragm and periphragm “appressed” between processes.
-
Wall features: autophragm smooth or faintly ornamented. Parasutural features between process bases faint (e.g., traces of low ridges) or absent altogether; features may be present over entire cyst or locally.
-
Processes: gonal only, or gonal and intergonal; tips of connected and unconnected processes are distally trifurcate (gonal) or bifurcate (intergonal) and occur on top of the distally connecting trabeculae. A variable portion of the processes distally links to form trabeculae that connect several processes, sometimes leading to a partial and incomplete parasutural network. Connections between processes may comprise one (as in Cannosphaeropsis) or two (as in Nematosphaeropsis) trabeculae.
-
Paratabulation: positions of gonal processes and faint sutural ornamentation indicates standard sexiform gonyaulacacean (Spiniferitioid) tabulation.
-
Archeopyle: precingular, Type P (3” only); operculum free.
-
Paracingulum: indicated, if at all, by parasutural lines and position of gonal processes.
-
Parasulcus: indicated, if at all, by parasutural lines.
Dimensions. Diameter central body 22(28)35 µm (n=10).
Holotype. 28 µm.
Paratypes. 35 µm, 22 µm.
Stratigraphic range/occurrence. Early Eocene
Remarks. It differs from S. pospelovae in being thin-walled, more fragile, transparent, and smaller and by the presence of distal, tri- or bifurcated processes occurring on top of the distally connecting trabeculae. In overall habitus and variations, this species superficially comes across as a Spiniferites but differs by forming a distinct, but incomplete, distally connecting network of essentially simple (sets of) strands or trabeculae.
-
Subfamily Leptodinioideae
-
Genus Spiniferella (Stover and Hardenbol, 1994)
-
Spiniferella crouchiae sp. nov.
Plates 7, S24
Etymology. Named for Erica Crouch in recognition of her achievements in marine palynology, at GNS Science, Lower Hutt, New Zealand.
Diagnosis. A species of Spiniferella characterized by its large size, with long gonal processes, which terminate in trifurcate ends, that may individually split again into secondary bifurcations or even trifurcations. Proximal markings between process bases are limited to faint parasutural lines; paratabulation standard sexiform gonyaulacacean, like all members of the spiniferitioids; archeopyle precingular, Type P, operculum free.
Holotype. Plate 7, A–B
Paratypes. Plate 7, C and D
Material. IODP 302 Hole M0004A, Lomonosov Ridge, Arctic Ocean
Type locality and horizon. IODP 302 Hole M0004A, Lomonosov Ridge, Arctic Ocean, lower Eocene, Core 302-0004A-27X-3-50/52
Age. Early Eocene
Description.
-
Shape: body subspherical.
-
Wall relationships: autophragm only or endophragm and periphragm appressed between processes.
-
Wall features: autophragm smooth to granulate to reticulate. Prominent parasutural ridges between process bases, like those in the type species Spiniferella cornuta (Stover and Hardenbol, 1994).
-
Processes: gonal only; tips trifurcate, with often secondary bi- and trifurcations.
-
Paratabulation: positions of gonal processes and faint sutural ornamentation indicate standard sexiform, L-type, gonyaulacacean tabulation.
-
Archeopyle: precingular, Type P (3′′ only); operculum free.
-
Paracingulum: indicated by parasutural ridges or septa and the position of gonal processes.
-
Parasulcus: indicated by parasutural ridges or septa.
Dimensions. Total diameter 125(135)150 µm (n=10), central body 70(75)80 µm (n=10).
Holotype. Total 130 µm, central body 80 µm.
Paratypes. 140/65 µm; 130/70 µm.
Stratigraphic range/occurrence. Early Eocene
Remarks. It differs from Spiniferella cornuta by its extraordinary large size and by typical secondary bifurcated or trifurcated endings of the rather long trifurcated gonal spines.
6.2 Photo plates
Codes in the plate captions represent sample codes (core, section, and interval following IODP convention) and England Finder coordinates. The Supplement includes 38 light microscope photoplates and 3 SEM plates, of which high-resolution versions are available on Zenodo (Sluijs and Brinkhuis, 2024, version 4: https://doi.org/10.5281/zenodo.10998747).
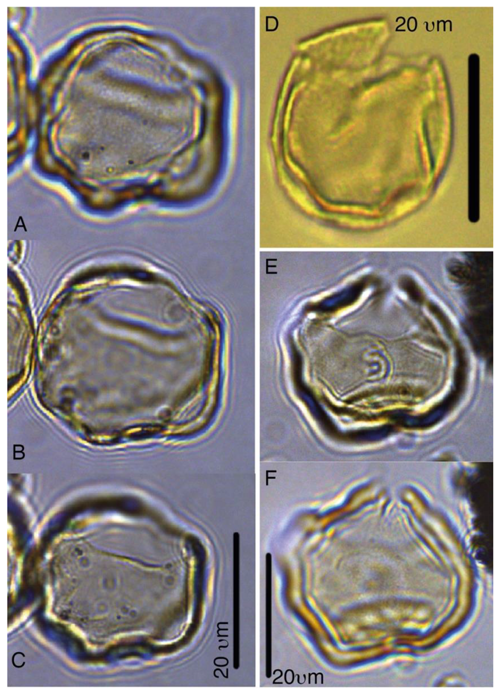
Plate 1Batiacasphaera obohikuenobeae. (a–c) Holotype, 27X-2-03/04 cm, slide 1, R23-1. (d) 27-X-3-30/32 cm, slide 1, G17-4. (e–f) Paratype, 27X-2-03/04 cm, slide 1, P22-3.
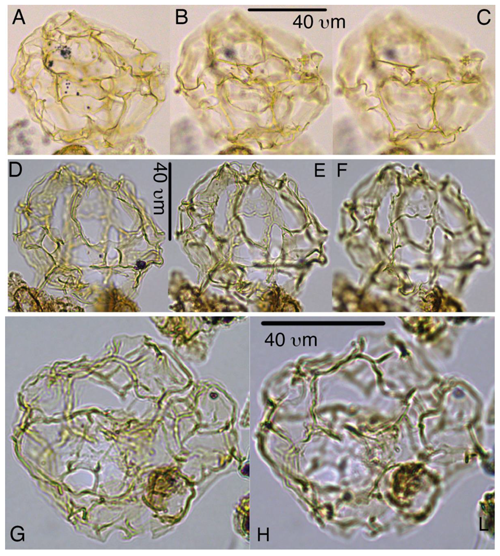
Plate 2Chaenosphaerula sliwinskae. (a–c) Holotype, 27X-2-00/02 cm, slide 1, Q39-2. (d–f) Paratype, 27X-2-14/16 cm, slide 1, O33-3. (g–h) Paratype, 27X-2-20/21 cm, slide 1, E13-4.
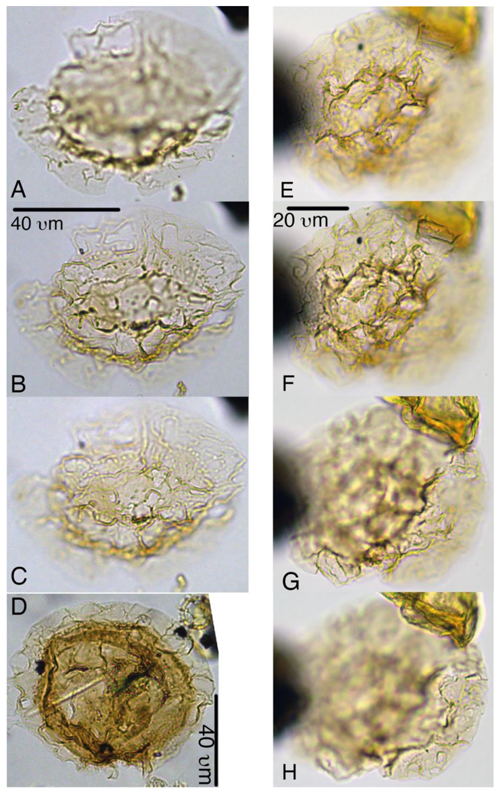
Plate 4Heteraulacacysta pramparoae. (a–c) Paratype, 27X-3-1-110/112 cm, slide 2, H17. (d) 27X-2-20/21 cm, slide 1, B19-4. (e–h) Paratype, 27X-3-90/92 cm, slide 1, D17.
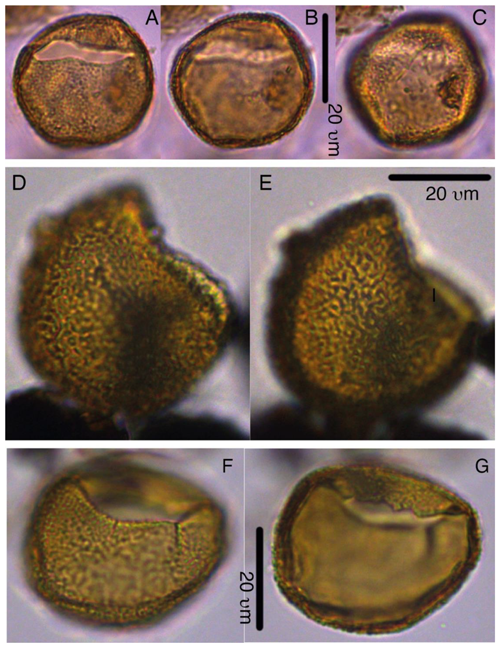
Plate 5Pyxidinopsis iakovlevae. (a–c) Paratype, 27X-1-10/12 cm, slide 2, W30-1. (d–e) Paratype, 33X-CC, slide 1, K13-1. (f–g) Paratype, 33X-CC, slide 1, V22-4.
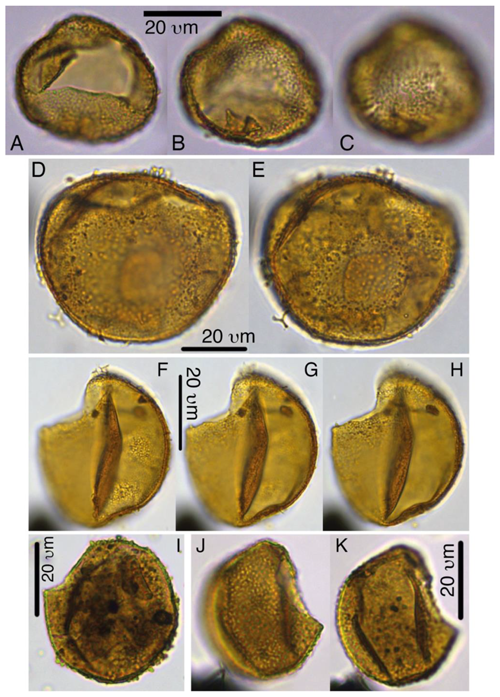
Plate 6Pyxidinopsis iakovlevae. (a–c) Holotype, 27X-3-20/22 cm, slide 1, W14-4. (d–e) Paratype, 27X-1-10/12 cm, slide 2, H27-4. (f–g) Paratype, 27X-3-50/52 cm, slide 2, M20-1. (i) 32X-2-81/83 cm, slide 1, L-41. (j–k) 32X-CC, F29-3.
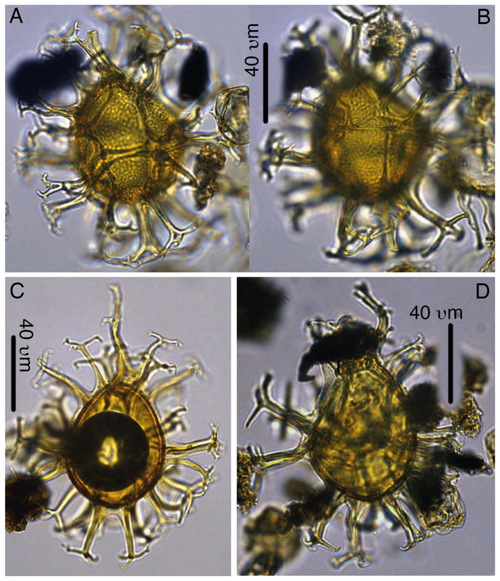
Plate 7Spiniferella crouchiae. (a–b) Holotype, 27X-3-50/52, slide 1, N26-4. (c) Paratype, 27X-2-20/21 cm, slide 1, C19-1. (d) Paratype, 27X-3-30/32, slide 1, K27.
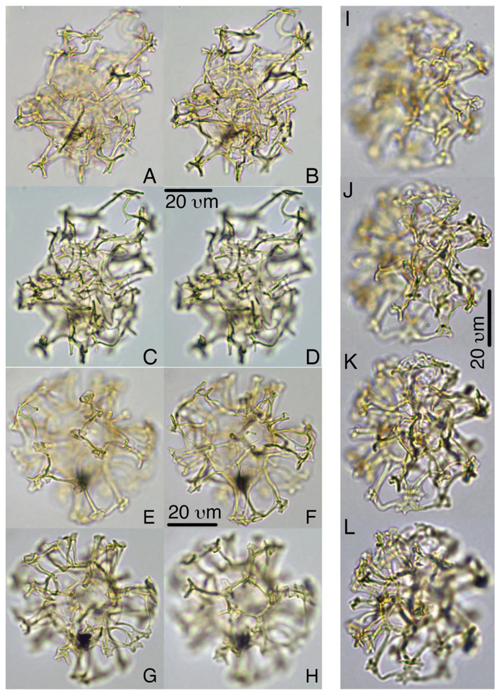
Plate 8Sangiorgia marretiae. (a–d) Paratype, 27X-3-80/82 cm, slide 1, G27-1. (e–h) Holotype, 27X-3-80/82 cm, slide 1, S25-2. (i–l) Paratype, 27X-3-80/82 cm, slide 1, O31-1.
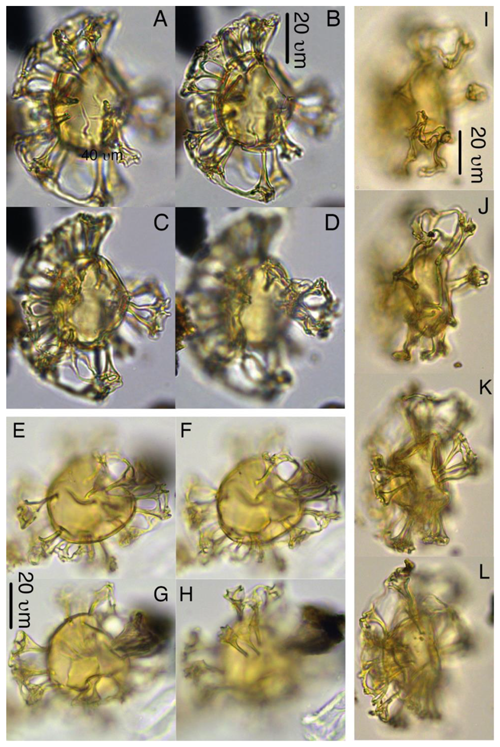
Plate 9Sangiorgia pospelovae. (a–d) Holotype, 28X-2-130/132 cm, slide 1, J27-2. (e–h) Paratype, 28X-40/42, slide 1, U20-4. (i–l) Paratype, 27X-4-10/12 cm, slide 1, L27-4.
6.3 Species list, taxonomic remarks, and taxonomical/ecological grouping
Listed below are all encountered species. Between brackets are the taxonomical/paleoecological groups they have been included in for our quantitative analysis.
-
Achomosphaera alcicornu (Spiniferites cpx)
-
Achomosphaera ramulifera (Spiniferites cpx)
-
Achomosphaera spp. (pars.) (Spiniferites cpx)
-
Adnatosphaeridium spp. (other G-cysts)
-
Alisocysta heilmannii (other G-cysts)
-
Alisocysta circumtabulata (other G-cysts)
-
Alisocysta margarita (other G-cysts)
-
Alisocysta reticulata (other G-cysts)
-
Apectodinium augustum. Remarks: we note that many specimens are somewhat small (Apectodinium spp.).
-
Apectodinium homomorphum (Apectodinium spp.; wetzelielloids)
-
Apectodinium hyperacanthum (Apectodinium spp.; wetzelielloids)
-
Apectodinium parvum (Apectodinium spp.; wetzelielloids). Remarks: some specimens of A. parvum could be confused with Wilsonidium.
-
Apectodinium quinquelatum. Remarks: we note that many specimens are somewhat small. (Apectodinium spp.; wetzelielloids)
-
Apectodinium summissum (Apectodinium spp.; wetzelielloids)
-
Apectodinium spp. (pars; Apectodinium spp.; wetzelielloids).
-
Apteodinium spp. (other G-cysts)
-
Areoligera coronata (Areoligera cpx)
-
Areoligera medusettiformis (Areoligera cpx)
-
Areoligera senonensis (Areoligera cpx)
-
Areoligera spp. (pars) (Areoligera cpx)
-
Batiacasphaera obohikuenobeae sp. nov. (other G-cysts)
-
Batiacasphaera spp. (pars). Remarks: this group includes only specimens with a size like B. compta, thus much larger than B. obohikuenobeae (other G-cysts)
-
Caligodinium aceras (other G-cysts)
-
Cannosphaeropsis spp. (other G-cysts)
-
Cerebrocysta waipawaense (other G-cysts)
-
Cerodinium depressum. Remarks: includes very big specimens (Cerodinium cpx; hexa-2A peridinioids; low-salinity-tolerant)
-
Cerodinium speciosum (Cerodinium cpx; hexa-2A peridinioids; low-salinity-tolerant)
-
Cerodinium striatum Remarks: includes very small specimens (Cerodinium cpx; hexa-2A peridinioids; low-salinity-tolerant)
-
Cerodinium wardenense (Cerodinium cpx; hexa-2A peridinioids; low-salinity-tolerant)
-
Cerodinium spp. (pars.) Remarks: this includes numerous strongly denticulate specimens, reminiscent of the ornamentation of Deflandrea denticulata. (Cerodinium cpx; hexa-2A peridinioids; low-salinity-tolerant)
-
Chaenosphaerula sliwinskae sp. nov. (other G-cysts)
-
Cleistosphaeridium placacanthum (other G-cysts)
-
Cordosphaeridium fibrospinosum (Cordosphaeridium fibrospinosum cpx; other G-cysts)
-
Cordosphaeridium fibrospinosum cpx. Remarks: this includes all specimens within this complex that could not be determined to the genus level. (Cordosphaeridium fibrospinosum cpx; other G-cysts)
-
Cribroperidinium spp.
-
Dapsilidinium spp. (other G-cysts)
-
Deflandrea denticulata Remarks: we have found numerous specimens that are difficult to distinguish from strongly denticulate forms of Cerodinium in this material if the shape and size of the archeopyle cannot be determined (Cerodinium cpx; hexa-2A peridinioids; low-salinity-tolerant)
-
Deflandrea oebisfeldensis (Cerodinium cpx; hexa-2A peridinioids; low-salinity-tolerant)
-
Deflandrea phosphoritica Remarks: we have encountered morphotypes with a “spongy” wall and with striations on its central body. (Cerodinium cpx; hexa-2A peridinioids; low-salinity-tolerant)
-
Deflandrea spp. (pars) (Cerodinium cpx; hexa-2A peridinioids; low-salinity-tolerant)
-
Diphyes colligerum (other G-cysts)
-
Dracodinium pachydermum (wetzelielloids)
-
Elytrocysta spp. Remarks: we have encountered morphotypes ranging from standard transparent to pale yellow to yellow. Moreover, specimens show vague to clear paratabulation reflected in the organization of the processes. Because the ectophragm is not always preserved, specimens might be confused with Histiocysta. (Elytrocysta cpx)
-
Eocladopyxis spp. (other G-cysts)
-
Fibrocysta spp. Remarks: some specimens have very slender processes. (other G-cysts)
-
Glaphyrocysta ordinata (Areoligera cpx)
-
Glaphyrocysta pastielsii (Areoligera cpx)
-
Glaphyrocysta spp. (pars) (Areoligera cpx)
-
Guersteinia delicata gen. et comb. nov. (other G-cysts)
-
Hafniasphaera septata (Spiniferites cpx)
-
Heteraulacacysta pramparoae (epicystal Goniodomidae; other G-cysts)
-
Homotryblium spp. (epicystal Goniodomidae; other G-cysts)
-
Histiocysta spp. (Elytrocysta cpx)
-
Hystrichokolpoma salacia (other G-cysts)
-
Hystrichokolpoma spp. (other G-cysts)
-
Hystrichosphaeridium tubiferum We encountered many specimens of H. tubiferum with a strongly axially elongated central body (see Plates S20, S21), notably in the upper part of the studied interval (Core 27X). Moreover, the shape of the processes is highly variable. We have also encountered specimens in which the processes are distally closed by a membrane, reminiscent of H. truswelliae (other G-cysts).
-
Hystrichostrogylon spp. (Spiniferites cpx; other G-cysts)
-
Impagidinium dispertitum (other G-cysts)
-
Impagidinium spp. (pars.; other G-cysts)
-
Impletosphaeridium spp. (other G-cysts)
-
Kallosphaeridium brevibarbatum (other G-cysts)
-
Lejeunecysta spp. (Protoperidinioid; low-salinity-tolerant)
-
Lentinia serrata (Cerodinium cpx; hexa-2A peridinioids; low-salinity-tolerant)
-
Lentinia wetzelii (Cerodinium cpx; hexa-2A peridinioids; low-salinity-tolerant)
-
Lentinia spp. (pars). Remarks: we note large variability among Lentinia types, including very small, large, striate, psilate, denticulate, and transparent specimens and including intermediates. (Cerodinium cpx; hexa-2A peridinioids; low-salinity-tolerant)
-
Melitasphaeridium pseudorecurvatum (other G-cysts)
-
Microdinium ornatum (Elytrocysta cpx)
-
Minisphaeridium latirictum (other G-cysts)
Muratodinium fimbriatum (Cordosphaeridium fibrospinosum cpx; other G-cysts)
-
Oligosphaeridium spp. (other G-cysts)
-
Operculodinium spp. Remarks: we encountered several morphotypes, dominantly those encountered globally in time-equivalent strata (e.g., Plate 6 C-D of Sluijs and Brinkhuis, 2009), but also large specimens that can be confused with Fibrocysta, specimens with processes resembling those of O. janduchenei, and specimens resembling O. microtrianium (Operculodinium spp.).
-
Palaeocystodinium australinum (Protoperidinioid; low-salinity-tolerant)
-
Palaeocystodinium spp. (pars). Remarks: we encountered some very small specimens (Protoperidinioid; low-salinity-tolerant)
-
Phelodinium magnificum (protoperidinioid; low-salinity-tolerant)
-
Phelodinium spp. (pars.) (protoperidinioid; low-salinity-tolerant)
-
Phthanoperidinium echinatum (Senegalinium cpx; hexa-2A peridinioids; low-salinity-tolerant)
-
Phthanoperidinium spp. (pars.) (Senegalinium cpx; hexa-2A peridinioids; low-salinity-tolerant)
-
Polysphaeridium spp. This includes specimens reminiscent of P. zoharyi. (Epicystal Goniodomidae; other G-cysts)
-
Pyxidinopsis iakovlevae sp. nov. (other G-cysts)
-
Rottnestia borussica (Spiniferites cpx)
-
Sangiorgia pospelovae gen. et sp. nov. (Spiniferites cpx)
-
Sangiorgia marretiae gen. et sp. nov. (Spiniferites cpx)
-
Selenopemphix nephroides (protoperidinioid; low-salinity-tolerant)
-
Selenopemphix selenoides (protoperidinioid; low-salinity-tolerant)
-
Senegalinium obscurum (Senegalinium cpx; hexa-2A peridinioids; low-salinity-tolerant)
-
Senegalinium dilwynense (Senegalinium cpx; hexa-2A peridinioids; low-salinity-tolerant)
-
Senegalinium spp. (pars). Remarks: we encountered a wide variety of morphologies within Senegalinium, including large variations in size, degree of discernible paratabular ridges, subtle to big granulations (sometimes resembling Vozzhennikovia), various types of cavation (notably cornucavate at the apical horn), and specimens with a brown endophragm or entirely brown specimens, resembling Lentinia if the archeopyle could not be discerned. (Senegalinium cpx; hexa-2A peridinioids; low-salinity-tolerant)
-
Spinidinium densispinatum (Senegalinium cpx; hexa-2A peridinioids; low-salinity-tolerant)
-
Spinidinium spp. (pars) (Senegalinium cpx; hexa-2A peridinioids; low-salinity-tolerant)
-
Spiniferella cornuta magna spp. nov. (Spiniferites cpx).
-
Spiniferites ramosus (Spiniferites cpx)
-
Spiniferites pseudofurcatus (Spiniferites cpx)
-
Spiniferites spp. (pars). Remarks: this also includes sometimes very large specimens that show reduced penitabular ridges (towards Achomosphaera). (Spiniferites cpx)
-
Tectatodinium spp. (other G-cysts)
-
Thalassiphora pelagica (Cordosphaeridium fibrospinosum cpx; other G-cysts)
-
Wetzeliella articulata (wetzelielloids)
-
Wetzeliella meckelfeldensis (wetzelielloids)
All raw data are included in a permanent online repository (Sluijs and Brinkhuis, 2024) (https://doi.org/10.5281/zenodo.10998747, version 4). A high-resolution version of the Supplement photoplates (38 plates with light microscope photos and 3 plates with scanning electron microscope photos) is also available under the same Zenodo record.
The supplement related to this article is available online at: https://doi.org/10.5194/jm-43-441-2024-supplement.
AS and HB did all the work and are frankly a bit sad that they finished it.
The contact author has declared that neither of the authors has any competing interests.
Publisher’s note: Copernicus Publications remains neutral with regard to jurisdictional claims made in the text, published maps, institutional affiliations, or any other geographical representation in this paper. While Copernicus Publications makes every effort to include appropriate place names, the final responsibility lies with the authors.
We thank the International Ocean Discovery Program and predecessors for samples and data and the science party of the Arctic Coring Expedition (2004) for their scientific and collaborative efforts. We thank Natasja Welters and Giovanni Dammers (Geolab, Utrecht University) for palynological processing and Gea Zijlstra (Utrecht University) for advice regarding the nomenclature of the newly described taxa. We also thank Ian Harding and an anonymous reviewer for their constructive and detailed analysis of the originally submitted version of this work.
This project was funded by a European Research Council Consolidator Grant (grant no. 771497), awarded to Appy Sluijs under the Horizon 2020 program.
This paper was edited by Luke Mander and reviewed by Ian Harding and one anonymous referee.
Backman, J., Moran, K., McInroy, D. B., Mayer, L. A., and the Expedition 302 Scientists: Proceedings of the Integrated Ocean Drilling Program, 302, Integrated Ocean Drilling Program Management International, Inc., Edinburgh, https://doi.org/10.2204/iodp.proc.302.2006, 2006.
Backman, J., Jakobsson, M., Frank, M., Sangiorgi, F., Brinkhuis, H., Stickley, C., O'Regan, M., Løvlie, R., Pälike, H., Spofforth, D., Gattacecca, J., Moran, K., King, J., and Heil, C.: Age model and core-seismic integration for the Cenozoic Arctic Coring Expedition sediments from the Lomonosov Ridge, Paleoceanography, 23, PA1S03, https://doi.org/10.1029/2007PA001476, 2008.
Barke, J., Abels, H. A., Sangiorgi, F., Greenwood, D. R., Sweet, A. R., Donders, T., Reichart, G.-J., Lotter, A. F., and Brinkhuis, H.: Orbitally forced Azolla blooms and Middle Eocene Arctic hydrology: Clues from palynology, Geology, 39, 427–430, https://doi.org/10.1130/G31640.1, 2011.
Bijl, P. K., Brinkhuis, H., Egger, L. M., Eldrett, J. S., Frieling, J., Grothe, A., Houben, A. J. P., Pross, J., Śliwińska, K. K., and Sluijs, A.: Comment on `Wetzeliella and its allies – the “hole” story: a taxonomic revision of the Paleogene dinoflagellate subfamily Wetzelielloideae' by Williams et al. (2015), Palynology, 41, 423–429, https://doi.org/10.1080/01916122.2016.1235056, 2016.
Bijl, P. K., Frieling, J., Cramwinckel, M. J., Boschman, C., Sluijs, A., and Peterse, F.: Maastrichtian–Rupelian paleoclimates in the southwest Pacific – a critical re-evaluation of biomarker paleothermometry and dinoflagellate cyst paleoecology at Ocean Drilling Program Site 1172, Clim. Past, 17, 2393–2425, https://doi.org/10.5194/cp-17-2393-2021, 2021a.
Bijl, P. K., Guerstein, G. R., Jaimes, E. A. S., Sluijs, A., Casadio, S., Valencia, V., Amenábar, C. R., and Encinas, A.: Campanian-Eocene dinoflagellate cyst biostratigraphy in the southern Andean foreland basin: Implications for drake passage throughflow, Andean Geol., 48, 185–218, https://doi.org/10.5027/andgeov48n2-3339, 2021b.
Brinkhuis, H.: Late Eocene to Early Oligocene dinoflagellate cysts from the Priabonian type-area (Northeast Italy); biostratigraphy and palaeoenvironmental interpretation, Palaeogeogr. Palaeocl., 107, 121–163, 1994.
Brinkhuis, H. and Schiøler, P.: Palynology of the Geulhemmerberg Cretaceous/Tertiary boundary section (Limburg, SE Netherlands), Geologie en Mijnbouw, 75, 193–213, 1996.
Brinkhuis, H., Bujak, J. P., Smit, J., Versteegh, G. J. M., and Visscher, H.: Dinoflagellate-based sea surface temperature reconstructions across the Cretaceous-Tertiary boundary, Palaeogeogr. Palaeocl., 141, 67–83, 1998.
Brinkhuis, H., Sengers, S., Sluijs, A., Warnaar, J., and Williams, G. L.: Latest Cretaceous to earliest Oligocene, and Quaternary dinoflagellate cysts from ODP Site 1172, East Tasman Plateau, in: Proceedings Ocean Drilling Program, Scientific Results, edited by: Exon, N. F., Kennett, J. P., and Malone, M., College Station, Texas, 1–48, https://doi.org/10.2973/odp.proc.sr.189.106.2003, 2003.
Brinkhuis, H., Schouten, S., Collinson, M. E., Sluijs, A., Sinninghe Damsté, J. S., Dickens, G. R., Huber, M., Cronin, T. M., Onodera, J., Takahashi, K., Bujak, J. P., Stein, R., van der Burgh, J., Eldrett, J. S., Harding, I. C., Lotter, A. F., Sangiorgi, F., van Konijnenburg-van Cittert, H., de Leeuw, J. W., Matthiessen, J., Backman, J., Moran, K., and the Expedition 302 Scientists: Episodic fresh surface waters in the Eocene Arctic Ocean, Nature, 441, 606–609, https://doi.org/10.1038/nature04692, 2006.
Bujak, J. P.: V. Dinoflagellate cysts and acritarchs from the Eocene of southern England, in: Bujak, J. P., Downie, C., Eaton, G. L., and Williams, G. L.: Dinoflagellate cysts and acritarchs from the Eocene of southern England, Special Papers in Palaeontology, no. 24, 100 p., pl. 1–22, 1980.
Bujak, J. P. and Brinkhuis, H.: Global warming and dinocyst changes across the Paleocene/Eocene Epoch boundary, in: Late Paleocene – early Eocene climatic and biotic events in the marine and terrestrial records, edited by: Aubry, M.-P., Lucas, S. G., and Berggren, W. A., Columbia University Press, New York, 277–295, ISBN 0-231-190238-0, 1998.
Bujak, J. P. and Mudge, D. C.: A high-resolution North Sea Eocene dinocyst zonation, Journal of the Geological Society, London, 151, 449–462, 1994.
Bujak, J. P., Downie, C., Eaton, G. L., and Williams, G. L.: Dinoflagellate cysts and acritarchs from the Eocene of southern England. Special Papers in Palaeontology, no. 24, 100 p., pl. 1–22, 1980.
Bütschli, O.: Protozoa, in: Klassen und Ordnungen des Thier-Reichs, Wissenschaftlich Dargestellt in Wort und Bild, edited by: Bronn, H. G., Wintersche Verlagsbuchhandling, Leibzig, 865–1088, 1885.
Casas-Gallego, M., Gogin, I., and Vieira, M.: Two new dinoflagellate cyst species and their biostratigraphical application in the Eocene and Oligocene of the North Sea, Palynology, 45, 337–349, https://doi.org/10.1080/01916122.2020.1819457, 2021.
Cookson, I. C. and Eisenack, A.: Some microplankton from the paleocene Rivernook Bed, Victoria, Proc. Roy. Soc. of Victoria, 80, 247–257, 1967.
Cramwinckel, M. J., Huber, M., Kocken, I. J., Agnini, C., Bijl, P. K., Bohaty, S. M., Frieling, J., Goldner, A., Hilgen, F. J., Kip, E. L., Peterse, F., van der Ploeg, R., Röhl, U., Schouten, S., and Sluijs, A.: Synchronous tropical and polar temperature evolution in the Eocene, Nature, 559, 382–386, https://doi.org/10.1038/s41586-018-0272-2, 2018.
Crouch, E. M. and Brinkhuis, H.: Environmental change across the Paleocene-Eocene transition from eastern New Zealand: A marine palynological approach, Mar. Micropaleontol., 56, 138–160, 2005.
Crouch, E. M., Heilmann-Clausen, C., Brinkhuis, H., Morgans, H. E. G., Rogers, K. M., Egger, H., and Schmitz, B.: Global dinoflagellate event associated with the late Paleocene thermal maximum, Geology, 29, 315–318, 2001.
Crouch, E. M., Brinkhuis, H., Visscher, H., Adatte, T., and Bolle, M.-P.: Late Paleocene- early Eocene dinoflagellate cyst records from the Tethys: Further observations on the global distribution of Apectodinium, in: Causes and Consequences of Globally Warm Climates in the Early Paleogene. Geological Society of America Special Paper 369, edited by: Wing, S. L., Gingerich, P. D., Schmitz, B., and Thomas, E., Geological Society of America, Boulder, Colorado, 113–131, 2003.
Crouch, E. M., Shepherd, C. L., Morgans, H. E. G., Naafs, B. D. A., Dallanave, E., Phillips, A., Hollis, C. J., and Pancost, R. D.: Climatic and environmental changes across the early Eocene climatic optimum at mid-Waipara River, Canterbury Basin, New Zealand, Earth-Sci. Rev., 200, 102961, https://doi.org/10.1016/j.earscirev.2019.102961, 2020.
Damassa, S. P.: Dinoflagellate cysts without walls: Evittosphaerula paratabulata Manum, 1979 and Chaenosphaerula magnifica gen. et sp. nov. from Deep Sea Drilling Project Site 338, Norwegian Sea, Rev. Palaeobot. Palyno., 98, 159–176, https://doi.org/10.1016/S0034-6667(97)00020-1, 1997.
Deflandre, G.: Considerations biologiques sur les microorganismes d'origine planctoniques conserves dans les silex de la craie, Bull. biol. France Belgique, 69, 213–244, 1935.
Denison, C. N.: Stratigraphic and sedimentological aspects of the worldwide distribution of Apectodinium in Paleocene/Eocene Thermal Maximum deposits, Geological Society, London, Special Publications, 511, 269–308, https://doi.org/10.1144/SP511-2020-46, 2021.
De Verteuil, L. and Norris, G.: Miocene dinoflagellate stratigraphy and systematics of Maryland and Virginia, Micropaleontology, 42 Supplement, 1–172, 1996.
Drugg, W. S.: Some New Genera, Species, and Combinations of Phytoplankton from the Lower Tertiary of the Gulf Coast, USA, Proceedings of the North American Paleontological Convention, 809–843, 1970.
Drugg, W. S. and Loeblich, A. R. J.: Some Eocene and Oligocene phytoplankton from the Gulf Coast, USA, Tulane Studies in Geology, 5, 181–194, 1967.
Eaton, G. L.: Dinoflagellate cysts from the Bracklesham Beds (Eocene) of the Isle of Wight, southern England, Bull. Brit. Mus (Nat. Hist.) Geol., 26, 227–332, 1976.
Edwards, L. E.: Miocene dinocysts from DSDP Leg 81, Rockall Plateau, eastern North Atlantic Ocean, in: Initial Reports of the Deep Sea Drilling Program 81, Washington, 581–594, https://doi.org/10.2973/dsdp.proc.81.114.1984, 1984.
Edwards, L. E.: Dinoflagellates from the Black Mingo Group (Paleocene) of South Carolina, T. Am. Philos. Soc., 88, 28–48, foi:10.2307/1006669, 1998.
Eisenack, A. and Gocht, H.: Neue Namen für einige Hystrichosphaeren der Bernsteinformation Ostpreussens, Neues Jahrb. Geol. P.-M., 511–581, 1960.
Eldrett, J. S. and Harding, I. C.: Palynological analyses of Eocene to Oligocene sediments from DSDP Site 338, Outer Vøring Plateau, Mar. Micropaleontol., 73, 226–240, https://doi.org/10.1016/j.marmicro.2009.10.004, 2009.
Evitt, W. R.: Sporopollenin dinoflagellate cysts: their morphology and interpretation, American Association of Stratigraphic Palynologists Foundation, Dallas, USA, 1985.
Fauconnier, D. and Masure, E.: Les dinoflagellés fossile. Guide pratique de détermination. Les genres à processus et à archéopyle apical, BRGM Editions, 2004.
Fensome, R. A., Taylor, F. J. R., Norris, G., Sarjeant, W. A. S., Wharton, D. I., and Williams, G. L.: A Classification of Fossil and Living Dinoflagellates, Micropaleontology, Special Publication, 351 pp., 1993.
Fensome, R. A., Williams, G. L., and MacRae, R. A.: The Lentin and Williams Index of Fossil Dinoflagellates, 2019 Edition, AASP Contributions Series Number 50, American Association of Stratigraphic Palynologists Foundation, 2019.
Fokkema, C. D., Agterhuis, T., Gerritsma, D., de Goeij, M., Liu, X., de Regt, P., Rice, A., Vennema, L., Agnini, C., Bijl, P. K., Frieling, J., Huber, M., Peterse, F., and Sluijs, A.: Polar amplification of orbital-scale climate variability in the early Eocene greenhouse world, Clim. Past, 20, 1303–1325, https://doi.org/10.5194/cp-20-1303-2024, 2024a.
Fokkema, C. D., Brinkhuis, H., Peterse, F., and Sluijs, A.: Orbital (Hydro)Climate Variability in the Ice-Free early Eocene Arctic, ESS Open Archive, Paleoceanogr. Paleocl., accepted, https://doi.org/10.22541/essoar.171259268.89696496/v1, 2024b.
Frieling, J. and Sluijs, A.: Towards quantitative environmental reconstructions from ancient non-analogue microfossil assemblages: Ecological preferences of Paleocene – Eocene dinoflagellates, Earth-Sci. Rev., 185, 956–973, https://doi.org/10.1016/j.earscirev.2018.08.014, 2018.
Frieling, J., Iakovleva, A. I., Reichart, G. J., Aleksandrova, G. N., Gnibidenko, Z. N., Schouten, S., and Sluijs, A.: Paleocene-Eocene warming and biotic response in the epicontinental West Siberian Sea, Geology, 42, 767–770, 2014.
Frieling, J., Huurdeman, E. P., Rem, C. C. M., Donders, T. H., Pross, J., Bohaty, S. M., Holdgate, G. R., Gallagher, S. J., McGowran, B., and Bijl, P. K.: Identification of the Paleocene–Eocene boundary in coastal strata in the Otway Basin, Victoria, Australia, J. Micropalaeontol., 37, 317–339, https://doi.org/10.5194/jm-37-317-2018, 2018a.
Frieling, J., Reichart, G.-J., Middelburg, J. J., Röhl, U., Westerhold, T., Bohaty, S. M., and Sluijs, A.: Tropical Atlantic climate and ecosystem regime shifts during the Paleocene–Eocene Thermal Maximum, Clim. Past, 14, 39–55, https://doi.org/10.5194/cp-14-39-2018, 2018b.
Gregory, W. A. and Hart, G. F.: Distribution of dinoflagellates in a subsurface marine Wilcox (Paleocene-eocene) section in southwest Louisiana, Palynology, 19, 45–75, https://doi.org/10.1080/01916122.1995.9989451, 1995.
Habib, D.: Neocomian dinoflagellate zonation in the western North Atlantic, Micropaleontology, 21, 373–392, 1975.
Harding, I. C., Charles, A. J., Marshall, J. E. A., Pälike, H., Roberts, A. P., Wilson, P. A., Jarvis, E., Thorne, R., Morris, E., Moremon, R., Pearce, R. B., and Akbari, S.: Sea-level and salinity fluctuations during the Paleocene-Eocene thermal maximum in Arctic Spitsbergen, Earth Planet. Sc. Lett., 303, 97–107, 2011.
Heilmann-Clausen, C.: Dinoflagellate stratigraphy of the Uppermost Danian to Ypresian in the Viborg 1 borehole, Central Jylland, Denmark, DGU A7, 1–69, https://doi.org/10.34194/seriea.v7.7026, 1985.
Hollis, C. J., Dunkley Jones, T., Anagnostou, E., Bijl, P. K., Cramwinckel, M. J., Cui, Y., Dickens, G. R., Edgar, K. M., Eley, Y., Evans, D., Foster, G. L., Frieling, J., Inglis, G. N., Kennedy, E. M., Kozdon, R., Lauretano, V., Lear, C. H., Littler, K., Lourens, L., Meckler, A. N., Naafs, B. D. A., Pälike, H., Pancost, R. D., Pearson, P. N., Röhl, U., Royer, D. L., Salzmann, U., Schubert, B. A., Seebeck, H., Sluijs, A., Speijer, R. P., Stassen, P., Tierney, J., Tripati, A., Wade, B., Westerhold, T., Witkowski, C., Zachos, J. C., Zhang, Y. G., Huber, M., and Lunt, D. J.: The DeepMIP contribution to PMIP4: methodologies for selection, compilation and analysis of latest Paleocene and early Eocene climate proxy data, incorporating version 0.1 of the DeepMIP database, Geosci. Model Dev., 12, 3149–3206, https://doi.org/10.5194/gmd-12-3149-2019, 2019.
Huber, M. and Caballero, R.: The early Eocene equable climate problem revisited, Clim. Past, 7, 603–633, https://doi.org/10.5194/cp-7-603-2011, 2011.
Iakovleva, A. I. and Heilmann-Clausen, C.: Early and middle Eocene dinoflagellate cysts from the Aktulagay section, Kazakhstan, Palynology, 45, 27–57, https://doi.org/10.1080/01916122.2019.1705933, 2021.
Iakovleva, A. I., Brinkhuis, H., and Cavagnetto, C.: Late Palaeocene-Early Eocene dinoflagellate cysts from the Turgay Strait, Kazakhstan; correlations across ancient seaways, Palaeogeogr. Palaeocl., 172, 243–268, 2001.
Jin, S., Kemp, D. B., Jolley, D. W., Vieira, M., Zachos, J. C., Huang, C., Li, M., and Chen, W.: Large-scale, astronomically paced sediment input to the North Sea Basin during the Paleocene Eocene Thermal Maximum, Earth Planet. Sc. Lett., 579, 117340, https://doi.org/10.1016/j.epsl.2021.117340, 2022.
Jolley, D., Vieira, M., Jin, S., and Kemp, D. B.: Palynofloras, palaeoenvironmental change and the inception of the Paleocene Eocene Thermal Maximum; the record of the Forties Fan, Sele Formation, North Sea Basin, J. Geol. Soc., 180, 2021–2131, https://doi.org/10.1144/jgs2021-131, 2023.
Kender, S., Stephenson, M. H., Riding, J. B., Leng, M. J., Knox, R. W. O. B., Peck, V. L., Kendrick, C. P., Ellis, M. A., Vane, C. H., and Jamieson, R.: Marine and terrestrial environmental changes in NW Europe preceding carbon release at the Paleocene–Eocene transition, Earth Planet. Sc. Lett., 353–354, 108–120, https://doi.org/10.1016/j.epsl.2012.08.011, 2012.
Klement, K. W.: Dinoflagellaten und Hystrichosphaerideen aus dem unteren und mittleren Malm Südwestdeutschlands, Palaeontographica, Abt. A., 114, 1–104, 1960.
Krishnan, S., Pagani, M., Huber, M., and Sluijs, A.: High latitude hydrological changes during the Eocene Thermal Maximum 2, Earth Planet. Sc. Lett., 404, 167–177, 2014.
Lentin, J. K. and Williams, G. L.: Fossil Dinoflagellates: Index to Genera and Species, 1977 Edition, Bedford Institute of Oceanography, Report Series, Bedford Institute of Oceanography, Dartmouth, NS, 209 pp., 1977.
Lentin, J. K. and Williams, G. L.: Fossil Dinoflagellates: Index to genera and species, 1993 Edition, in: AASP Contributions Series, 4, American Association of Stratigraphic Palynologists, 101–134, 1993.
Liengjarern, M., Costa, L., and Downie, C.: Dinoflagellate cysts from the Upper Eocene–Lower Oligocene of the Isle of Wight, Palaeontology, 23, 475–499, 1980.
Lindemann, E.: Abteilung Peridineae (Dinoflagellatae), in: Die Natürlichen Pflanzenfamilien nebst ihren Gattungen und wichtigeren Arten insbesondere den Nutzpflanzen. Zweite stark vermehrte und verbesserte Auflage herausgegeven von A. Engler. 2 Band, edited by: Engler, A. and Prantl, K., Wilhelm Engelmann, Leipzig, Germany, 3–104, 1928.
Manum, S. B.: Two new tertiary dinocyst genera from the norwegian sea: Lophocysta and Evittosphaerula, Rev. Palaeobot. Palyno., 28, 237–248, https://doi.org/10.1016/0034-6667(79)90026-5, 1979.
Marheinecke, U.: Monographie der Dinozysten, Acritarcha und Chlorophyta des Maastrichtium von Hemmoor (Niedersachsen), Palaeontogr. Abt. B, 227, 1–173, 1992.
März, C., Schnetger, B., and Brumsack, H. J.: Paleoenvironmental implications of Cenozoic sediments from the central Arctic Ocean (IODP Expedition 302) using inorganic geochemistry, Paleoceanography, 25, PA3206, https://doi.org/10.1029/2009pa001860, 2010.
März, C., Vogt, C., Schnetger, B., and Brumsack, H.-J.: Variable Eocene-Miocene sedimentation processes and bottom water redox conditions in the Central Arctic Ocean (IODP Expedition 302), Earth Planet. Sc. Lett., 310, 526–537, https://doi.org/10.1016/j.epsl.2011.08.025, 2011.
Morgan, R.: Some Early Cretaceous organic-walled microplankton from the Great Australian Basin, Australia J. and Proc. Roy. Soc. New South Wales, 108, 157–167, 1975.
Morgenroth, P.: Neue in organischer Substanz erhaltene Mikrofossilien des Oligozäns, Neues Jahrb. Geol. P.-A., 127, 1–12, 1966.
Mudie, P. J., Fensome, R. A., Rochon, A., and Bakrač, K.: The dinoflagellate cysts Thalassiphora subreticulata n.sp. and Thalassiphora balcanica: their taxonomy, ontogenetic variation and evolution, Palynology, 44, 237–269, https://doi.org/10.1080/01916122.2019.1567614, 2020.
Müller, R. D., Cannon, J., Qin, X., Watson, R. J., Gurnis, M., Williams, S., Pfaffelmoser, T., Seton, M., Russell, S. H. J., and Zahirovic, S.: GPlates: Building a Virtual Earth Through Deep Time, Geochem. Geophy. Geosy., 19, 2243–2261, https://doi.org/10.1029/2018gc007584, 2018.
Niechwedowicz, M.: Dinoflagellate cysts from the Upper Cretaceous (upper Campanian to lowermost Maastrichtian) of the Middle Vistula River section, Poland, Palynology, 46, 1–37, https://doi.org/10.1080/01916122.2021.1945700, 2022.
Nøhr-Hansen, H., Williams, G. L., and Fensome, R. A.: Biostratigraphic correlation of the western and eastern margins of the Labrador–Baffin Seaway and implications for the regional geology, GEUS Bulletin, 37, 1–74, https://doi.org/10.34194/geusb.v37.4356, 2016.
Norvick, M. S.: The microplankton genus Disphaeria Cookson and Eisenack emend, Bull. Austr. Bur. Min. Res, Geol & Geophys., 140, 45–46, 1973.
Pagani, M., Pedentchouk, N., Huber, M., Sluijs, A., Schouten, S., Brinkhuis, H., Sinninghe Damsté, J. S., Dickens, G. R., and Expedition 302 Scientists, T.: Arctic hydrology during global warming at the Palaeocene-Eocene thermal maximum, Nature, 442, 671–675, 2006.
Pascher, A.: Über Flagellaten und Algen, Deutsche Botanische Gesellachaft, Berichte, 32, 136–160, 1914.
Powell, A. J., Brinkhuis, H., and Bujak, J. P.: Upper Paleocene – Lower Eocene dinoflagellate cyst sequence biostratigraphy of southeast England, in: Correlation of the Early Paleogene in Northwest Europe, Geological Society Special Publication, 101, edited by: Knox, R. W. O. B., Corfield, R. M., and Dunay, R. S., 145–183, 1996.
Prasad, V., Uddandam, P. R., Agrawal, S., Bajpai, S., Singh, I., Mishra, A. K., Sharma, A., Kumar, M., and Verma, P.: Biostratigraphy, palaeoenvironment and sea level changes during pre-collisional (Palaeocene) phase of the Indian plate: palynological evidence from Akli Formation in Giral Lignite Mine, Barmer Basin, Rajasthan, Western India, International Union of Geological Sciences, 43, 476–488, https://doi.org/10.18814/epiiugs/2020/020030, 2020.
Premaor, E., Ferreira, E. P., Guerstein, G. R., and Souza, P. A.: Eocene paleoceanographic and paleoclimatic events recognized by assemblages of dinoflagellate cysts in the Southwest Atlantic Ocean, J. S. Am. Earth Sci., 130, 104587, https://doi.org/10.1016/j.jsames.2023.104587, 2023.
Pross, J. and Brinkhuis, H.: Organic-walled dinoflagellate cysts as paleoenvironmental indicators in the Paleogene; a synopsis of concepts, Palaeont. Z., 79, 53–59, 2005.
Pross, J. and Schmiedl, G.: Early Oligocene dinoflagellate cysts from the Upper Rhine Graben (SW Germany): Paleoenvironmental and paleoclimatic implications, Mar. Micropaleontol., 45, 1–24, 2002.
Prothero, D. R. and Schwab, F.: Sedimentary Geology (2nd Edition), Freeman and Company, New York, 557 pp., ISBN 0716739054, 9780716739050, 2004.
Rush, W., Self-Trail, J., Zhang, Y., Sluijs, A., Brinkhuis, H., Zachos, J., Ogg, J. G., and Robinson, M.: Assessing environmental change associated with early Eocene hyperthermals in the Atlantic Coastal Plain, USA, Clim. Past, 19, 1677–1698, https://doi.org/10.5194/cp-19-1677-2023, 2023.
Sangiorgi, F., van Soelen, E. E., Spofforth, D. J. A., Pälike, H., Stickley, C. E., St. John, K., Koç, N., Schouten, S., Sinninghe Damsté, J. S., and Brinkhuis, H.: Cyclicity in the middle Eocene central Arctic Ocean sediment record: Orbital forcing and environmental response, Paleoceanography, 23, PA1S08, https://doi.org/10.1029/2007PA001487, 2008.
Schiøler, P.: Dinoflagellate cysts and acritarchs from the Oligocene–Lower Miocene interval of the Alma-1X well, Danish North Sea, J. Micropalaeontol., 24, 1–37, https://doi.org/10.1144/jm.24.1.1, 2005.
Schreck, M., Meheust, M., Stein, R., and Matthiessen, J.: Response of marine palynomorphs to Neogene climate cooling in the Iceland Sea (ODP Hole 907A), Mar. Micropaleontol., 101, 49–67, https://doi.org/10.1016/j.marmicro.2013.03.003, 2013.
Seton, M., Müller, R. D., Zahirovic, S., Gaina, C., Torsvik, T., Shephard, G., Talsma, A., Gurnis, M., Turner, M., Maus, S., and Chandler, M.: Global continental and ocean basin reconstructions since 200Ma, Earth-Sci. Rev., 113, 212–270, https://doi.org/10.1016/j.earscirev.2012.03.002, 2012.
Sluijs, A. and Brinkhuis, H.: A dynamic climate and ecosystem state during the Paleocene-Eocene Thermal Maximum: inferences from dinoflagellate cyst assemblages on the New Jersey Shelf, Biogeosciences, 6, 1755–1781, https://doi.org/10.5194/bg-6-1755-2009, 2009.
Sluijs, A. and Brinkhuis, H.: High Arctic late Paleocene and early Eocene dinoflagellate cysts; dinocyst results from IODP Expedition 302 (ACEX), Zenodo [data set], https://doi.org/10.5281/zenodo.10998746, 2024.
Sluijs, A. and Dickens, G. R.: Assessing offsets between the δ13C of sedimentary components and the global exogenic carbon pool across Early Paleogene carbon cycle perturbations, Global Biogeochem. Cy., 26, GB4005, https://doi.org/10.1029/2011GB004224, 2012.
Sluijs, A., Pross, J., and Brinkhuis, H.: From greenhouse to icehouse; organic-walled dinoflagellate cysts as paleoenvironmental indicators in the Paleogene, Earth-Sci. Rev., 68, 281–315, 2005.
Sluijs, A., Schouten, S., Pagani, M., Woltering, M., Brinkhuis, H., Sinninghe Damsté, J. S., Dickens, G. R., Huber, M., Reichart, G.-J., Stein, R., Matthiessen, J., Lourens, L. J., Pedentchouk, N., Backman, J., Moran, K., and the Expedition 302 Scientists: Subtropical Arctic Ocean temperatures during the Palaeocene/Eocene thermal maximum, Nature, 441, 610–613, 2006.
Sluijs, A., Brinkhuis, H., Schouten, S., Bohaty, S. M., John, C. M., Zachos, J. C., Reichart, G.-J., Sinninghe Damsté, J. S., Crouch, E. M., and Dickens, G. R.: Environmental precursors to light carbon input at the Paleocene/Eocene boundary, Nature, 450, 1218–1221, 2007.
Sluijs, A., Brinkhuis, H., Crouch, E. M., John, C. M., Handley, L., Munsterman, D., Bohaty, S., M., Zachos, J. C., Reichart, G.-J., Schouten, S., Pancost, R. D., Sinninghe Damsté, J. S., Welters, N. L. D., Lotter, A. F., and Dickens, G. R.: Eustatic variations during the Paleocene-Eocene greenhouse world, Paleoceanography, 23, PA4216, https://doi.org/10.1029/2008PA001615, 2008a.
Sluijs, A., Röhl, U., Schouten, S., Brumsack, H.-J., Sangiorgi, F., Sinninghe Damsté, J. S., and Brinkhuis, H.: Arctic late Paleocene–early Eocene paleoenvironments with special emphasis on the Paleocene-Eocene thermal maximum (Lomonosov Ridge, Integrated Ocean Drilling Program Expedition 302), Paleoceanography, 23, PA1S11, https://doi.org/10.1029/2007PA001495, 2008b.
Sluijs, A., Schouten, S., Donders, T. H., Schoon, P. L., Röhl, U., Reichart, G. J., Sangiorgi, F., Kim, J.-H., Sinninghe Damsté, J. S., and Brinkhuis, H.: Warm and Wet Conditions in the Arctic Region during Eocene Thermal Maximum 2, Nat. Geosci., 2, 777–780, 2009.
Sluijs, A., Bijl, P. K., Schouten, S., Röhl, U., Reichart, G.-J., and Brinkhuis, H.: Southern ocean warming, sea level and hydrological change during the Paleocene-Eocene thermal maximum, Clim. Past, 7, 47–61, https://doi.org/10.5194/cp-7-47-2011, 2011.
Sluijs, A., Frieling, J., Inglis, G. N., Nierop, K. G. J., Peterse, F., Sangiorgi, F., and Schouten, S.: Late Paleocene–early Eocene Arctic Ocean sea surface temperatures: reassessing biomarker paleothermometry at Lomonosov Ridge, Clim. Past, 16, 2381–2400, https://doi.org/10.5194/cp-16-2381-2020, 2020.
Speelman, E. N., Sewall, J. O., Noone, D., Huber, M., von der Heydt, A., Sinninghe Damsté, J. S., and Reichart, G.-J.: Modeling the influence of a reduced equator-to-pole sea surface temperature gradient on the distribution of water isotopes in the Early/Middle Eocene, Earth Planet. Sc. Lett., 298, 57–65, https://doi.org/10.1016/j.epsl.2010.07.026, 2010.
Steeman, T., De Weirdt, J., Smith, T., De Putter, T., Mees, F., and Louwye, S.: Dinoflagellate cyst biostratigraphy and palaeoecology of the early Paleogene Landana reference section, Cabinda Province, Angola, Palynology, 44, 280–309, https://doi.org/10.1080/01916122.2019.1575091, 2020.
Stein, R., Boucsein, B., and Meyer, H.: Anoxia and high primary production in the Paleogene central Arctic Ocean: First detailed records from Lomonosov Ridge, Geophys. Res. Lett., 33, L18606, https://doi.org/10.1029/2006GL026776, 2006.
Stockmarr, J.: Tablets with spores used in absolute pollen analysis, Pollen et Spores, 13, 615–621, 1972.
Stover, L. E. and Evitt, W. R.: Analyses of Pre-Pleistocene organic-walled Dinoflagellates, Geological Sciences, Stanford University Publications, Stanford, 300 pp., 1978.
Stover, L. E. and Hardenbol, J.: Dinoflagellates and depositional sequences in the Lower Oligocene (Rupelian) Boom clay formation, Belgium, Bulletin van de Belgische Vereniging voor Geologie/Bulletin de la Societe belge de Geologie, T., 102, 5–77, 1994.
Taylor, F. J. R.: On dinoflagellate evolution, BioSystems, 13, 65–108, 1980.
Thöle, L. M., Nooteboom, P. D., Hou, S., Wang, R., Nie, S., Michel, E., Sauermilch, I., Marret, F., Sangiorgi, F., and Bijl, P. K.: An expanded database of Southern Hemisphere surface sediment dinoflagellate cyst assemblages and their oceanographic affinities, J. Micropalaeontol., 42, 35–56, https://doi.org/10.5194/jm-42-35-2023, 2023.
Torsvik, T. H., Van der Voo, R., Preeden, U., Mac Niocaill, C., Steinberger, B., Doubrovine, P. V., van Hinsbergen, D. J. J., Domeier, M., Gaina, C., Tohver, E., Meert, J. G., McCausland, P. J. A., and Cocks, L. R. M.: Phanerozoic polar wander, palaeogeography and dynamics, Earth-Sci. Rev., 114, 325–368, https://doi.org/10.1016/j.earscirev.2012.06.007, 2012.
Vieira, M. and Jolley, D.: Stratigraphic and spatial distribution of palynomorphs in deep-water turbidites: A meta-data study from the UK central North Sea paleogene, Mar. Petrol. Geol., 122, 104638, https://doi.org/10.1016/j.marpetgeo.2020.104638, 2020.
Vieira, M., Mahdi, S., Casas-Gallego, M., and Fenton, J.: Three new Paleocene dinoflagellate cysts from the North Sea and the Norwegian Sea, Rev. Palaeobot. Palyno., 258, 256–264, https://doi.org/10.1016/j.revpalbo.2018.09.002, 2018.
Vieira, M., Mahdi, S., and Holmes, N.: High resolution biostratigraphic zonation for the UK central North Sea Paleocene, Mar. Petro. Geol., 117, 104400, https://doi.org/10.1016/j.marpetgeo.2020.104400, 2020.
Waddell, L. M. and Moore, T. C.: Salinity of the Eocene Arctic Ocean from Oxygen Isotope Analysis of Fish Bone Carbonate, Paleoceanography, 23, PA1S12, https://doi.org/10.1029/2007PA001451, 2008.
Wall, D., Dale, B., Lohmann, G. P., and Smith, W. K.: The environmental and climatic distribution of dinoflagellate cysts in modern marine sediments from regions in the North and South Atlantic Oceans and adjacent seas, Mar. Micropaleontol., 2, 121–200, 1977.
Willard, D. A., Donders, T. H., Reichgelt, T., Greenwood, D. R., Sangiorgi, F., Peterse, F., Nierop, K. G. J., Frieling, J., Schouten, S., and Sluijs, A.: Arctic vegetation, temperature, and hydrology during Early Eocene transient global warming events, Global Planet. Change, 178, 139–152, https://doi.org/10.1016/j.gloplacha.2019.04.012, 2019.
Williams, G. L. and Downie, C.: Further dinoflagellate cysts from the London Clay, in: Studies on Mesozoic and Cainozoic dinoflagellate cysts. Bulletin of the British Museum (Natural History) Geology 3, edited by: Davey, R. J., Downie, C., Sarjeant, W. A. S., and Williams, G. L., 215–248, 1966.
Williams, G. L., Brinkhuis, H., Pearce, M. A., Fensome, R. A., and Weegink, J. W.: Southern Ocean and global dinoflagellate cyst events compared; index events for the Late Cretaceous-Neogene, in: Proceedings Ocean Drilling Program, Scientific Results, 189, edited by: Exon, N. F., Kennett, J. P., and Malone, M. J., College Station, Texas, 1–98, https://doi.org/10.2973/odp.proc.sr.189.107.2004, 2004.
Williams, G. L., Fensome, R. A., and MacRae, R. A.: The Lentin and Williams Index of Fossil Dinoflagellates, 2017 Edition, AASP Contributions Series Number 48, American Association of Stratigraphic Palynologists Foundation, 2017.
Zonneveld, K. A. F., Marret, F., Versteegh, G. J. M., Bogus, K., Bonnet, S., Bouimetarhan, I., Crouch, E., de Vernal, A., Elshanawany, R., Edwards, L., Esper, O., Forke, S., Grøsfjeld, K., Henry, M., Holzwarth, U., Kielt, J.-F., Kim, S.-Y., Ladouceur, S., Ledu, D., Chen, L., Limoges, A., Londeix, L., Lu, S. H., Mahmoud, M. S., Marino, G., Matsouka, K., Matthiessen, J., Mildenhal, D. C., Mudie, P., Neil, H. L., Pospelova, V., Qi, Y., Radi, T., Richerol, T., Rochon, A., Sangiorgi, F., Solignac, S., Turon, J.-L., Verleye, T., Wang, Y., Wang, Z., and Young, M.: Atlas of modern dinoflagellate cyst distribution based on 2405 data points, Rev. Palaeobot. Palyno., 191, 1–197, https://doi.org/10.1016/j.revpalbo.2012.08.003, 2013.