the Creative Commons Attribution 4.0 License.
the Creative Commons Attribution 4.0 License.
A deep-sea agglutinated foraminifer tube constructed with planktonic foraminifer shells of a single species
IODP Expedition 363 Shipboard Scientific Party
Agglutinated foraminifera are marine protists that show apparently complex behaviour in constructing their shells, involving selecting suitable sedimentary grains from their environment, manipulating them in three dimensions, and cementing them precisely into position. Here we illustrate a striking and previously undescribed example of complex organisation in fragments of a tube-like foraminifer (questionably assigned to Rhabdammina) from 1466 m water depth on the northwest Australian margin. The tube is constructed from well-cemented siliciclastic grains which form a matrix into which hundreds of planktonic foraminifer shells are regularly spaced in apparently helical bands. These shells are of a single species, Turborotalita clarkei, which has been selected to the exclusion of all other bioclasts. The majority of shells are set horizontally in the matrix with the umbilical side upward. This mode of construction, as is the case with other agglutinated tests, seems to require either an extraordinarily selective trial-and-error process at the site of cementation or an active sensory and decision-making system within the cell.
- Article
(2929 KB) -
Supplement
(446 KB) - BibTeX
- EndNote
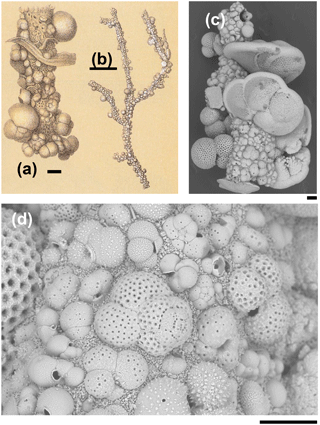
Figure 1Rhizammina algaeformis Brady: (a, b) modified from Brady 1884, pl. 28, fig. 3 (scales approximately 200 µm and 1 mm); (c, d) specimen from IODP Sample U1482B-mudline. Note the large variation in the size, species, and orientation of the planktonic foraminifer shells along the tube, and the siliciclastic matrix. Foraminifera included in the construction include various species of Globigerinita, Globigerinoides, Globorotalia, Globoturborotalita, Tenuitella, Trilobatus, and Turborotalita (scales = 100 µm).
Agglutinated foraminifera are unicellular organisms that construct their shells from sedimentary grains gathered from the sea-floor environment and cement them together to form what are sometimes intricate three-dimensional constructions (Brady, 1879; Gooday, 1990). To do this they must select, orientate and secure the grains in ways that are, at present, poorly understood (Allen et al., 1988; Hemleben and Kaminski, 1990; Makled and Langer, 2010; Rothe et al., 2011). The use of grain type can seem relatively haphazard (Armynot du Châtelet et al., 2013) or it can be highly selective of both size and composition. Examples of selectivity include foraminifera that gather specific minerals, sometimes heavy ones, such as ilmenite (Makled and Langer, 2010), rutile (Cole and Valentine, 2006) and garnet (Allen et al., 1999), or particular biological clast types such as sponge spicules (Brady, 1879), echinoderm plates (Heron-Allen and Earland, 1909), or coccoliths (Holbourn and Kaminski, 1997; Thomsen and Rasmussen, 2008). It is also common for some agglutinated foraminifera to re-use the shells of dead planktonic foraminifera from the surrounding sediment in constructing their tubes (Brady, 1879; Cartwright et al., 1989).
Tube-like agglutinated foraminifera that use planktonic foraminifer shells in their construction are common in bathyal and abyssal environments worldwide, where they live either as suspension or deposit feeders (Gooday, 1990). Fragments are difficult to assign to genus level if the proloculus is absent and the branching pattern unclear, as is the case with the pieces described here. The most important genera that secrete such tubes are Rhabdammina Sars (for thick-walled, inflexible, generally branching tubes), Rhizammina Brady (for thinner-walled, more elastic tubes which form mat-like clumps), Hyperammina Brady (with an enlarged proloculus) and Bathysiphon Sars for slowly expanding tubes. The tubes can include mineral grains and foraminifer shells of various species, sizes and orientations that are attached along their length (Brady, 1879; Gooday, 1983; Cartwright et al., 1989).
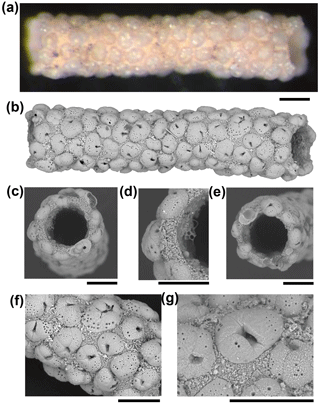
Figure 2A fragment of Rhabdammina sp. that uses planktonic foraminifera of a single species in its construction. (a, b) Light microscope and SEM images of the whole specimen. (c) Broken end of tube. (d) Detail of broken end of tube showing wall in cross section. (e) Other broken end of tube. (f, g) Details of external surface. Specimen from IODP Sample U1482B-mudline (all scales = 100 µm).
Here we illustrate four fragments of what was probably a single tube, which are remarkable because the individual grains belong to a single species of planktonic foraminifer and occur in an organised arrangement. We also show one specimen of Rhizammina from the same sample for comparison. The specimens are from International Ocean Discovery Program (IODP) Site U1482 on the northwest Australian margin at 15∘3.32′ S, 120∘26.10′ E and 1466 m below sea level, from the “mudline”, that is, from unconsolidated seawater and sediment slurry from the top of the first piston core taken from the hole (Sample 363-U1482B-1H-mudline).
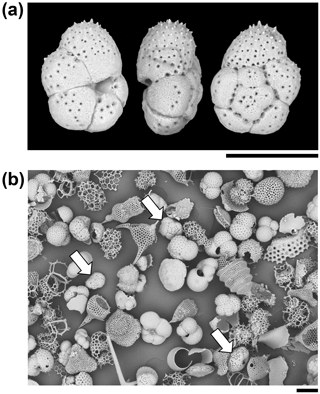
Figure 3Particles from the sediment in IODP Sample U1482B-mudline. (a) Unattached Turborotalita clarkei specimen imaged in umbilical, edge, and spiral views. The umbilical side is distinguished by a deep groove into which the aperture opens. (b) General view of the > 63 µm size fraction showing various species of planktonic foraminifer and radiolarians. Three specimens of T. clarkei are highlighted with arrows. One other species of Turborotalita (T. quinqueloba) and many Tenuitella, Globigerinita, Globoturborotalita, and other genera are present (scales = 100 µm).
The Rhizammina in the sample is of a type commonly encountered in deep-sea sediments, similar to a paratype of Rhizammina algaeformis Brady (Fig. 1). The tube is constructed above an internal organic layer with very fine siliciclastic grains glued together with an organic cement that is not visible in scanning electron microscopy (SEM) but has been described as a probable mucopolysaccharide (Cartwright et al., 1989). The shells of planktonic foraminifera, which have been gathered from the surrounding sediment, are set into this siliciclastic matrix. As is normal in Rhizammina, the planktonic foraminifer tests are of a wide range of sizes and species. The illustrated example contains a variety of adult and juvenile shells belonging to various genera and species, much as occur in the surrounding sediment, although other types of grain such as radiolaria have been avoided. The planktonic foraminifer shells are cemented in apparently random orientations, as has previously been illustrated in this species (Brady, 1879; Cartwright et al., 1989).
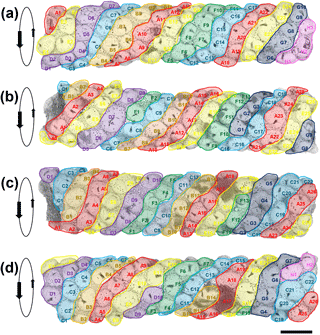
Figure 4Four SEM images of the tube successively rotated forward by approximately 70∘. The apparent sinistral helical arrangement of the planktonic foraminifer shells is highlighted by colour coding in bands A–H. Each specimen is given a unique identifier according to its position on a band. Orientation data for each specimen are available in Table S1 in the Supplement (scale = 100 µm).
The four fragments of ?Rhabdammina (illustrated as Fig. S1 in the Supplement) are all broken at both ends. The largest fragment is a very regular tube, nearly perfectly circular in internal cross section and about 1 mm long with a fairly constant external diameter of about 250 µm and an internal diameter of 150 µm (Fig. 2). Although polychaetes, nematodes, and crustaceans are all known to construct agglutinated tubes (Gooday, 1990; Finger et al., 2008), the specimens described here can be securely identified as a foraminifer because of the small size and mode of construction which is similar to other agglutinated foraminifera including Rhizammina from the same sample. As in Rhizammina (see Fig. 1), the tube has a matrix of angular siliciclastic grains into which planktonic foraminifer shells are set.
A total of 123 planktonic foraminifer shells are cemented along the tube of the largest fragment, all of which belong to the single species, Turborotalita clarkei (Rögl and Bolli, 1973) (as do the > 100 shells in the smaller fragments). This species is one of the smallest living planktonic foraminifera, spinose in life, with a cosmopolitan distribution (Hemleben et al., 1989). All specimens on the tube appear to be adult, with a diameter of about 100 µm and a thick external cortex, which is not always the case in the sediment. The sediment itself contains over 20 species of planktonic foraminifer, among which T. clarkei is a relatively frequent but not dominant component among its size class, which also includes abundant radiolarians (Fig. 3). Hence the exclusive use of T. clarkei in shell construction appears highly selective. It may have been preferred because of its relatively high density, small size and simple, elliptical outline.
The construction of the matrix also implies considerable selectivity because detrital grains are a relatively minor component of the very fine fraction sediments recovered at this location, compared to biogenic grains such as coccoliths, which are entirely excluded (although they can be seen adhering to the specimen in places, which was only gently cleaned). The binding cement appears to be very strong because planktonic foraminifer shells at both broken ends of the tube are fractured through the middle rather than having been detached at their edges (see Fig. 2c, e).
The most notable feature of the specimen is the regular way in which T. clarkei shells are attached along the outside of the tube, which contrasts strongly with the more haphazard pattern seen in Rhizammina (see Fig. 1). The arrangement appears to be roughly helical, because it is possible to trace a series of sinistrally coiled helical bands along all or most of the length of the tube (Fig. 4). The T. clarkei shells are regularly spaced and oriented, with the great majority having their equatorial peripheries set into the matrix rather than being edge-on or at an oblique angle as is frequently seen in Rhizammina (see Fig. 1d). The T. clarkei shells generally just touch the inner surface but project above the outer surface, giving the tube a beaded appearance. For the larger, more oval specimens, the long axis is generally along the line of the helix. Most remarkable of all, the majority of shells are umbilical side up, with the umbilical groove in which the aperture is set visible on the outside (104 specimens are umbilical side up versus 14 that are spiral side up and 5 which are on their side). The umbilical-side-up orientation may have been preferred because it avoids having the umbilical groove on the inside, making it easier to construct a smooth inner surface. (The three smaller fragments of tube are also exclusively made of T. clarkei with a strong preference for umbilical side upward orientation although the helical pattern is less clear because of the narrower tube diameters; see Supplement.)
Although to our knowledge Rhabdammina has not been observed alive, it presumably locates and moves sedimentary grains with its pseudopodial network similar to many other foraminifera. The high degree of grain-type selectivity exhibited by this and other agglutinated foraminifera must be time- and energy-expensive. Once in position at the growing tip of the tube, the grains are presumably cemented in place with an admixture of fine siliciclastic material and secreted polysaccharide cement. The helical arrangement may reflect the growth sequence, in which generally five or six T. clarkei shells were located around the growing tip (or fewer when the diameter was narrower), with each new shell being cemented to the left (looking from the outside) of a pre-existing one. Clues to the construction mode come from instances where unusually large or small shells were included, for which there may have been subsequent compensation (e.g. the large specimen D7 in Fig. 4b may have been compensated for by the smaller specimens C8 and E1, moving left to right, and causing a new helical band to start).
The apparently complex behaviour of agglutinated foraminifera has attracted attention since it was discovered in the 1860s and described by W. B. Carpenter (Carpenter, 1873, p. 784) as “most distinct evidence of selective power; and the question forces itself upon us, – by what instrumentality is it exercised” (italics and punctuation as in original). Charles Darwin wrote to Carpenter describing it as “almost the most wonderful fact I ever heard of. One cannot believe that they have mental power enough to do so, and how any structure or kind of viscidity can lead to this result passes all understanding” (Charles Darwin letter to W. B. Carpenter, 1873, transcribed in Burkhardt et al., 2014, item DAR 261.6:7). The issue was debated again in 1916, when the foraminifer specialist Edward Heron-Allen claimed it as an example of “intelligence”, which was robustly disputed by the physiologist Edward Ray Lankester in an exchange that quickly became a semantic argument (as described by Hemleben and Kaminski, 1990). But while terms such as “mental power” and “intelligence” remain jarring, the basic problem of how a single cell effects this kind of behaviour remains almost entirely unresolved and the debate has hardly moved on (Allen et al., 1988; Hemleben and Kaminski, 1990; Makled and Langer, 2010; Rothe et al., 2011).
The phenomenon seems to belong to a different category from cases in which unicellular organisms such as other foraminifera, radiolaria, and diatoms secrete intricate skeletons of silica or carbonate as part of their life cycle (see, for instance, Fig. 3). In those cases the biomineralisation sequence is presumably under genetic and epigenetic control in which information is stored in genes which switch on or off in response to internal triggers. In the case of complex agglutinating behaviour, the correct grain type must be discovered by the pseudopodial network, transported to the precise location required, and then manipulated in three dimensions before being cemented into place. At some point in the process it must be discriminated from other similar grains. We suggest two possible models for how this discrimination occurs. Either (1) it occurs via a highly specific trial-and-error process at the site of cementation to which many grains are brought and rejected, and only T. clarkei grains in the correct orientation are accepted, presumably because of having the correct affinity to the binding site, or (2) it occurs in the pseudopodial network wherein only the correct grain type is selected from the sediment and is then moved into position. Both these mechanisms are difficult to envisage, however. The former would be extremely costly and time-consuming whereas the latter demands a sensory system capable of continuously monitoring grain position and orientation plus an information processing ability with decision-making capacity. If such behaviour was exhibited by a metazoan, it would naturally be attributed to the functioning of the nervous system. Observation of living agglutinated foraminifera may help distinguish between these models.
Much behaviour in protists is apparently highly stereotyped and involves chemosensory responses to environmental cues in relation to feeding, predator avoidance, and discriminating clones and potential mates (Vandromme et al., 2010; Harvey et al., 2013). However recent work on partner recognition in some ciliates has concluded that they are able to actively encode, process and respond to information from external pheromone signals which in turn produces planned “courtship strategies” and “social decision making” (Clark, 2013). In the agglutinated foraminifer cell discussed here, the decision-making must also, presumably, have a specialised molecular basis, whether it occurs at the site of cementation or distributed in the pseudopodial network. As far as we know, such processes are obscure, but if they can occur in one type of cell, they could occur in others, so the phenomenon could be more than just a curiosity.
Seawater and sediment slurry from the top of the first piston core at IODP Hole U1482B was collected in a bucket, treated with rose bengal biological stain for 24 h to detect living cells and washed over 150 and 63 µm sieves. The sample residues were dried on a warm plate at 40 ∘C. Foraminifer specimens including shells of Turborotalita clarkei and fragments of Rhabdammina sp. and Rhizammina algaeformis were picked by brush and transferred to conductive adhesive discs on metal pedestals for microscopic investigation. Specimens were photographed using a Zeiss Discovery V8 light microscope (LM) and a Hitachi TM 3000 tabletop scanning electron microscope (SEM) in various orientations without conductive coating.
The data to this paper can be found in the Supplement.
The supplement related to this article is available online at: https://doi.org/10.5194/jm-37-97-2018-supplement.
Ivano W. Aiello (Geological Oceanography, Moss Landing Marine Laboratories, 8272 Moss Landing Road, Moss Landing, CA 95039-9647, USA), Tali L. Babila (Ocean Sciences Department, University of California, Santa Cruz, 1156 High Street, Santa Cruz, CA 95064, USA), Germain Bayon (Unité Géosciences Marines, Institut Francaise de Recherche pour l'Exploitation de la Mer (IFREMER), 29280 Plouzané, France), Luc Beaufort (Centre Europén de Recherche et d'Enseignement de Géologie de l'Environnement (CEREGE) BP80, Centre National de la Recherche Scientifique (CNRS), Aix-Marseille Université, Ave L. Philbert, 13545 Aix-en-Provence, France), Samantha C. Bova (Institute of Marine and Coastal Sciences, Rutgers, The State University of New Jersey, 71 Dudley Road, New Brunswick, NJ 08901-8521, USA), Jong-Hwa Chun (Petroleum and Marine Research Division, Korea Institute of Geoscience and Mineral Resources (KIGAM), 124 Gwahnag-no, Yuseong-gu, Daejeon 305-350, Korea), Haowen Dang (State Key Laboratory of Marine Geology, Tongji University, 1239 Siping Road, Shanghai 200092, P.R. China), Anna Joy Drury (MARUM, University of Bremen, Leobener Str., 28359 Bremen, Germany), Tom Dunkley Jones (School of Geography, Earth and Environmental Sciences, University of Birmingham, Edgbaston, Birmingham B15 2TT, UK), Patrícia P. B. Eichler (Department of Geophysics, Geodynamics and Geology, Federal University of Rio Grande do Norte, Campus Universitário, Lagoa Nova, Natal RN 59078-970, Brazil), Fernando Allan Gil Salazar (National Institute of Geological Sciences, University of the Philippines, Diliman, Quezon City 1101, Philippines), Kelly Gibson (SEOE, University of South Carolina, 701 Sumter Street, EWS 617, Columbia, SC 29208, USA), Robert G. Hatfield (College of Earth, Ocean and Atmospheric Sciences, Oregon State University, 104 CEOAS Admin. Building, Corvallis, OR 97331, USA), Ann E. Holbourn (Institute of Geosciences, Christian-Albrechts-Universität zu Kiel, Ludewig-Meynstr. 10–14, 24118 Kiel, Schleswig Holstein, Germany), Daniel L. Johnson (Department of Geological and Planetary Sciences, California Institute of Technology, 1200 E. California Boulevard, Pasadena, CA 91125, USA), Denise K. Kulhanek (International Ocean Discovery Program, Texas A&M University, 1000 Discovery Drive, College Station, TX 77845, USA), Yuho Kumagai (Department of Earth Science, Tohoku University, Aoba 6-3, Sendai 980-8578, Japan), Tiegang Li (The First Institute of Oceanography, SOA, 7 Nanhai Road, 6 Xian Xialing Rd. 266061, P.R. China), Braddock K. Linsley (Lamont-Doherty Earth Observatory, Columbia University Geoscience 104, 61 Route 9W, Palisades, NY 10964, USA), Niklas Meinicke (Department of Earth Science, University of Bergen, Norway, Allegaten 41, 5007 Bergen, Norway), Gregory S. Mountain (Department of Earth and Planetary Sciences, Rutgers, The State University of New Jersey, 610 Taylor Road, Piscataway, NJ 08854-8066, USA), Bradley N. Opdyke (Research School of Earth Sciences, Australian National University, Canberra ACT 0200, Australia), Christopher R. Poole (Department of Earth Sciences, University College London, Gower Street, London WC1E 6BT, UK), Christina Ravelo (Ocean Sciences Department, University of California, Santa Cruz, 1156 High Street, Santa Cruz, CA 95064, USA), Yair Rosenthal (Institute of Marine and Coastal Sciences, Rutgers, The State University of New Jersey, 71 Dudley Road, New Brunswick, NJ 08901-8521, USA), Takuya Sagawa (Institute of Science and Engineering, Kanazawa University, Kakuma-machi, Kanazawa 920-1192, Japan), Anaïs Schmitt (Faculty of Science and Technology, Université de Nantes, 2 rue de la Houssinière, 44322 Nantes, France), Jennifer B. Wurtzel (Research School of Earth Sciences, Australian National University, Canberra ACT 0200, Australia), Jian Xu (Department of Geology, Northwest University, 229 North Taibai Road, Xi'an Shaanxi 710069, P.R. China), Masanobu Yamamoto (Faculty of Environmental Earth Science, Hokkaido University, Kita-10, Nishi-5, Kita-ku, Sapporo 060-0810, Japan), and Yi Ge Zhang (Texas A&M University, Department of Oceanography, MS 3146, College Station, TX 77843, USA).
The authors declare that they have no conflict of interest.
We thank Bill Crawford for ship-board photography, Wolfgang Kuhnt for advice,
and Trevor Dale for stimulating discussion.
Edited by: Laia Alegret
Reviewed by: Andrew Gooday and one
anonymous referee
Allen, K., Roberts, S., and Murray, J. W.: Fractal grain distribution in agglutinated foraminifera, Paleobiology, 24, 349–358, 1988.
Allen, K., Roberts, S., and Murray, J. W.: Marginal marine agglutinated Foraminifera: Affinities for mineral phases, J. Micropalaeontology, 18, 183–191, 1999.
Armynot du Châtelet, E., Frontalini, F., Guillot, F., Recourt, P., and Ventalon, S.: Surface analysis of agglutinated benthic foraminifera through ESEM-EDS and Raman analysis: An expeditious approach for tracing mineral diversity, Mar. Micropaleontol., 105, 18–29, 2013.
Brady, H. B.: Notes on some of the reticularian Rhizopoda of the “Challenger” expedition. 1. On new or little known arenaceous types, Q. J. Microsc. Sci., New Series, 19, 20–63, 1879.
Burkhardt, F., Secord, J. A., Bowne, J., Evans, S., Innes, S., Neary, F., Pearn, A. M., Secord, A., and White, P. (Eds.): The Correspondence of Charles Darwin: Volume 21, 1873, Cambridge University Press, 274 pp., 2014.
Carpenter, W. B.: On the hereditary transmission of acquired psychical habits, The Contemporary Review, 21, 779–795, 1873.
Cartwright, N. G., Gooday, A. J., and Jones, A. R.: The morphology, internal organization, and taxonomic position of Rhizammina algaeformis Brady, a large, agglutinated, deep-sea foraminifer, J. Foramin. Res., 19, 115–125, 1989.
Clark, K. B.: Ciliates learn to diagnose and correct classical error syndromes in mating strategies, Frontiers in Microbiology, 4, 1–22, 2013.
Cole, K. E. and Valentine, A. M.: Titanium biomaterial: Titania needles in the test of the foraminiferan Bathysiphon argenteus, Dalton T., 3, 430–432, 2006.
Finger, K. L., Flenniken, M. M., and Lipps, J. E.: Foraminifera used in the construction of Miocene polychaete worm tubes, Monterey Formation, California, USA, J. Foramin. Res., 38, 277–291, 2008.
Gooday, A. J.: Bathysiphon rusticus de Folin, 1886 and Bathysiphon folinin. sp.; two large agglutinated foraminifers abundant in abyssal NE Atlantic epibenthic sledge samples, J. Foramin. Res., 13, 262–276, 1983.
Gooday, A. J.: Recent deep-sea agglutinated foraminifera: a brief review, in: Paleoecology, Biostratigraphy, Paleoceanography and Taxonomy of Agglutinated Foraminifera, edited by: Hemleben, C., Kaminski, M. A., Kuhnt, W., and Scott, D. B., Kluwer, Dordrecht, 271–304, 1990.
Harvey, E. L., Jeong, H. J., and Menden-Deuer, S.: Avoidance and attraction: chemical cues influence predator-prey interactions of planktonic protists, Limnol. Oceanogr., 58, 1176–1184, 2013.
Hemleben, C. and Kaminiski, M.: Agglutinated foraminifera: an introduction, in: Paleoecology, Biostratigraphy, Paleoceanography and Taxonomy of Agglutinated Foraminifera, Hemleben, C., Kaminski, M. A., Kuhnt, W., and Scott, D. B., Kluwer, Dordrecht, 3–11, 1990.
Hembleben, C., Spindler, M., and Anderson, O. R.: Modern Planktonic Foraminifera, Springer-Verlag, New York, 363 pp., 1989.
Heron-Allen, E. and Earland, A.: On a new species of Technitella from the North Sea, with observations on selective power as exercised by certain species of arenaceous foraminifera, Journal of the Queckett Microscopical Club, 10, 403–412, 1909.
Holbourn, A. and Kaminski, M.: Lower Cretaceous deep-water benthic foraminifera of the Indian Ocean, Grzybowski Foundation Special Publication, 4, 172 pp., 1997.
Makled, W. A. and Langer, M. R.: Preferential selection of titanium-bearing minerals in agglutinated foraminifera: Ilmenite (FeTiO3) in Textulatia hauerii d'Orbigny from the Bazaruto Archipelago, Mozambique, Revue de Micropaléontologie, 53, 163–173, 2010.
Rögl, F. and Bolli, H. M.: Holocene to Pleistocene planktonic foraminifera of Leg 15, Site 147 (Cariaco Basin (Trench), Caribbean Sea) and their climatic interpretation, in: Initial Reports of the Deep Sea Drilling Project, edited by: Edgar, N. T., Kaneps, A. G., and Herring, J. R., US Government Printing Office, Washington DC, 15, 553–615, 1973.
Rothe, N., Gooday, A. J., and Pearce, R. B.: Intracellular mineral grains in the xenophyophore Nazareammina tenera (Rhizaria, Foraminifera) from the Nazare Canyon (Portuguese margin, NE Atlantic), Deep-Sea Res., 58, 1189–1195, 2011.
Thomsen, E. and Rasmussen, T. L.: Coccolith-agglutinating Foraminifera from the early Cretaceous and how they construct their tests, J. Foramin. Res., 38, 193–214, 2008.
Vandromme, P., Schmidtt, F., Souissi, S., Buskey, E. J., Strickler, J. R., Wu, C.-H., and Hwang, J.-S.: Symbolic analysis of plankton swimming trajectories: case study of Strobilidium sp. (Protista) helical walking under various food conditions, Zool. Stud., 49, 289–303, 2010.