the Creative Commons Attribution 4.0 License.
the Creative Commons Attribution 4.0 License.
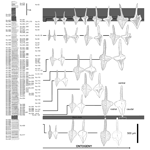
Anagenetic evolution and peramorphosis of a latest Devonian conodont from Holy Cross Mountain (Poland)
Przemysław Świś
I explored the fossil record of the Dasbergina marburgensis → Dasbergina trigonica lineage in Kowala, situated in the Holy Cross Mountains of central Poland. Through biometrical measurements of the platform P1 element, I traced the trajectory of anagenetic evolution. The collected data reveal a gradual shift in the morphology of elements, encompassing the development of branches, a change in the platform line, and transformations of the basal cavity. An interesting aspect lies in the ontogeny evolution, which I studied using rhythmic increments corresponding to potential days of the animals lifespan. Notably, the organogenesis of branches, calibrated based on ontogeny, indicates that these conodonts underwent a process of peramorphosis. Furthermore, this study introduces an alternative approach for age correlation during the latest Famennian period and perspectives on the evolutionary history of Dasbergina.
- Article
(8305 KB) - Full-text XML
- BibTeX
- EndNote
Conodonts are an extinct group of small, lamprey-shaped organisms that inhabited the seas from the Late Cambrian to the Late Triassic period (Rigo et al., 2018) or even the earliest Jurassic (Du et al., 2020, 2023). They hold a significant place in the study of vertebrate evolution, paleoecology, and biostratigraphy (e.g. Leonhard et al., 2021; Martínez-Pérez et al., 2016; Shohel et al., 2022; Sandberg and Ziegler, 1979). Characterized by their unique tooth-like structures, called conodont elements, they can be compared in terms of complexity to mammalian teeth (Donoghue and Purnell, 1999; Assemat et al., 2023). These elements were arranged in a sophisticated oral apparatus usually comprising 15 elements. As conodont elements developed, they expanded by outward deposition by adding subsequent increments (Dzik, 2000; Donoghue, 1998). The mode of growth and inner structure resembles that of teeth, where the crown and basal tissues bear a similarity to enamel and dentine (Schmidt and Müller, 1964; Dzik, 1986; Smith et al., 1996; Donoghue et al., 2000; McCollum and Sharpe, 2001). Each increment becomes visible either in cross section or on the internal surface of the basal cavity of each element, provided there is no basal filling on its aboral side (Müller and Nogami, 1971; Dzik, 2000; Donoghue, 1998). The conodont incremental layers demonstrate a consistent and uniform thickness, hinting at their likely secretion at regular intervals. This regular pattern of deposition suggests the possibility of alignment with daily (Armstrong and Smith, 2001; Dzik, 2008, 2015; Świś, 2019) and/or seasonal rhythms (Shunxin et al., 1997). Whatever cyclicity they exhibit, this regular deposition pattern offers the possibility of determining the relative ontogenetic age of individuals and enables a precise morphological comparison between individuals of the same ontogenetic age. The development of cusps, denticles, or processes is reflected within the aforementioned increments. This characteristic pattern permits calibration of their morphogenesis throughout ontogeny (Müller and Nogami, 1971; Mazza and Martínez-Pérez, 2016; Shirley et al., 2018).
Several studies dealing with conodonts used the most durable and variable last pair of pharyngeal elements – P1. These organisms were the object of interest for researchers due to their exceptional position in the evolutionary history of vertebrates (Donoghue and Keating, 2014; Dzik, 2000; Donoghue et al., 2000; Donoghue, 1998), their role as paleoenvironment indicators (Joachimski and Buggisch, 2002; Trotter et al., 2008; Girard et al., 2020), their potential role in ancient trophic networks (Terrill et al., 2022; Ginot and Goudemand, 2019; Zhuravlev, 2020; Kelz et al., 2023), and their value in petroleum exploration (Epstein et al., 1977). Conodont elements were among the oldest mineralized skeletons secreted by any vertebrate, setting them as a remarkable tool to understand the early skeletal evolution (Murdock et al., 2013). Moreover, the intricate variations in conodont species and their elements across different geological formations provide an invaluable biostratigraphical tool for dating and correlating rocks (e.g. Ziegler and Sandberg, 1984; Sandberg and Ziegler, 1996; Spalletta et al., 2017). These elements, often preserved as fossils within carbonate rocks, represent valuable information about ecological interactions within deep-time ecosystems (Zaton et al., 2017; Jarochowska et al., 2017, 2021; Świś, 2019).
However, conodont studies were made difficult by uncertainties about their biological origins and affinities, multielementary structure of apparatus, and different naming conventions (review in Sweet and Donoghue, 2001). The first attempt to produce a comprehensive classification scheme for biostratigraphic purposes was in the 1920s (Ulrich and Bassler, 1926); however, it was based upon the incorrect hypothesis that elements were not part of an oral apparatus. Later on, the discovery of an articulated apparatus (Scott, 1934; Schmidt, 1934) led to a boost of the taxonomy in 1960s and 1970s with a multielemental apparatus approach (e.g. Lindström, 1970; Jeppsson, 1969; Klapper and Philip, 1971). Investigations of the interrelationships amongst conodonts were not an easy task due to the high rate of convergences of certain structures in conodont elements (Donoghue, 2001). Recently, the majority of the authors have focused on selected genera, instead of reconstructing the whole conodont evolution (Dzik, 2015; Świś, 2022; Guenser et al., 2021; Renaud et al., 2021; Girard et al., 2022; Petryshen et al., 2020; Souquet et al., 2022; Kelz et al., 2023). A promising method for studying the evolution in the fossil record is tracing morphological changes by biometrical analysis. Through biometrics we can retrace interspecific variations in the fossil species in subsequent populations. If there was no genetic isolation, a given species might undergo anageny that is a gradual transformation in new morphological features (e.g. Aze et al., 2011; Czepiński, 2020; Olempska, 1989; Carr et al., 2017). The continuous sequences of species that underwent anagenesy are chronospecies (Dzik, 1985, 2005). The investigation of morphology in dense sampling to describe evolution processes and establish relationships between taxa is referred to stratophenetic (Gingerich, 1979). The results were shown for mammals (e.g. Rose and Bown, 1986; Krishtalka, 1993; Silcox, 2014; Silcox et al., 2021), conodonts (e.g. Dzik, 1985, 1987), foraminifera (e.g. Wei, 1994; Georgescu et al., 2008; Pearson and Coxall, 2014), and ostracods (Olempska, 1989).
The objective of this article is to reconstruct anagenesis using a stratophenetic approach within the conodont lineage D. marburgensis – D. trigonica (Dzik, 2006; Dzik et al., 2022) by using morphometric methods applied to the rich collections from Kowala in the Holy Cross Mountains (central Poland). During the study, emphasis will be given to the character and trajectory of anagenetic morphological changes in individuals development. This could help detect heterochrony, which corresponds to changes in the timing of development events or processes during an organism's evolution. Such a pattern was already identified in conodonts (Renaud and Girard, 1999; Broadhead and MeComb, 1983) and related to a temperature control (Souquet et al., 2022). In this study, I will use the general shape of the conodont elements along with growth increments in order to retrace the process of development of new branches (new functional surfaces in the elements, independent from the main denticles row). Such structures will be described using scanning electron microscope (SEM) images of the basal cavity. This approach will help establish evolution of element growth and confirm potential heterochrony based not only on general shape but also on organogenesis (process of developing structures).
The studied elements of conodont apparatuses come from the Kowala locality in the southern part of the Kielce region (central Poland) of the Holy Cross Mountains (Fig. 1). The section is exposed in the active quarry located in the southern limb of the Gałęzice-Bolechowice Syncline (Szulczewski, 1971; Racki et al., 2002). The Kowala quarry strata range from the middle Givetian carbonate sediments to the Lower Carboniferous (lowermost Tournaisian) shales, marls, and marly shales, which are unconformably overlain by the Permian conglomerates (see Marynowski et al., 2011, 2017). The uppermost Frasnian and Famennian part contains a record of all the major Devonian marine anoxic events: the Kellwasser (Joachimski et al., 2001; Racki et al., 2002; Bond et al., 2004), Annulata (Bond and Zatoń, 2003; Racka et al., 2010; Hartenfels, 2011), Dasberg (Marynowski et al., 2010), and Hangenberg black shales (Marynowski and Filipiak, 2007; Marynowski et al., 2012; Myrow et al., 2014).
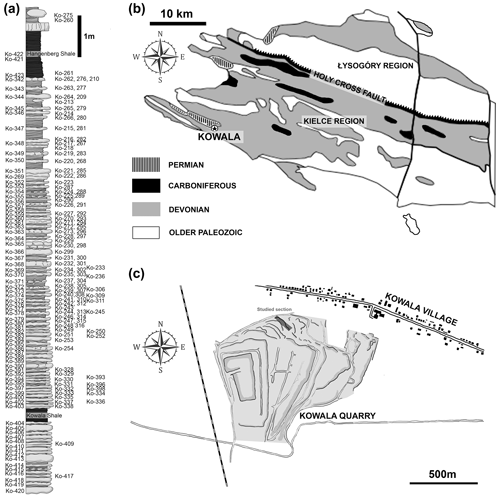
Figure 1Geological settings. (a) The studied section from the upper Famenian with the Hangenberg Black Shale and Kowala Black Shale. (b) Locality of Kowala quarry (marked by a star) in the geological context of the Holy Cross Mountains (c).
The Kowala locality is known for being very fossiliferous and not diagenetically altered. The preservation of some fossils is exquisite (Zatoń et al., 2014). Among arthropods there are thylacocephalans with preserved carapace microstructures (Broda and Zatoń, 2017) and remains of phyllocarids and angustidontids (Zatoń et al., 2014; Broda et al., 2018). Other fossils worth mentioning are conulariids (Sendino et al., 2017), non-biomineralized algae, and coelacanth fish species that fed on conodonts (Zatoń et al., 2017). The conodont record in Kowala is abundant and includes clusters of elements (Racki et al., 2002; Zatoń et al., 2017) and the natural assemblage of the Ctenopolygnathus brevilaminus apparatus (Świś et al., 2020). The isolated elements collected from the section provided a set of phylogenetic, biostratigraphic, and paleoecological data (Racki and Szulczewski, 1996; Dzik, 1997, 2002, 2006, 2008; Świś, 2019; Świś and Dzik, 2021; Rakociński et al., 2016; Dzik et al., 2022; Pisarzowska et al., 2020). This study is based on the isolated elements from the topmost part of the section ranging from the Kowala Black Shale (Marynowski and Filipiak, 2007) to the Hangenberg Black Shale (Dzik et al., 2022).
The rock samples were obtained from 2013, 2016, and 2017 field trip campaigns from the Famennian part of Kowala quarry (Dzik et al., 2022). The conodont elements were extracted with standard procedure of dissolving carbonate rocks using 10 % acetic acid. After this the residuum was decanted and allowed to dry. The preliminary separation of the material was possible due to the use of an electromagnetic Franz separator. After this step the elements are picked from non-electromagnetic fraction manually with a binocular microscope. The 127 P1 elements were assigned to D. marburgensis or D. trigonica species; however, only 85 elements were complete to the extent that biometric values can be measured. From those elements 81 P1 elements with fully preserved platform outline were treated with principal component analysis (PCA). From those, 34 P1 elements with well-preserved and clean basal cavity regions were picked for incremental layer examination. The majority of elements had free blade damage so the measurements of this part were not conducted.
3.1 Taxonomic identification
In this paper, I am applying the taxonomic classification introduced by Dzik (2006), who used a population approach to define the species. They defined a given species based on the most distinguishable characteristics present in the entire apparatus, regardless of whether the element that bears these traits is easily observable or not. Once the apparatus structure of a species is determined, individual specimens can be accurately identified and classified based on their diagnostic features. Morphology alone is usually not sufficient to determine the species identity, especially when specimens fall at the extreme ends of a continuous spectrum of population variability. In such cases, the identification is based on the co-occurrence of the specimen with other taxonomically identifiable specimens in the same sample.
The Dasbergina trigonica apparatus includes one of the most easily recognizable P1 elements occurring in the uppermost Famennian rocks. The most diagnostic feature of D. trigonica is the shape of basal cavity that is bifurcated in the caudal part of the element, as described by Dzik (2006). The composition of its apparatus remains superficially known, mostly because its ramiform elements are similar to those of co-occurring idiognathodontids and are difficult to differentiate from them (Dzik, 2006; Dzik et al., 2022). However, Dzik (2006) illustrated probable S1 elements for this species based on the material from the Dzikowiec locality in the Sudetes. The species was first introduced by Ziegler (1962) as Pseudopolygnathus trigonicus and later reinterpreted as Pseudopolygnathus marburgensis trigonicus by Sandberg and Ziegler (1979). They reduced its rank to subspecies because two variants (P. marburgensis marburgensis and P. marburgensis trigonicus) with characters earlier considered diagnostic for species may co-occur in the same sample. However, owing to data from the continuous latest Famennian sections in Kowala and Dzikowiec (Dzik, 2006; Dzik et al., 2022), it seems reasonable that these forms belong to an anagenetic series. Therefore, Dzik (2006) presented a diagnosis for D. marburgensis different from D. trigonica based on the basal cavity morphology. The diagnosis describes transversely elongated lobes of the basal cavity with parallel margins. Both of these taxa are morphologically closer to the genus Dasbergina introduced by Schäfer (1976) than to the type species of Pseudopolygnathus. The genus is probably rooted in Pandorinellina vulgaris as an early idiognathodontid (Dzik, 2006). The closest relative to Dasbergina marburgensis and its probable ancestor was Dasbergina kowalensis described by Dzik (2006), which has convergent features to those of idiognathodontids, including the parapet on the rostral part of the element near the cusp. Thus, D. kowalensis possibly originated from Pandorinellina vulgaris with P1 element superficially similar to Carboniferous species of Gnathodus.
The occurrences of these species were reported from different parts of the Euramerica shore, from former Laurentia (the modern North American region; Over, 1992, 2021) but also in the shallow seas of the eastern part (Dzik, 2006; Kalvoda et al., 2015; Becker et al., 2021; Feist et al., 2021; Dzik et al., 2022; Matyja et al., 2021). There were also occurrences from modern southern Europe, corresponding to the southern part of the Rheic Ocean (Kaiser et al., 2008; Mossoni et al., 2015; Spalletta et al., 2021), and in the Palaeotethys on the northern shore of Gondwana (Najjarzadeh et al., 2020; Hartenfels and Becker, 2018). Studied conodont elements were also present in the Northern Hemisphere in present-day Vietnam and China (Dzik et al., 2022; Paschall et al., 2019; Qie et al., 2016, 2021; Qiang et al., 1988) and reach the far northern shores of Siberia (Plotitsyn and Gatovsky, 2019; Nemirovskaya et al., 1993). The only region where D. marburgensis or D. trigonica were not recognized are far southern regions, where data on the conodont fauna are limited.
3.2 Calibration of ontogeny
Exposed crown tissue increments within the basal cavity imply a lack of the mineralized dentine-like basal body that may obliterate these increments. For the purpose of this study, only elements with non-preserved (un-mineralized) basal bodies are of interest. They are relatively common and well preserved in the latest Devonian strata exposed in the Kowala Quarry. This allows the estimation of the age of individuals and tracking the ontogenetic changes in a given lineage. Herein, I used a scanning electron microscope (SEM) to measure the length of elements and capture the image of the aboral side of 34 P1 elements of Dasbergina from 12 rock beds. For each element I was then able to count the number of incremental layers. The new branch development on each element is reflected by the basal cavity by changing the trajectory of incremental growth. I considered increments that are at the beginning of the formation of the branch (new functional area of elements independent from the main row of denticles) only when the direction of the new increment is dramatically changing, forming a surface twice thicker than the previous increment. This change in the development of the new functional branch can be associated with a relative time duration of formation and can be compared between different specimens across the section beds (see Fig. 3).
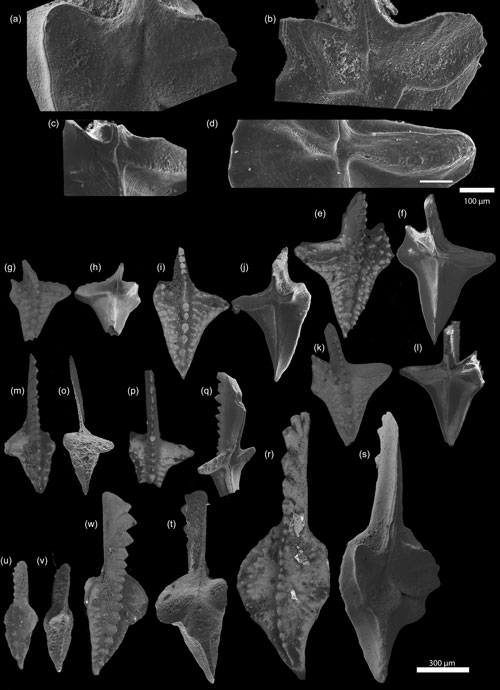
Figure 2SEM photographs of Dasbergina P1 elements from the latest Famennian of Kowala. There is visible change in shape, reduction in the basal cavity, and an increase in inversion. (a–d) Basal cavity of D. marburgensis ZPAL C16/3095 from sample Ko-408 (a) and D. trigonica ZPAL C16/3096 from samples Ko-248 (b), ZPAL C16/3097 Ko-281 (c), and ZPAL C16/3098 Ko-266 (d). (g–v) Oral and aboral sides of D. trigonica from samples Ko-281 ZPAL C16/3099, ZPAL C16/3100, ZPAL C16/3101, and ZPAL C16/3102 (g–l); Ko-321 ZPAL C16/3103 (m–o); and Ko-299 ZPAL C16/3104 (p–q). Oral and aboral sides of D. marburgensis ZPAL C16/3095, ZPAL C16/3105, and ZPAL C16/3106 from sample Ko-408 (s–v).
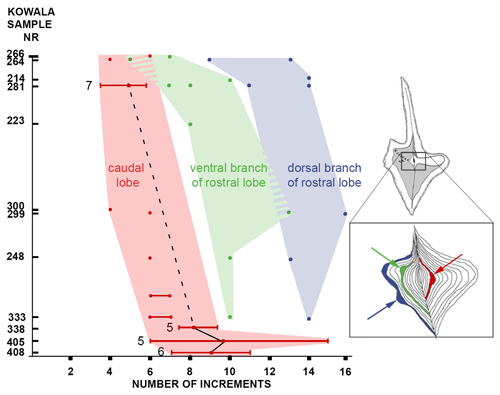
Figure 3Evolutionary transformation of ontogeny in the lineage of Dasbergina marburgensis → D. trigonica shown by the number of increments at which the widening or the bifurcation of the platform lobe occurred. The beginning of branch development is shifted to earlier stages of ontogeny with ongoing anageny. Horizontal lines indicate standard deviation from the mean values.
3.3 Morphometric measurements
The 85 P1 elements of Dasbergina were selected and photographed using an Olympus E-510 camera mounted on an Olympus SZX10 microscope. Some of the elements were additionally imaged in a scanning electron microscope (SEM). The measurements were done with ImageJ software (Schneider et al., 2012) chosen to capture the proportion, angle changes, and discrete features to capture the most meaningful change in morphology. The following measurements (Fig. 4) were made: length of the platform excluding free blade (L1); length from the cusp to the dorsal tip (L2); number of denticles (n); angle between dorsal denticle row; cusp and the rostral lobe edge (α); angle between two caudal branches (if identified) and cusp (β) and between the ventral-caudal branch, cusp, and the base of the free blade (γ). In order to perform biometrical analysis, I used the outline of the platform without the free blade part, which is in many cases damaged. I only used complete elements, which limits the number of measurements.
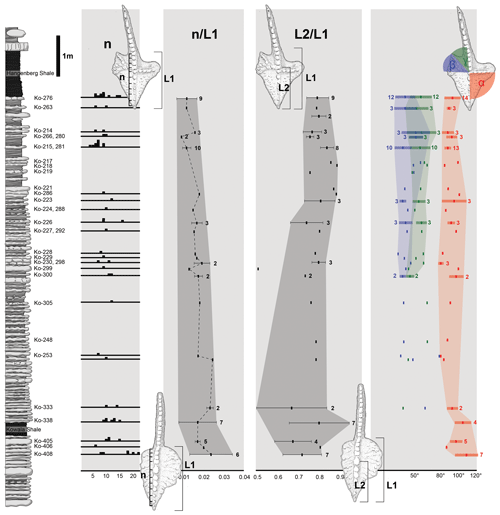
Figure 4Stratophenetic chart of character development of the Dasbergina marburgensis → D. trigonica line. (a) Change in the denticle numbers (n). (b) Changes in the number of denticles (n) according to the length of the platform (L1). (c) Changes in the position of the cusp on the platform measured by the relation of platform length and the distance between ventral edge of the platform and cusp (L2). (d) Changes in the angle between the ventral edge of the platform cusp and the edge of the rostral branch (α), changes in angle between both caudal branches (β), and changes in angle between the dorsal-most caudal branch and carina (γ). Horizontal lines indicate standard deviation from the mean values of feature in sample.
3.4 Statistical analysis
To capture the changes in the platform outline I used nine homologous landmarks: the cusp (1); the rostral side of free blade base (2); the most distal part of rostral lobe (3); the rostro-dorsal notch (4); the dorsal tip of the platform (5); the caudal dorsal notch (6); the dorsal caudal edge of the branch or the furthest point on the dorsal caudal side if the branch is not distinguishably developed (7); the ventral caudal edge of the branch or the furthest point on the ventral caudal side if the branch is not distinguishably developed (8); and the caudal side of free blade base (9). The 90 semi-landmarks were digitized from LM2 to LM9: from LM2 to LM6 there are 15 semi-LM between two LM and from LM6 to LM9 there are 10 semi-LM between two LM (Fig. 5). In order to obtain only morphological information, I performed a general Procrustes superimposition. Based on previous steps and related morphological information, I was able to perform multivariate analysis, namely principal component analysis (PCA) and canonical variate analysis (CVA). Using PCA 1 (43 % of the variance) and the length of the platform (L1), I estimated the trajectory of ontological change in platform in time. I applied canonical variate analysis (CVA) on the same dataset using the MorphoJ software (Klingenberg, 2011) in order to maximize inter-group variance and depict potential gradual transformation of the groups. The PCA and analysis of outline measurement and covariance test were made using the TPSdig and TPSutil software (Rohlf, 2015; Klingenberg, 2011). The resulting outputs are available on an osf data storage.
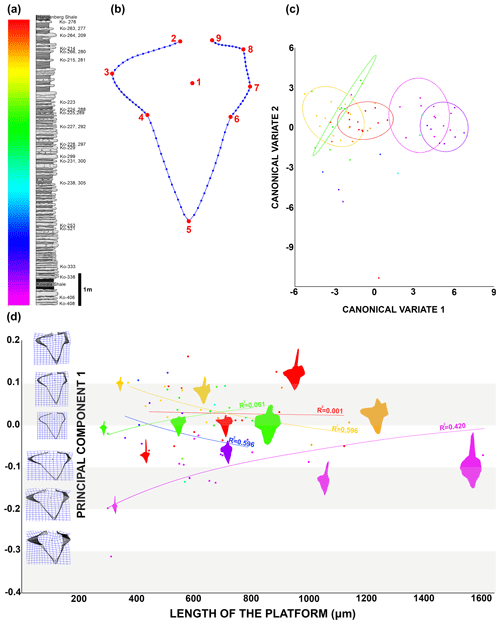
Figure 5Morphometric analysis results. (a) The studied section showing colour-coded identification elements and beds. (b) Diagram of the Dasbergina P1 element with landmarks (red) and semi-landmarks (blue). (c) Canonical variate analysis depicting elements coloured according to bed identification in (a), along with confidence ellipses (90 %). (d) Chart illustrating the ontogenetic transformation of the Dasbergina P1 element outline. Principal component 1 (accounting for 43 % of the variance) is represented on the y axis, while the indicator of ontogenetic stage length of the platform is shown on the x axis.
In the studied section of Kowala, Dasbergina marburgensis first occurs about 0.5 m below the Kowala Black Shale (sample Ko-408) and is consistently present up to the base of the Hangenberg Black Shale (sample Ko-276; Fig. 1). The outline of the platform of its P1 elements gradually changes from oval to polygonal, eventually developing processes on both sides of the carina. In particular, the caudal process bifurcates, developing the typical morphology of D. trigonica (Fig. 2).
The smallest P1 elements from the oldest studied sample Ko-408 (Fig. 6) are narrow and almost symmetrical. Juvenile elements in their dorsal part are slightly bent in the oral–aboral axis. While growing, their caudal platform lobe became wider and relatively rounded in outline. Their rostral lobe is larger, and the margin of the platform lacks any ornamentation at this stage. The free blade is relatively long with wide denticles. The basal cavity is wide and asymmetric, resembling the one in Dasbergina stabilis.
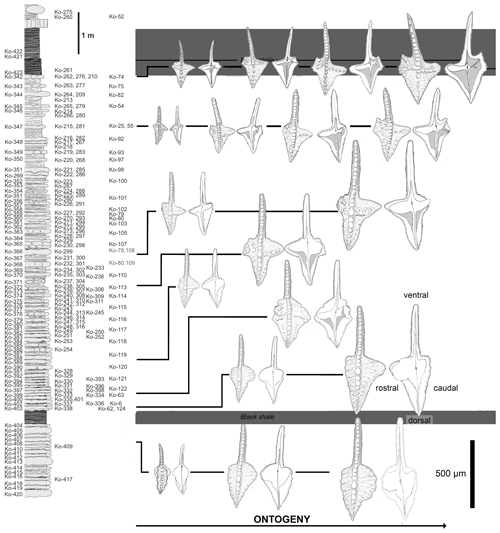
Figure 6Distribution of morphologies at particular ontogenetic stages within the Dasbergina marburgensis → D. trigonica lineage in the latest Devonian of Kowala.
The specimens of Dasbergina found immediately above the Kowala Black Shale (sample Ko-338) are similar in shape to the previous ones, but they are more angular in shape, and their rostral branch developed to form larger individuals. A single specimen recorded in the following bed (Ko-333) looks like a gerontic individual. It has more than 40 increments observed within the basal cavity, and its platform tends to be slightly angular in shape with an extended rostral lobe. The margins of the platform are prominently ornamented. The basal cavity is similar to that observed in specimens of the same size from below the Kowala Black Shale but with a deeper incision in the dorsal part of the caudal lobe. Probably co-occurring with the specimen maturity, linearly arranged denticles developed aside the carina along with chaotically distributed tubercles on its occlusal surface. The caudal part of the basal cavity is subdivided. Its rostral branch is longer and almost reaches the margin of the element.
Juvenile elements recovered from samples taken about 1 m above the Kowala Black Shale (Ko-320 to Ko-305) show a more angular outline. Their basal cavity is broad. Larger specimens are more triangular in the caudal platform part than specimens occurring in lower beds in the section and tend to develop a tongue-like lobe in their rostral part. In larger specimens, the platform becomes wider, and its overall shape is more triangular and more asymmetric, the rostral part being larger. Nodes and subtle ornamentation develop at the platform margin. In the rostral part of the basal cavity, a slight indentation develops. The shape of the platform is conform to the basal cavity. In its dorsal part, the cavity becomes narrower until developing a constricted furrow along the free carina. Even the largest collected specimen has the rostral part of the platform round in shape with a subtle ornamentation.
In the middle part of the studied section (samples Ko-304 to Ko-230), the smallest elements have the rostral lobe more expanded but do not bear denticulation. Both the caudal and rostral parts of the platform are acute, and the whole platform developed a triangular shape. The caudal lobe is variable in shape, but in at least some adult specimens it develops a slightly rectangular outline. Higher in the section, juvenile specimens present a rather narrow caudal lobe, but in the course of ontogeny it splits into two branches. The rostral and caudal part of the basal cavity are also very narrow. Ventrally, the basal cavity becomes narrower close to the basal cavity tip and stays narrow towards the element tip. The caudal side of the platform is more angular and, unlike the juvenile specimens recorded in older samples, the two branches of the basal cavity make a V-shaped structure. The basal cavity corresponds to a surface smaller than in specimens from older beds. Its dorsal part is narrow, beginning from near the basal pit. In this respect, the juvenile forms are similar to the adult specimens recovered from older beds. Adult specimens from these lower strata show a more extensive inversion of the basal cavity.
Above this section, juvenile elements have a very narrow basal cavity. Specimens of intermediate size develop a caudal bifurcation of the basal cavity and a rostral ridge like the mature individuals recovered in older samples. V-shaped caudal branches are ornamented by denticles on the oral side of the platform. Denticles are more abundant, and the inversion of the basal cavity increases.
In the bed immediately below the Hangenberg Black Shale, juvenile specimens present a basal cavity extremely narrow. The ridges on the caudal side already develop in the earliest phases of ontogeny. In the course of development, the basal cavity becomes increasingly inverted. More pronounced denticles on the oral side mark the rostral branch. The caudal V-shaped branches are present at all development stages, the dorsal one being longer in the largest specimens.
During anagenesis, the general shape of the conodont elements evolves into a more rectangular outline. The rostral branch gradually expanded along with the development of two caudal branches. The denticulation appears after the branches developed. The wide denticles on the platform part of the carina changed during anagenesis (Fig. 4a). The denticles are less numerous as relates to the length of the platform in geologically younger specimens (Fig. 4b). This pattern suggests a decreasing role of carina denticles in feeding with respect to denticles on the platform branch. The cusp position also changes (Fig. 4c), starting from a wide spectrum (from the half of the platform length to an extremely ventral position) to a ventral position observed in the specimens from the youngest beds. The location of the cusp is not related to the ontogenetic stage. A change is also visible in the angle between the rostral branch, cusp, and dorsal part of the carina. It shifts from the obtuse angle (100–120∘) in the specimens below the Kowala Shale to an almost right angle in the strata near the Hangenberg Shale (Fig. 4c). The angle between the line relying on V-shaped caudal branches and ventral branches and carina seems to be more variable in younger beds.
In order to better show the changes in overall shape of the platform in the Dasbergina marburgensis → Dasbergina trigonica chronospecies of the studied section, I used canonical variate analysis (CVA). The CVA shows an almost gradual trend towards elements that are more angular with more distinct caudal branches. However, after having developed such characters, the specimens recovered from the upper part of the section again become more round and compact in shape. This pattern seems like a return to a previous state with preservation of Dollo's law (Gould, 1970), also known as the law of irreversibility. In the present case, the increase in platform surface was achieved by the development of rounded and expanded branches not by lobe expansion. The similar outline was therefore achieved by adaptation of new structures, not by a reverse evolution. Thus, all the features related to advanced evolution, such as the inversion of the basal cavity, bifurcation of aboral lobes, and branch development, are still present, but the outline becomes more round, superficially resembling the ancestor state. This change observed in the most advanced forms is peculiar when considering that conodont elements are oral apparatus parts involved in food processing. Along the section, the gradual changes in platform outline and the development of branches suggest a redirection to a more specialist rather than an opportunist feeding strategy. The re-occurring of rounded elements in the platform in the most advanced members of the considered lineage could hint toward a switch in diet to a more opportunistic food source just before the Hangenberg event. However, the data presented in this account are not sufficient proof for it, and further studies to better assess the trophic level (e.g. the ratio) are needed.
To illustrate the trajectory of ontological changes, I compiled data about the ontological stage (platform length), with the PC1 reflecting around 43 % of the variance (Fig. 5d). The results show gradual changes in elements outline, with the earliest stages following the shape of the adult specimens in anageny. The evolution of the aboral side of the elements is mainly represented by the gradual reduction and inversion of the basal cavity, which impacts on the diagnosis of the species. The basal cavity evolves following the development of branches and by forming lobes that reproduce structures on the oral site. This pattern gives us the opportunity to see the increments inside the basal cavity and to calibrate the ontogeny and branch development in these chronospecies. The time of bifurcation of the caudal lobe can be calibrated thanks to the observation of incremental intervals. The branching of the basal cavity developed with local thickening of increments that is not necessarily reflected in the oral side morphology. In the oldest samples, the rostral branch develops at the 8th to 10th increments. In the course of evolution, this change occurs during the 6th or 7th increment, and in the uppermost bed it occurs at the 4th or 5th increment. Therefore, the time necessary to develop a branch was reduced by a half within the D. marburgensis → D. trigonica lineage. In the case of the two other branches, this event occurs at about the 10th increment. It appears that the intrinsic control of bifurcation was rather loose, but in the course of evolution this duration changed from 14 increment cycles (plus an unknown number of cycles preceding mineralization) to 12 cycles (days?).
Thus, it seems like the morphology of elements in juvenile specimens became more and more similar to the adult specimens in the course of anageny. The stages that in lower stratigraphic beds are visible only in the adult elements can be observed in the juvenile specimens occurring in higher beds. The branch development process is beginning earlier in more evolved specimens, which illustrates the gradual shift toward more juvenile individuals (Fig. 3). Therefore, the anageny of ontogeny in this lineage represents a good example of heterochrony that occurs when there are shifts in the timing or rate of development compared to ancestor forms (McNamara, 2012). In the case of the D. marburgensis → D. trigonica transition, we observe peramorphosis, which is when an organism grows and develops advanced traits earlier than its ancestors did (here, the early development of branches). According to data gathered by the increments analysis, it is reasonable that peramorphosis occurs through a pre-displacement mechanism, which means that growth and development of advanced characteristics are achieved earlier than in the ancestors (Fig. 4). Heterochrony in conodonts was observed in several cases (Souquet et al., 2022; Mei et al., 2004; Broadhead and MeComb, 1983; Renaud and Girard, 1999; Mellgren and Eriksson, 2006; Kiliç et al., 2016; Lambert et al., 2003); however, the majority of the cases correspond to paedomorphosis. The temperature is proposed as a cause for conodont heterochrony (Souquet et al., 2022). However, in the case of D. marburgensis → D.trigonica paleotemperature estimates in the Upper Devonian Kowala section (De Vleeschouwer et al., 2013) instead show a decreasing trend. According to the data shown here, the pre-displacement mechanism could be instead related to a specialization in diet regime in a relatively stable environment.
In the conodont literature, the term “morphotype” is widely used to designate a morphology that somehow deviates from the typical morphology of a given species. Morphotype can also express different ontogenetic trajectories, which in some conodonts may possibly have developed during larval stages with different diet regimes (Dzik, 2008; Shirley et al., 2018). Some authors named morphotypes or subspecies members of the same anagenetic continuum and referred to them as chronospecies (Dzik, 1985, 1987). In other cases, morphometrics allows the recognition of various “subspecies” as parts of the ontogenetic variability (Girard et al., 2022). The two subspecies Pseudopolygnathus marburgensis marburgensis and P. marburgensis trigonicus with partially overlapping stratigraphic ranges (Sandberg and Ziegler, 1979) represent a case study of the application of the concept of chrono(sub)species, defined by arbitrarily chosen characters (or morphotypes). Such morphotypes by definition do not change in geological time. They emerge suddenly (when the diagnostic characters are observed in the course of evolution in at least a single specimen) and overlap in range with their successors, which have been defined in the same way. This approach does not make sense from a biological point of view that takes into account evolution within populations showing some range of morphological variability. If the alternative biological concept of evolving species is applied, a chronospecies is defined on the basis of the modal value of its population variability. Such species do not emerge suddenly, but a transitional series of populations can be arbitrarily subdivided and attributed to the older or younger segment (chronospecies) of an evolutionary continuum. This pattern can be partially hidden by the compaction of sediments or the occurrence of hiatuses in the fossil record, which artificially produce abrupt morphological changes (Hohmann, 2021).
This is also the case with the Dasbergina marburgensis → D. trigonica lineage (Dzik, 2006). In biology, subspecies are usually considered a geographic race, which means that different subspecies of the same biological species could not occur in the same place. In palaeontology, the concept of chronosubspecies is frequently used to designate segments of an evolutionary continuum with a morphological range narrower than that considered to characterize a standard chronospecies. The crucial issue is not in the taxonomic rank, but in the way the taxonomic unit is defined. In this particular case, I consider samples from around the Kowala Black Shale as representing typical D. marburgensis and those from near the Hangenberg Black Shale as representing D. trigonica. It is somewhat arbitrary to decide to which (chrono)species the specimens from the middle part of the studied section belong to.
The biological concept of species supposes the ability of populations to inter-reproduce and thus exchange their genetic pools. This is obviously not possible to be used as a test of species designation in palaeontology, but in the case of fossil assemblages with negligible time averaging the morphological concept of species is applied, as it is usually done in biology. In the case of chronospecies, which are composed of a series of populations with different geological age, one may assume that there was a continuum in the genetic pool transfer in time-successive samples from the same locality (section) if specimens show a close morphologic similarity. This can be assumed also in the case of the Dasbergina marburgensis → D. trigonica lineage record in Kowala. The presence of the same lineage in places as distant from Kowala as the Vietnam Cát Bà locality (Dzik et al., 2022) suggests that the anagenetic (phyletic) evolution of the lineage took place in a large and geographically extended metapopulation.
Biostratigraphy uses approaches based on environmental control of species distribution (Klapper, 2007; Corradini et al., 2017) or hypothetical phylogeny of marker fossils or their phyletic evolution (Dzik, 1995; Dzik and Phong, 2016; Dzik et al., 2022). The biological entities that serve as geological markers have been living organisms, and as such their presence depends on ecological factors. Therefore, changes in environmental conditions, species-specific interactions, and migrations could result in a temporary disappearance or appearance in the geological record. Such a complex pattern could produce an apparent diachroneity in the paleontological record. However, we can also build our biostratigraphical interpretations on the evolutionary changes, which influences the organisms through selective pressure. Evolution is a continuous process that leads to an increase in morphological differences, and genetic isolation and speciation can eventually occur in isolated populations. More globally distributed species can develop global metapopulations containing subpopulations capable of mixing gene pools with each other (Millstein, 2010). This pattern may result in synchronous evolution and the occurrence at a global scale of the same morphology. When this process occurs, the fossil marker represents a reliable stratigraphic marker, and the changes in this chronospecies have an anagenetic character. The anagenesis refers to the evolutionary process through which a chronospecies undergoes gradual, directional changes over successive generations, leading to the transformation to chronospecies with different morphological features, without a sudden cutting point as occurs in speciation.
Conodont elements are highly valuable and extensively applied tools in biostratigraphy (Ferretti et al., 2020). Their advantages in stratigraphy stem from their widespread occurrence, easy acquisition, efficient extraction methods, widespread distribution, and high rate of evolutionary change. As a result, conodonts have proven to be useful in dating marine carbonate rocks across various time intervals, ranging from the Late Cambrian to the end of the Triassic, including the Late Devonian (upon which this article focuses). However, the question remains whether we can improve biostratigraphy by incorporating the anagenesis pattern.
The latest Devonian conodont biozone of the widely used Sandberg and Ziegler (1996) scheme is based upon the range of the species Siphonodella praesulcata Sandberg et al. (1972). The co-occurrence of P elements of its apparatus with S elements classified in Dinodus suggests that it belongs to the same apparatus. It has to be referred to as Dinodus praesulcatus, the senior synonym (Cooper, 1939). Unfortunately, the evolutionary root of this lineage of importance for the biostratigraphy of the Tournaisian remains unknown (Świś and Dzik, 2021). Moreover, there are problems with the identification of P1 elements of this species because of its high variability and rarity (Kaiser and Corradini, 2011). This makes its use as a zonal marker problematic. It seems that D. praesulcatus did not emerge synchronously in various places, a general problem with the application of the first appearance datum (FAD) in biostratigraphy (Dzik et al., 2018). In Kowala (central Poland), the FAD of D. praesulcatus precedes the FAD of Pseudopolygnathus ultimus (Dzik et al., 2022), whereas most authors reported a reverse order in other localities (e.g. Spalletta et al., 2017). This pattern is apparently a result of migration (Dzik et al., 2018, 2022). The same consideration can apply to the expansa zone (Dzik et al., 2022). The species giving its name to this zone, Tripodellus expansus (Sandberg and Ziegler, 1979), is missing in Kowala, so it is not possible to use it in dating this particular section. To avoid these difficulties, Corradini et al. (2016), Spalletta et al. (2017), and Kaiser et al. (2020) replaced the latest Famennian praesulcata and expansa zones with ultimus and kockeli zones based on occurrences of Pseudopolygnathus ultimus and Protognathodus kockeli, respectively. The former species appears indeed as a convenient zonal marker, but P. kockeli presents the same limits already shown by Sandberg and Ziegler (1996), having unknown ancestors and mainly not appearing synchronously in various regions of the world. Using the data presented in this article, we can correlate sedimentary rocks based on the anagenetic state of D. marburgensis → D. trigonica. The best feature to be considered is the basal cavity, namely the smaller the surface of the basal cavity and the greater the inversion related to the outline of the platform, the younger the rocks (closer to Hangenberg Black Shale). This can be used as an alternative method to the actual zonation or as a supplementary tool to get more stratigraphic precision.
The two subspecies commonly referred to in the literature as Pseudopolygnathus marburgensis marburgensis and P. marburgensis trigonicus form a morphological continuum than can translate chronospecies, namely Dasbergina marburgensis → D. trigonica. Based on morphometric studies involving 85 elements from the Kowala section in the Holy Cross Mountains, I was able to describe the anagenetic evolutionary change as an alternative approach to classical morphological analysis.
In synthesis, during anagenesis the shape of the elements becomes more rectangular, with the rostral branch expanding along with the development of two caudal branches, followed by the appearance of denticulation. The broad denticles on the carina evolve into rarer denticles in younger specimens. The variability in cusp position evolves from a wide spectrum to a strictly ventral position in younger beds, regardless of the ontogenetic stage. The angle between the rostral branch, cusp, and dorsal carina shifts from obtuse to an almost right angle in strata near the Hangenberg Shale. The angle between the V-shaped caudal branches, including the more ventral one, appears more variable in younger beds.
The timing of the bifurcation occurring in the caudal lobe was calibrated based on incremental layers observed in different specimens. In the oldest samples, the rostral branch appears at the 8th to 10th increment, and it is reduced by a half to appearing at the 4th or 5th increment in the uppermost beds. For the other branches, bifurcation control seems to be loose initially but, with evolution, the timing shifts from 14 cycles of increments to 12 cycles. The shift in branch development and the changes in platform structures suggest the occurrence of a peramorphosis process, which corresponds to heterochrony in this lineage. The observed anageny from D. marburgensis to D. trigonica could be usefully applied to improve stratigraphic schemes.
The data are available on the osf.io platform with following DOI: https://doi.org/10.17605/OSF.IO/JNHT7 (Świś, 2023).
The samples are housed in the Institute of Evolutionary Biology of the University of Warsaw.
The author has declared that there are no competing interests.
Publisher's note: Copernicus Publications remains neutral with regard to jurisdictional claims made in the text, published maps, institutional affiliations, or any other geographical representation in this paper. While Copernicus Publications makes every effort to include appropriate place names, the final responsibility lies with the authors.
I would like to express my gratitude to my mentor Jerzy Dzik, whose articles inspired me to conduct this research. I am also very grateful to all the people who were interested and took the time to discuss with me about different aspects of this work. I am thankful to two anonymous reviewers who pointed out problems in the manuscript and contributed to its improvement. Finally, I would like to thank editors who were watching over manuscript improvement progress and were exceptionally patient and understanding.
This paper was edited by Emanuela Mattioli and reviewed by two anonymous referees.
Armstrong, H. A. and Smith, C. J.: Growth patterns in euconodont crown enamel: Implications for life history and mode-of-life reconstruction in the earliest vertebrates, P. R. Soc. B, 268, https://doi.org/10.1098/rspb.2001.1591, 2001.
Assemat, A., Thiery, G., Lieffroy, T., and Girard, C.: Shape diversity in conodont elements, a quantitative study using 3D topography, Mar. Micropaleontol., 184, 102292, https://doi.org/10.1016/j.marmicro.2023.102292, 2023.
Aze, T., Ezard, T. H. G., Purvis, A., Coxall, H. K., Stewart, D. R. M., Wade, B. S., and Pearson, P. N.: A phylogeny of Cenozoic macroperforate planktonic foraminifera from fossil data, Biol. Rev., 86, 900–927, https://doi.org/10.1111/j.1469-185X.2011.00178.x, 2011.
Becker, R. T., Hartenfels, S., and Kaiser, S. I.: Review of Devonian-Carboniferous Boundary sections in the Rhenish Slate Mountains (Germany), Palaeobio. Palaeoenv., 101, 357–420, https://doi.org/10.1007/s12549-020-00469-6, 2021.
Bond, D. and Zatoń, M.: Gamma-ray spectrometry across the Upper Devonian basin succession at Kowala in the Holy Cross Mountains (Poland), Acta Geol. Pol., 53, 93–99, 2003.
Bond, D., Wignall, P. B., and Racki, G.: Extent and duration of marine anoxia during the Frasnian-Famennian (Late Devonian) mass extinction in Poland, Germany, Austria and France, Geol. Mag., 141, 173–193, https://doi.org/10.1017/S0016756804008866, 2004.
Broadhead, T. W. and MeComb, R.: Paedomorphosis in the conodont family Icriodontidae and the evolution of lcriodus, Foss. Strat., 15, 149–154, 1983.
Broda, K. and Zatoń, M.: A set of possible sensory system preserved in cuticle of Late Devonian thylacocephalan arthropods from Poland, Hist. Biol., 29, 1045–1055, https://doi.org/10.1080/08912963.2017.1284834, 2017.
Broda, K., Collette, J., and Budil, P.: Phyllocarid crustaceans from the Late Devonian of the Kowala quarry (Holy Cross Mountains, central Poland), Pap. Palaeontol., 4, 67–84, https://doi.org/10.1002/spp2.1099, 2018.
Carr, T. D., Varricchio, D. J., Sedlmayr, J. C., Roberts, E. M., and Moore, J. R.: A new tyrannosaur with evidence for anagenesis and crocodile-like facial sensory system, Sci. Rep., 7, 44942, https://doi.org/10.1038/srep44942, 2017.
Cooper, C. L.: Conodonts from a Bushberg-Hannibal horizon in Oklahoma, J. Paleontol., 13, 379–422, 1939.
Corradini, C., Spalletta, C., Mossoni, A., Matyja, H., and Jeffrey Over, D.: Conodonts across the Devonian/Carboniferous boundary: A review and implication for the redefinition of the boundary and a proposal for an updated conodont zonation, Geol. Mag., 154, 888–902, https://doi.org/10.1017/S001675681600039X, 2017.
Czepiński, Ł.: New protoceratopsid specimens improve the age correlation of the upper cretaceous gobi desert strata, Acta Palaeontol. Pol., 65, 481–497, https://doi.org/10.4202/APP.00701.2019, 2020.
De Vleeschouwer, D., Rakociński, M., Racki, G., Bond, D. P. G., Sobień, K., and Claeys, P.: The astronomical rhythm of Late-Devonian climate change (Kowala section, Holy Cross Mountains, Poland), Earth Planet. Sc. Lett., 365, 25–37, https://doi.org/10.1016/j.epsl.2013.01.016, 2013.
Donoghue, P. C. J.: Growth and patterning in the conodont skeleton, Philos. T. R. Soc. B, 353, 633–666, https://doi.org/10.1098/rstb.1998.0231, 1998.
Donoghue, P. C. J.: Conodonts Meet Cladistics: Recovering Relationships and Assessing the, Palaeontology, 44, 65–93, 2001.
Donoghue, P. C. J. and Keating, J. N.: Early vertebrate evolution, Palaeontology, 57, 879–893, https://doi.org/10.1111/pala.12125, 2014.
Donoghue, P. C. J. and Purnell, M. a.: Mammal-like occlusion in conodonts, Paleobiology, 25, 58–74, 1999.
Donoghue, P. C. J., Forey, P. L., and Aldridge, R. J.: Conodont affinity and chordate phylogeny, Biol. Rev., 75, 191–251, https://doi.org/10.1111/j.1469-185X.1999.tb00045.x, 2000.
Du, Y., Chiari, M., Karádi, V., Nicora, A., Onoue, T., Pálfy, J., Roghi, G., Tomimatsu, Y., and Rigo, M.: The asynchronous disappearance of conodonts: New constraints from Triassic-Jurassic boundary sections in the Tethys and Panthalassa, Earth Sci. Rev., 203, 103176, https://doi.org/10.1016/j.earscirev.2020.103176, 2020.
Du, Y., Onoue, T., Tomimatsu, Y., Wu, Q., and Rigo, M.: Lower Jurassic conodonts from the Inuyama area of Japan: implications for conodont extinction, Front. Ecol. Evol., 11, 1135789, https://doi.org/10.3389/fevo.2023.1135789, 2023.
Dzik, J.: Typologic versus population concepts of chronospecies: implications for ammonite biostratigraphy, Acta Palaeontol. Pol., 30, 71–92, 1985.
Dzik, J.: Chordate affinities of the conodonts, in: Problematica fossil taxa, edited by: Hoffman, A. and Nitecki, M. H., Oxford University Press, Clarendon Press, 240–254, 1986.
Dzik, J.: The concept of chronospecies in ammonites, Atti del Second. Convegno Internazionale Foss. Evol. Ambient. Pergola, 2530, 273–289, 1987.
Dzik, J.: Emergence and succession of Carboniferous conodont and ammonoid communities in the Polish part of the Variscan sea, Acta Palaeontol. Pol., 42, 57–170, 1997.
Dzik, J.: The Origin of the Mineral Skeleton in Chordates, in: Evolutionary Biology, edited by: Hecht, M. K., Macintyre, R. J., and Clegg, M. T., Springer, Boston, MA, https://doi.org/10.1007/978-1-4615-4185-1_3, 2000.
Dzik, J.: Emergence and collapse of the Frasnian conodont and ammonoid communities in the holy cross mountains, Poland, Acta Palaeontol. Pol., 47, 565–650, 2002.
Dzik, J.: The chronophyletic approach: Stratophenetics facing an incomplete fossil record, Spec. Pap. Palaeontol., 159–183, 2005.
Dzik, J.: The Famennian “golden age” of conodonts and ammonoids in the Polish part of the Variscan sea, Palaeontol. Pol., 63, 1–360, 2006.
Dzik, J.: Evolution of morphogenesis in 360-million-year-old conodont chordates calibrated in days, Evol. Dev., 10, 769–777, 2008.
Dzik, J.: Evolutionary roots of the conodonts with increased number of elements in the apparatus, Earth Env. Sci. T. R. So., 106, 29–53, https://doi.org/10.1017/S1755691015000195, 2015.
Dzik, J. and Phong, N. D.: Dating of Cambrian-Ordovician boundary strata in northernmost Vietnam and methodological aspects of evolutionary biostratigraphic inference, Sratigraphy, 13, 83–93, 2016.
Dzik, J., Świś, P., and Phong, N. D.: The Frasnian-Famennian boundary in Vietnam and evolutionary meaning of FADs and LADs, Newsletters Stratigr., 51, 327–342, https://doi.org/10.1127/nos/2017/0418, 2018.
Dzik, J., Phong, N. D., Thuy, N. T., and Świś, P.: Evolution and migration of conodonts and ammonoids near the end of Devonian recorded in distant localities, Stratigraphy, 19, 27–50, 2022.
Epstein, A. G., Epstein, J. B., and Harris, L. D.: Conodont color alteration – an index to organic metamorphism, USGS Prof. Pap., 995, 1–25, 1977.
Feist, R., Cornée, J. J., Corradini, C., Hartenfels, S., Aretz, M., and Girard, C.: The Devonian–Carboniferous boundary in the stratotype area (SE Montagne Noire, France), Palaeobio. Palaeoenv., 101, 295–311, https://doi.org/10.1007/s12549-019-00402-6, 2021.
Ferretti, A., Bancroft, A. M., and Repetski, J. E.: GECkO: Global Events impacting COnodont evolution, Palaeogeogr. Palaeocl., 549, 109677, https://doi.org/10.1016/j.palaeo.2020.109677, 2020.
Georgescu, M. D., Saupe, E. E., and Huber, B. T.: Morphometric and stratophenetic basis for phylogeny and taxonomy in late cretaceous gublerinid planktonic foraminifera, Micropaleontology, 54, 397–424, https://doi.org/10.47894/mpal.54.5.02, 2008.
Gingerich, P. D.: The Stratophenetic Approach to Phylogeny Reconstruction in Vertebrate in: Phylogenetic Analysis and Paleontology, edited by: Cracraft, J. and Eldredge, N., Columbia University Press, New York, 41–78, https://doi.org/10.7312/crac92306-004, 1979.
Ginot, S. and Goudemand, N.: Conodont size, trophic level, and the evolution of platform elements, Paleobiology, 45, 458–468, https://doi.org/10.1017/pab.2019.19, 2019.
Girard, C., Cornée, J. J., Joachimski, M. M., Charruault, A. L., Dufour, A. B., and Renaud, S.: Paleogeographic differences in temperature, water depth and conodont biofacies during the Late Devonian, Palaeogeogr. Palaeocl., 549, 108852, https://doi.org/10.1016/j.palaeo.2018.06.046, 2020.
Girard, C., Charruault, A.-L., Gluck, T., Corradini, C., and Renaud, S.: Deciphering the morphological variation and its ontogenetic dynamics in the Late Devonian conodont Icriodus alternatus, Foss. Rec., 25, 25—41, https://doi.org/10.3897/fr.25.80211, 2022.
Gould, S. J.: Dollo on Dollo's law: Irreversibility and the status of evolutionary laws, J. Hist. Biol., 3, 189–212, https://doi.org/10.1007/BF00137351, 1970.
Guenser, P., Warnock, R. C. M., Donoghue, P. C. J., and Jarochowska, E.: Does time matter in phylogeny? A perspective from the fossil record, bioRxiv, 1–9, https://doi.org/10.1101/2021.06.11.445746, 2021.
Hartenfels, S.: Die globalen Annulata-Events und die Dasberg-Krise (Famennium, Oberdevon) in Europa und Nord-Afrika: hochauflösende Conodonten-Stratigraphie, Karbonat-Mikrofazies, Paläoökologie und Paläodiversität, Münsterische Forschungen zur Geol. und Paläontologie, 105, 17–527, 2011.
Hartenfels, S. and Becker, R. T.: Age and correlation of the transgressive Gonioclymenia Limestone (Famennian, Tafilalt, eastern Anti-Atlas, Morocco), Geol. Mag., 155, 586–629, https://doi.org/10.1017/S0016756816000893, 2018.
Hohmann, N.: INCORPORATING INFORMATION ON VARYING SEDIMENTATION RATES INTO PALEONTOLOGICAL ANALYSES, Palaios, 36, 53–67, https://doi.org/10.2110/palo.2020.038, 2021.
Jarochowska, E., Viira, V., Einasto, R., Nawrot, R., Bremer, O., Männik, P., and Munnecke, A.: Conodonts in Silurian hypersaline environments: Specialized and unexpectedly diverse, Geology, 45, 3–6, https://doi.org/10.1130/G38492.1, 2017.
Jarochowska, E., Bremer, O., Yiu, A., Märss, T., Blom, H., Mörs, T., and Vajda, V.: Revision of thelodonts, acanthodians, conodonts, and the depositional environments in the Burgen outlier (Ludlow, Silurian) of Gotland, Sweden, GFF, 143, 168–189, https://doi.org/10.1080/11035897.2021.1907441, 2021.
Jeppsson, L.: Notes on Some Upper Silurian Multielement Conodonts, GFF, 91, 12–24, https://doi.org/10.1080/11035896909448421, 1969.
Joachimski, M. M. and Buggisch, W.: Conodont apatite δ18O signatures indicate climatic cooling as a trigger of the Late Devonian mass extinction, Geology, 30, 711–714, https://doi.org/10.1130/0091-7613(2002)030<0711:CAOSIC>2.0.CO;2, 2002.
Joachimski, M. M., Ostertag-Henning, C., Pancost, R. D., Strauss, H., Freeman, K. H., Littke, R., Sinninghe Damsté, J. S., and Racki, G.: Water column anoxia, enhanced productivity and concomitant changes in δ13C and δ34S across the Frasnian-Famennian boundary (Kowala – Holy Cross Mountains/Poland), Chem. Geol., 175, 109–131, https://doi.org/10.1016/S0009-2541(00)00365-X, 2001.
Kaiser, S. I. and Corradini, C.: The early siphonodellids (conodonta, late devonian-early carboniferous): Overview and taxonomie state, Neues Jahrb. Geol. P.-A., 261, 19–35, https://doi.org/10.1127/0077-7749/2011/0144, 2011.
Kaiser, S. I., Steuber, T., and Becker, R. T.: Environmental change during the Late Famennian and Early Tournaisian (Late Devonian-Early Carboniferous): Implications from stable isotopes and conodont biofacies in southern Europe, Geol. J., 43, 241–260, https://doi.org/10.1002/gj.1111, 2008.
Kaiser, S. I., Kumpan, T., and Rasser, M. W.: High-resolution conodont biostratigraphy in two key sections from the Carnic Alps (Grüne Schneid) and Graz Paleozoic (Trolp) – implications for the biozonation concept at the Devonian-Carboniferous boundary, Newsl. Stratigr., 53, 249–274, https://doi.org/10.1127/nos/2019/0520, 2020.
Kalvoda, J., Kumpan, T., and Bábek, O.: Upper Famennian and Lower Tournaisian sections of the Moravian Karst (Moravo-Silesian Zone, Czech Republic): A proposed key area for correlation of the conodont and foraminiferal zonations, Geol. J., 50, 17–38, https://doi.org/10.1002/gj.2523, 2015.
Kelz, V., Guenser, P., Rigo, M., and Jarochowska, E.: Growth allometry and dental topography in Upper Triassic conodonts support trophic differentiation and molar-like element function, Paleobiology, 49, 665–683, https://doi.org/10.1017/pab.2023.8, 2023.
Kiliç, A. M., Plasencia, P., Ishida, K., Guex, J., and Hirsch, F.: Proteromorphosis of Neospathodus (Conodonta) during the Permian-Triassic crisis and recovery, Rev. Micropaleontol., 59, 33–39, https://doi.org/10.1016/j.revmic.2016.01.003, 2016.
Klapper, G.: Conodont taxonomy and the recognition of the Frasnian/Famennian (Upper Devonian) State Boundary, Stratigraphy, 4, 67–76, 2007.
Klapper, G. and Philip, G. M.: DEVONIAN CONODONT APPARATUSES AND THEIR VICARIOUS SKELETAL ELEMENTS, Lethaia, 4, 429–452, https://doi.org/10.1111/j.1502-3931.1971.tb01865.x, 1971.
Klingenberg, C. P.: MorphoJ: An integrated software package for geometric morphometrics, Mol. Ecol. Resour., 11, 353–357, https://doi.org/10.1111/j.1755-0998.2010.02924.x, 2011.
Krishtalka, L.: Anagenetic Angst, in: Species, Species Concepts and Primate Evolution, edited by: Kimbel, W. H. and Martin, L. B., Springer US, Boston, MA, 331–344, https://doi.org/10.1007/978-1-4899-3745-2_13, 1993.
Lambert, L. L., Heckel, P. H., and Barrick, J. E.: Swadelina new genus (Pennsylvanian conodonta), a taxon with potential chronostratigraphic significance, Micropaleontology, 49, 151–158, https://doi.org/10.2113/49.2.151, 2003.
Leonhard, I., Shirley, B., Murdock, D. J. E., Repetski, J., and Jarochowska, E.: Growth and feeding ecology of coniform conodonts, PeerJ, 9, e12505, https://doi.org/10.7717/peerj.12505, 2021.
Lindström, M.: A SUPRAGENERIC TAXONOMY OF THE CONODONTS, Lethaia, 3, 427–445, https://doi.org/10.1111/j.1502-3931.1970.tb00834.x, 1970.
Martínez-Pérez, C., Rayfield, E. J., Botella, H., and Donoghue, P. C. J.: Translating taxonomy into the evolution of conodont feeding ecology, Geology, 44, 247–250, https://doi.org/10.1130/G37547.1, 2016.
Marynowski, L. and Filipiak, P.: Water column euxinia and wildfire evidence during deposition of the Upper Famennian Hangenberg event horizon from the Holy Cross Mountains (central Poland), Geol. Mag., 144, 569–595, 2007.
Marynowski, L., Filipiak, P., and Zatoń, M.: Geochemical and palynological study of the Upper Famennian Dasberg event horizon from the Holy Cross Mountains (central Poland), Geol. Mag., 147, 527–550, https://doi.org/10.1017/S0016756809990835, 2010.
Marynowski, L., Rakociński, M., Borcuch, E., Kremer, B., Schubert, B. A., and Jahren, A. H.: Molecular and petrographic indicators of redox conditions and bacterial communities after the F/F mass extinction (Kowala, Holy Cross Mountains, Poland), Palaeogeogr. Palaeocl., 306, 1–14, https://doi.org/10.1016/j.palaeo.2011.03.018, 2011.
Marynowski, L., Zatoń, M., Rakociński, M., Filipiak, P., Kurkiewicz, S., and Pearce, T. J.: Deciphering the upper Famennian Hangenberg Black Shale depositional environments based on multi-proxy record, Palaeogeogr. Palaeocl., 346–347, 66–86, https://doi.org/10.1016/j.palaeo.2012.05.020, 2012.
Marynowski, L., Pisarzowska, A., Derkowski, A., Rakociński, M., Szaniawski, R., Środoń, J., and Cohen, A. S.: Influence of palaeoweathering on trace metal concentrations and environmental proxies in black shales, Palaeogeogr. Palaeocl., 472, 177–191, https://doi.org/10.1016/j.palaeo.2017.02.023, 2017.
Matyja, H., Woroncowa-Marcinowska, T., Filipiak, P., Brański, P., and Sobień, K.: The Devonian/Carboniferous boundary interval in Poland: multidisciplinary studies in pelagic (Holy Cross Mountains and Sudetes) and ramp (Western Pomerania) successions, Palaeobiodiversity and Palaeoenvironments, 101, https://doi.org/10.1007/s12549-020-00442-3, 2021.
Mazza, M. and Martínez-Pérez, C.: Evolutionary convergence in conodonts revealed by synchrotron-based tomographic microscopy, Palaeontol. Electron., 19, 1–11, https://doi.org/10.26879/681, 2016.
McCollum, M. and Sharpe, P. T.: Evolution and development of teeth, J. Anat., 199, 153–159, https://doi.org/10.1017/S0021878201008123, 2001.
McNamara, K. J.: Heterochrony: The Evolution of Development, Evol. Educ. Outreach, 5, 203–218, https://doi.org/10.1007/s12052-012-0420-3, 2012.
Mei, S., Henderson, C. M., and Cao, C.: Conodont sample-population approach to defining the base of the Changhsingian Stage, Lopingian Series, Upper Permian, Geol. Soc. Lond. Spec. Publ., 230, 105–121, 2004.
Mellgren, J. S. and Eriksson, M. E.: A model of reconstruction for the oral apparatus of the Ordovician conodont genus Protopanderodus Lindström, 1971, Earth Env. Sci. T. R. So., 97, 97–112, https://doi.org/10.1017/S0263593300001425, 2006.
Millstein, R.: The concepts of population and metapopulation in evolutionary biology and ecology, in: Evolution since Darwin: the first, 150, edited by: Bell, M. A., Futuyma, D. J., Eanes, W. F., and Levinton, J. S., Sinauer Associates is an imprint of Oxford University Press, Oxford, 61–86, ISBN 9780878934133, 2010.
Mossoni, A., Carta, N., Corradini, C., and Spalletta, C.: Conodonts across the Devonian/Carboniferous boundary in SE Sardinia (Italy), B. Geosci., 90, 371–388, https://doi.org/10.3140/bull.geosci.1524, 2015.
Müller, K. J. and Nogami, Y.: Über den Feinbau der Conodonten, Mem. Fac. Sci. Kyoto Univ. Ser. Geol. Mineral., 38, 1–109, 1971.
Murdock, D. J. E., Dong, X. P., Repetski, J. E., Marone, F., Stampanoni, M., and Donoghue, P. C. J.: The origin of conodonts and of vertebrate mineralized skeletons, Nature, 502, 546–549, https://doi.org/10.1038/nature12645, 2013.
Myrow, P. M., Ramezani, J., Hanson, A. E., Bowring, S. A., Racki, G., and Rakociński, M.: High-precision U-Pb age and duration of the latest devonian (Famennian) hangenberg event, and its implications, Terra Nov., 26, 222–229, https://doi.org/10.1111/ter.12090, 2014.
Najjarzadeh, M. T., Ashouri, A. R., Yazdi, M., and Bahrami, A.: Biostratigraphy of Devonian-Carboniferous boundary in Tuyeh-Darvar section, north of Iran, Iran. J. Earth Sci., 12, 98–123, https://doi.org/10.30495/ijes.2020.673332, 2020.
Nemirovskaya, T. I., Chermnykh, V. A., Kononova, L. I., and Pazukhin, V. N.: Conodonts of the Devonian-Carboniferous boundary section, Kozhim, Polar Urals, Russia, Ann.-Soc. Geol. Belgique, 115, 629–647, 1993.
Olempska, E.: Gradual evolutionary transformations of ontogeny in an Ordovician ostracod lineage, Lethaia, 22, 159–168, https://doi.org/10.1111/j.1502-3931.1989.tb01678.x, 1989.
Over, D. J.: Paleontological Society Conodonts and the Devonian-Carboniferous Boundary in the Upper Woodford Shale, Arbuckle Mountains, South-Central Oklahoma, Paleontological Society, 66, 293–311, 1992.
Over, D. J.: The Devonian-Carboniferous boundary in the United States, Palaeobio. Palaeoenv., 101, 529–540, https://doi.org/10.1007/s12549-020-00428-1, 2021.
Paschall, O., Carmichael, S. K., Königshof, P., Waters, J. A., Ta, P. H., Komatsu, T., and Dombrowski, A.: The Devonian-Carboniferous boundary in Vietnam: Sustained ocean anoxia with a volcanic trigger for the Hangenberg Crisis?, Global Planet. Change, 175, 64–81, https://doi.org/10.1016/j.gloplacha.2019.01.021, 2019.
Pearson, P. N. and Coxall, H. K.: Origin of the Eocene planktonic foraminifer Hantkenina by gradual evolution, Palaeontology, 57, 243–267, https://doi.org/10.1111/pala.12064, 2014.
Petryshen, W., Henderson, C. M., De Baets, K., and Jarochowska, E.: Evidence of parallel evolution in the dental elements of Sweetognathus conodonts: Sweetognathus Evolutionary Mechanisms, P. R. Soc. B, 287, 20201922, https://doi.org/10.1098/rspb.2020.1922, 2020.
Pisarzowska, A., Rakociński, M., Marynowski, L., Szczerba, M., Thoby, M., Paszkowski, M., Perri, M. C., Spalletta, C., Schönlaub, H. P., Kowalik, N., and Gereke, M.: Large environmental disturbances caused by magmatic activity during the Late Devonian Hangenberg Crisis, Glob. Planet. Change, 190, 103155, https://doi.org/10.1016/j.gloplacha.2020.103155, 2020.
Plotitsyn, A. N. and Gatovsky, Y. A.: New Conodont Species from the Famennian (Upper Devonian) of the Urals, Paleontol. J., 53, 629–635, https://doi.org/10.1134/S0031030119060108, 2019.
Qiang, J., Jiayong, W., Hongdi, W., Ning, W., and Xiaosong, L.: New Advances in the Study of the Devonian – Carboniferous Boundary Stratotype in Muhua, Changshun, Guizhou – An Introduction to the Daposhang Devonian-Carboniferous Boundary Section, Acta Geol. Sin.-Engl., 1, 349–363, https://doi.org/10.1111/j.1755-6724.1988.mp1004001.x, 1988.
Qie, W., Wang, X. D., Zhang, X., Ji, W., Grossman, E. L., Huang, X., Liu, J., and Luo, G.: Latest Devonian to earliest Carboniferous conodont and carbon isotope stratigraphy of a shallow-water sequence in South China, Geol. J., 51, 915–935, https://doi.org/10.1002/gj.2710, 2016.
Qie, W., Sun, Y., Guo, W., Nie, T., Chen, B., Song, J., Liang, K., Yin, B., Han, S., Chang, J., and Wang, X.: Devonian-Carboniferous boundary in China, 101, 589–611, https://doi.org/10.1007/s12549-021-00494-z, 2021.
Racka, M., Marynowski, L., Filipiak, P., Sobstel, M., Pisarzowska, A., and Bond, D. P. G.: Anoxic Annulata Events in the Late Famennian of the Holy Cross Mountains (Southern Poland): Geochemical and palaeontological record, Palaeogeogr. Palaeocl., 297, 549–575, https://doi.org/10.1016/j.palaeo.2010.08.028, 2010.
Racki, G. and Szulczewski, M.: Stop 4. Kowala railroad cut and quarry, in: Sixth European conodont symposium (ECOS VI), edited by: Szulczewski, M. and Skompski, S., Inst. Paleobiol. PAN, Inst. Geol. Podst., Warsaw, 1986, excursion guide, 27–30, ISBN 8301098600, 1996.
Racki, G., Racka, M., Matyja, H., and Devleeschouwer, X.: The Frasnian/Famennian boundary interval in the South Polish-Moravian shelf basins: Integrated event-stratigraphical approach, Palaeogeogr. Palaeocl., 181, 251–297, https://doi.org/10.1016/S0031-0182(01)00481-3, 2002.
Rakociński, M., Pisarzowska, A., Janiszewska, K., and Szrek, P.: Depositional conditions during the Lower Kellwasser Event (Late Frasnian) in the deep-shelf Łysogóry Basin of the Holy Cross Mountains Poland, Lethaia, 49, 571–590, https://doi.org/10.1111/let.12167, 2016.
Renaud, S. and Girard, C.: Strategies of survival during extreme environmental perturbations: Evolution of conodonts in response to the Kellwasser crisis (upper devonian), Palaeogeogr. Palaeocl., 146, https://doi.org/10.1016/S0031-0182(98)00138-2, 1999.
Renaud, S., Ecalle, B., Claisse, P., Charruault, A. L., Ledevin, R., and Girard, C.: Patterns of bilateral asymmetry and allometry in Late Devonian Polygnathus conodonts, Palaeontology, 64, 137–159, https://doi.org/10.1111/pala.12513, 2021.
Rigo, M., Mazza, M., Karádi, V., and Nicora, A.: New Upper Triassic Conodont Biozonation of the Tethyan Realm, edited by: Tanner, L.,pringer Cham, 189–235, https://doi.org/10.1007/978-3-319-68009-5_6, 2018.
Rohlf, F. J.: The tps series of software, Hystrix, 26, 9–12, https://doi.org/10.4404/hystrix-26.1-11264, 2015.
Rose, K. D. and Bown, T. M.: Gradual evolution and species discrimination in the fossil record, in: Vertebrates, phylogeny and philosophy, edited by: Flanagan, K. M. and Lillegraven, J. A., University of Wyoming, 119–130, https://doi.org/10.2113/gsrocky.24.special_paper_3.119, 1986.
Sandberg, C. A. and Ziegler, W.: Taxonomy and biofacies of important conodonts of Late Devonian styriacus Zone, United States and Germany, Geol. Palaeontol., 13, 173–212, 1979.
Sandberg, C. A. and Ziegler, W.: Devonian conodont biochronology in geologic time calibration, Senckenbergiana Lethaea, 76, 259–265, https://doi.org/10.1007/BF03042852, 1996.
Sandberg, C. A., Streel, M., and Scott, R. A.: Comparison between conodont zonation and spore assemblages at the Devonian-Carboniferous boundary in the western and central United States and in Europe, in: Congres International de Stratigraphie et de Géologie du Carbonifère, 1971, Krefeld, 1972.
Schäfer, W.: Einige neue Conodonten aus dem höheren Oberdevon des Sauerlandes (Rheinisches Schiefergebirge), Geol. Palaeontol., 10, 141–152, 1976.
Schmidt, H.: Conodonten-Funde in ursprünglichem Zusammenhang, Palaeontol. Z., 16, 76–85, https://doi.org/10.1007/BF03041668, 1934.
Schmidt, H. and Müller, K. J.: Weitere Funde von Conodonten-Gruppen aus dem oberen Karbon des Sauerlandes, Paläontol. Z., 38, 105–135, https://doi.org/10.1007/BF02988842, 1964.
Schneider, C. A., Rasband, W. S., and Eliceiri, K. W.: NIH Image to ImageJ: 25 years of image analysis, 9, 671–675, https://doi.org/10.1038/nmeth.2089, 2012.
Scott, H. W.: The zoological relationships of the conodonts, J. Paleontol., 8, 448–455, 1934.
Sendino, C., Broda, K., and Zatoń, M.: First record of true conulariids from the Upper Devonian of Poland, Proc. Geol. Assoc., 128, 401–406, https://doi.org/10.1016/j.pgeola.2017.03.004, 2017.
Shirley, B., Grohganz, M., Bestmann, M., and Jarochowska, E.: Wear, tear and systematic repair: Testing models of growth dynamics in conodonts with high-resolution imaging, P. R. Soc. B, 285, 20181614, https://doi.org/10.1098/rspb.2018.1614, 2018.
Shohel, M., Ray, K. K., Tivanski, A. V., McAdams, N. E. B., Bancroft, A. M., Cramer, B. D., and Forbes, T. Z.: Nanomechanical variability in the early evolution of vertebrate dentition, Sci. Rep., 12, 10203, https://doi.org/10.1038/s41598-022-14157-2, 2022.
Shunxin, Z., Aldridge, R. J., and Donoghue, P. C. J.: An Early Triassic conodont with periodic growth?, J. Micropalaeontol., 16, 65–72, https://doi.org/10.1144/jm.16.1.65, 1997.
Silcox, M. T.: A pragmatic approach to the species problem from a paleontological perspective, Evol. Anthropol., 23, 24–26, https://doi.org/10.1002/evan.21386, 2014.
Silcox, M. T., Selig, K. R., Bown, T. M., Chew, A. E., and Rose, K. D.: Cladogenesis and replacement in the fossil record of Microsyopidae (?Primates) from the southern Bighorn Basin, Wyoming, Biol. Lett., 17, 20200824, https://doi.org/10.1098/rsbl.2020.0824, 2021.
Smith, M. M., Sansom, I. J., and Smith, M. P.: “TEETH” BEFORE ARMOUR: THE EARLIEST VERTEBRATE MINERALIZED TISSUES, I I Mod. Geol., 20, 303–319, 1996.
Souquet, L., Guenser, P., Girard, C., Mazza, M., Rigo, M., and Goudemand, N.: Temperature-driven heterochrony as a main evolutionary response to climate changes in conodonts, P. R. Soc. B, 289, 20220614, https://doi.org/10.1098/rspb.2022.0614, 2022.
Spalletta, C., Perri, M. C., Jeffrey Over, D., and Corradini, C.: Famennian (Upper devonian) conodont zonation: Revised global standard, B. Geosci., 92, 31–57, https://doi.org/10.3140/bull.geosci.1623, 2017.
Spalletta, C., Corradini, C., Feist, R., Korn, D., Kumpan, T., Perri, M. C., Pondrelli, M., and Venturini, C.: The Devonian–Carboniferous boundary in the Carnic Alps (Austria and Italy), Palaeobio. Palaeoenv., 101, 487–505, https://doi.org/10.1007/s12549-019-00413-3, 2021.
Sweet, W. C. and Donoghue, P. C. J.: Conodonts: Past, present, future, J. Paleontol., 75, 1174–1184, https://doi.org/10.1017/S0022336000017224, 2001.
Świś, P.: Population dynamics of the Late Devonian conodont Alternognathus calibrated in days, Hist. Biol., 31, 1161–1169, https://doi.org/10.1080/08912963.2018.1427088, 2019.
Świś, P.: A new Devonian species of the enigmatic Carboniferous conodont Dollymae, Palaeoworld, 31, 86–92, https://doi.org/10.1016/j.palwor.2021.03.003, 2022.
Świś, P.: Anagenetic evolution and peramorphosis of a latest Devonian conodont from Holy Cross Mountain (Poland), OSF [data set], https://doi.org/10.17605/OSF.IO/JNHT7, 2023.
Świś, P. and Dzik, J.: The oral apparatus composition of the Early Carboniferous elictognathid conodont “Siphonodella”, Lethaia, 54, 341–353, https://doi.org/10.1111/let.12406, 2021.
Świś, P., Broda, K., Duda, P., Dróżdż, D., and Łazuka, A.: The early Famennian (Late Devonian) conodonts natural assemblages from Holy Cross Mountains (Poland), Hist. Biol., 33, 1565–1572, https://doi.org/10.1080/08912963.2020.1719085, 2020.
Szulczewski, M.: Upper Devonian conodonts, stratigraphy and facial development in the Holy Cross Mts, Acta Geol. Pol., 21, 129 pp., 1971.
Terrill, D. F., Jarochowska, E., Henderson, C. M., Shirley, B., and Bremer, O.: and ratios support trophic partitioning within a Silurian conodont community from Gotland, Sweden, Paleobiology, 48, 1–21, 2022.
Trotter, J. A., Williams, I. S., Barnes, C. R., Lécuyer, C., and Nicoll, R. S.: Did cooling oceans trigger Ordovician biodiversification? Evidence from conodont thermometry, Science, 321, 550–554, https://doi.org/10.1126/science.1155814, 2008.
Ulrich, E. O. and Bassler, R. S.: A classification of the toothlike fossils, conodonts, with descriptions of American Devonian and Mississippian species, Proc. United States Natl. Museum, 68, 1–63, 1926.
Wei, K. Y.: Stratophenetic Tracing of Phylogeny Using SIMCA Pattern Recognition Technique: A Case Study of the Late Neogene Planktic Foraminifera Globoconella Clade, Paleobiology, 20, 52–65, https://doi.org/10.1017/S0094837300011131, 1994.
Zatoń, M., Filipiak, P., Rakociński, M., and Krawczyński, W.: Kowala Lagerstätte: Late Devonian arthropods and non-biomineralized algae from Poland, Lethaia, 47, 352–364, https://doi.org/10.1111/let.12062, 2014.
Zaton, M., Broda, K., Qvarnström, M., Niedźwiedzki, G., and Ahlberg, P. E.: The first direct evidence of a late devonian coelacanth fish feeding on conodont animals, Sci. Nat., 104, 26, https://doi.org/10.1007/s00114-017-1455-7, 2017.
Zhuravlev, A. V.: Trophic position of some late devonian-carboniferous (Mississippian) conodonts revealed on carbon organic matter isotope signatures: A case study of the east european basin, Geodiversitas, 42, 443–453, https://doi.org/10.5252/geodiversitas2020v42a24, 2020.
Ziegler, W.: Taxionomie und Phylogenie Oberdevonischer Conodonten und ihre stratigraphische Bedeutung, Abhandlunghen des Hess. Landesamt für Bodenforsch., 38, 1–166, 1962.
Ziegler, W. and Sandberg, C. A.: Palmatolepis-6aW revision of upper part of standard late Devonian conodont zonation, Spec. Pap. Geol. Soc. Am., 196, 179–194, https://doi.org/10.1130/SPE196-p179, 1984.
- Abstract
- Introduction
- Geological settings
- Materials and methods
- Results of P1 morphology
- Interpretation of general trends in the evolution of P1
- Example of peramorphosis in D. marburgensis → D. trigonica lineage
- Morphotypes within the population variability
- To reconcile anagenesis and biostratigraphy
- Conclusions
- Data availability
- Sample availability
- Competing interests
- Disclaimer
- Acknowledgements
- Review statement
- References
- Abstract
- Introduction
- Geological settings
- Materials and methods
- Results of P1 morphology
- Interpretation of general trends in the evolution of P1
- Example of peramorphosis in D. marburgensis → D. trigonica lineage
- Morphotypes within the population variability
- To reconcile anagenesis and biostratigraphy
- Conclusions
- Data availability
- Sample availability
- Competing interests
- Disclaimer
- Acknowledgements
- Review statement
- References