the Creative Commons Attribution 4.0 License.
the Creative Commons Attribution 4.0 License.
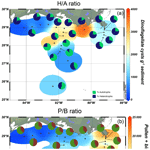
Dinoflagellate cyst and pollen assemblages as tracers for marine productivity and river input in the northern Gulf of Mexico
Timme Donders
Francien Peterse
Francesca Sangiorgi
Both marine dinoflagellate cysts and terrestrially derived pollen and spores are abundant in coastal sediments close to river mouths, making sediment records from such settings ideal to simultaneously study land–ocean climate interactions, marine productivity patterns and freshwater input over time. However, few studies consider the combined calibration of these palynological proxies in modern coastal sediments offshore from rivers, which is needed to strengthen the interpretation of paleoreconstructions. Here, we analyze the palynological content of marine surface sediments along land–sea transects off the Mississippi and Atchafalaya river mouths in the northern Gulf of Mexico (GoM) and test three palynological indices which are often employed in the paleo-domain: (i) the abundance of cysts of heterotrophic and autotrophic dinoflagellates (dinocysts) as a tracer for primary productivity ( ratio) and (ii) the ratio between non-bisaccate pollen and bisaccates () as well as (iii) the ratio between pollen (excluding bisaccates) and dinocysts (), which are both tracers for river input and distance to the coast.
Our results show that dinoflagellate cysts are most abundant on the shelf, where heterotrophic dinocyst species dominate coastal assemblages in reach of the river plume, while autotrophic taxa are more present in the oligotrophic open ocean. This is clearly reflected in decreasing values further offshore. Individual dinocyst taxa also seem to inhabit specific niches along an onshore–offshore transect, linked to nutrient availability and proximity to the turbid river plume. The highest pollen concentrations are found close to the Mississippi river mouth and mostly represent a mixture of local coastal and upstream vegetation, whereas bisaccate pollen was most abundant further offshore of the Mississippi river. Multivariate redundancy analysis (RDA) performed on both pollen and dinocyst assemblages, a set of environmental parameters, and the three palynological ratios showed that net primary productivity was the most important variable influencing the dinocyst assemblages, likely as the result of nutrient input. Additionally, the RDA confirmed that the ratio indeed seems to track primary productivity, while the ratio results in a robust indicator for distance to the coast, and the ratio better reflects river input. Together, our data confirm and further specify the suitability of these three palynological ratios in river-dominated coastal margins as proxies for (past) marine productivity and distance to the coast and river.
- Article
(7202 KB) - Full-text XML
- BibTeX
- EndNote
Palynology involves the study of organic-walled aquatic (e.g., dinoflagellate cysts, green algae, tintinnids, copepod eggs, foraminifer linings) and terrestrial (primarily pollen, spores, fungi) remains and is a powerful tool in paleoclimate reconstructions (Dale, 1996; McCarthy and Mudie, 1998; Pross and Brinkhuis, 2005; de Vernal, 2009; Birks, 2019). Coastal sediments usually contain both dinoflagellate cysts and pollen in sufficient amounts to allow simultaneous investigation of (past) climatic and environmental changes in both the marine and terrestrial realms (Sangiorgi and Donders, 2004; Donders et al., 2018; Mudie et al., 2021). About ∼ 15 % of the dinoflagellates, one of the major groups of primary producers in modern oceans (Hoppenrath et al., 2009), form organic-walled cysts (dinocysts) during their lifecycle, which can be preserved in sediments for millions of years (Head, 1996). Since dinoflagellates are distributed in the upper water column according to environmental parameters such as salinity, temperature, nutrients, and productivity as well as the presence of sea ice, their cysts occupy distinct niches in the modern ocean such as upwelling systems, lagoonal environments, sea-ice-dominated zones, and oligotrophic open ocean waters (Rochon et al., 1999; Zonneveld et al., 2013; Marret et al., 2019; de Vernal et al., 2020; Thöle et al., 2023). Furthermore, dinoflagellate taxa have different feeding strategies (autotrophic, heterotrophic, and mixotrophic; Jeong et al., 2010). While autotrophs produce energy by photosynthesis, heterotrophs are zooplankton that graze on diatoms, bacteria, and other dinoflagellates (Jacobson and Anderson, 1986). Therefore, cysts of autotrophic and heterotrophic dinoflagellates have been used to investigate nutrient availability, annual productivity, and (cultural) eutrophication in the present and in the past (Sangiorgi and Donders, 2004; Radi and de Vernal, 2008; Dale, 2009; Zonneveld et al., 2009; Limoges et al., 2013).
Similarly, coastal sediments contain abundant pollen and spores that are derived from terrestrial higher plants and are transported to the marine environment via wind and rivers (Mudie and McCarthy, 1994; Cohen et al., 2014). Several studies have shown that pollen assemblages from marine sediments provide an accurate representation of the regional flora (Muller, 1959; Groot and Groot, 1966; Mudie, 1982; Hooghiemstra, 1988), and therefore, pollen stored in marine sediment cores has been used to reconstruct past vegetation changes (e.g., Mudie and McCarthy, 1994; Hooghiemstra et al., 2006; Zwiep et al., 2018). In river-dominated coastal areas, fluvial transport acts as the main input of pollen and spores, resulting in high pollen concentrations and richer assemblages close to the river mouth (Heusser, 1978; Beaudouin et al., 2007). However, rivers can transport pollen from throughout their drainage basin and thus likely carry a mixed signal of several upstream sources that can potentially overprint local, near-shore vegetation signals (Chmura et al., 1999). Changes in the provenance of pollen may thus partially alter the reliability of paleoreconstructions based on pollen assemblages in river-dominated continental-margin sediments, although this still allows the use of pollen as an indication of terrestrial and/or riverine input (Heusser, 1978; Chmura and Liu, 1990).
Several ratios based on pollen and/or dinocysts have been applied to coastal marine sediment archives to reconstruct marine productivity and terrestrial input (Iria et al., 2023). For example, the ratio of heterotrophic dinocysts over autotroph dinocysts ( ratio) has been used to reconstruct paleoproductivity, based on the observation that heterotrophic taxa are often more abundant in nutrient-rich waters (Bujak, 1984; McCarthy and Mudie, 1998; Pieńkowski et al., 2011; van Helmond et al., 2015; Zwiep et al., 2018). However, since heterotrophic and autotrophic dinocysts have different preservation potentials, this ratio should be carefully examined – especially in low-oxygen zones, where the proxy can be influenced by this preservation bias (Zonneveld et al., 2001; Versteegh et al., 2010). Next, the ratio of non-bisaccate pollen over bisaccate pollen ( ratio) utilizes the assumption that bisaccate pollen (mainly derived from the genus Pinus) is relatively more abundant offshore in marine environments – compared to other morphological pollen types – as a result of its higher susceptibility to airborne transport (Mudie, 1982; Heusser, 1988), also known as the Neves effect (Chaloner and Muir, 1968). Since non-bisaccate pollen is mainly fluvially transported in river-dominated coastal zones, its abundance usually decreases further offshore (Muller, 1959; Heusser, 1983; Rochon and Vernal, 1994). Therefore, the ratio can be used to reconstruct distance from the coast and/or river. Similarly, the ratio between pollen grains and dinocysts ( ratio) can trace river input and has also been used as an indicator for terrestrial input, distance from the coast, and relative sea level change (McCarthy and Mudie, 1998; Munoz Sobrino et al., 2012; Li et al., 2017; Donders et al., 2018). For example, the ratio has been used as an indication of river input through the Quaternary (e.g., McCarthy and Mudie, 1998; Leroy et al., 2007; Mudie et al., 2021). The use of this ratio as an indicator for terrestrial influence was justified by a study that showed a decrease in pollen vs. dinocyst ratio offshore from the St. Lawrence Estuary, coinciding with an increase in δ13Corg, indicating the shift in organic matter source from terrestrial to marine along the transect (De Vernal et al., 1991). However, the use of a modern calibration dataset that tests these indices is still limited, especially in river-dominated coastal margins along a land–sea transect (Coussin et al., 2022; Iria et al., 2023). It is, therefore, important to understand the occurrence and spatial distribution of pollen and dinocysts in the modern environment.
Here, we aim to test the applicability of these ratios in the northern Gulf of Mexico, a river-dominated continental margin fed by the Mississippi–Atchafalaya river system (MAR). The MAR transports large amounts of terrestrial organic matter and nutrients, in turn fueling primary production on the Louisiana shelf (Lohrenz et al., 1990, 1999; Rabalais et al., 2007). The northern Gulf of Mexico (GoM) hosts many specific microenvironments, as it is characterized by freshwater, nutrient, and suspended-sediment input from the MAR, as well as the influence of warm, saline waters from the Loop Current. Previous studies of this area report high dinocyst diversity and have also labeled the GoM as a glacial refugium for species previously thought extinct, such as Melitasphaeridium choanophorum (Limoges et al., 2013; Price et al., 2017). The presence of both pollen and dinocysts in the sediments of the northern GoM makes this location ideal to study the palynological proxies based on these palynomorphs. Both modern pollen and dinocyst assemblages have previously been studied in the northern Gulf of Mexico (Chmura and Liu, 1990; Chmura et al., 1999; Edwards and Willard, 2001; Limoges et al., 2013, 2014; Price et al., 2017, 2018; Yedema et al., 2023), although most of these studies have focused on only one group of palynomorphs, i.e., either pollen or dinocysts, and thus lack the combined approach that can strengthen paleoreconstructions by linking environmental changes on land and in the ocean. For example, Limoges et al. (2013) report concentrations of both palynomorphs but do not differentiate between pollen taxa, while the work by Edwards and Willard (2001) also includes both but is limited to the shallow waters of the Mississippi Sound and therefore lacks the offshore aspect to test these proxies. Therefore, we analyzed here the palynological content of marine surface sediments along two land–sea (coast to offshore) transects and one longitudinal transect following the MAR plume west along the coast of the northern GoM (Fig. 1). We then tested the suitability of the aforementioned palynological ratios (, , ) as (paleo)proxies for marine productivity, river influence, and coastline variability induced by sea level fluctuations by performing a redundancy analysis on the palynological ratios, the dinocyst and pollen assemblages, and a suite of environmental parameters. Table 1 reports the list of frequently used abbreviations to facilitate readability of the text.
2.1 Study site
The northern GoM is heavily influenced by freshwater, nutrient, and sediment inputs from the MAR, which cover a discharge area of 3.3 × 106 km2 (Milliman and Syvitski, 1992). These rivers discharge on average 22 400 m3 of water per second into the northern GoM, from which 70 % is directed through the Mississippi river (MR) channel, while 30 % of the outflow enters the GoM via the Atchafalaya river (AR; Reuss, 2004). Upon discharge, surface winds generally direct the MR plume westwards along the Louisiana shelf, while storm events or cold fronts can cause offshore dispersal of plume waters (Cochrane and Kelly, 1986; Walker et al., 2005). The size of the sediment plume is dependent on river discharge and covers 92 km2 during low river discharge while reaching an extent of > 2700 km2 during high river discharge in spring (Walker et al., 2005). During easterly winds, the river plume generally reaches until the shelf break (100 m isobar), while enhanced offshore transport of shelf waters occurs during southwesterly winds (Schiller et al., 2011). Here, circulation from the Loop Current, which carries warm waters from the Caribbean, disperses the plume waters further offshore.
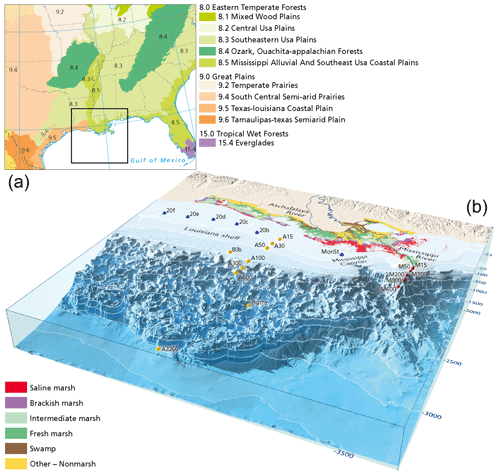
Figure 1(a) Ecoregions in the lower Mississippi basin. Main forest types along the Mississippi river include oak–hickory (Quercus–Carya) forests, loblolly–shortleaf pine (Pinus taeda, P. echinate) forests, and longleaf–slash pine vegetation (P. palustris, P. elliottii). Ecoregions were adapted from Omernik and Griffith (2014) and the National Forest Type Dataset (USDA). (b) Locations of the surface sediments collected from the northern Gulf of Mexico. Sediments were retrieved from three transects: the Mississippi transect (red dots), the Atchafalaya transect (yellow dots), and a transect along the Louisiana shelf (blue dots). Vegetation types of coastal Louisiana derived from Nyman et al. (2021).
The MAR carries nutrient-rich waters, which upon discharge fuel algal blooms, eventually leading to the formation of a seasonal hypoxic zone (Rabalais et al., 2002). This process was accelerated in the last decades in response to increased nutrient use in agriculture (Tian et al., 2020). The hypoxic zone (< 2 mg L−1 oxygen concentrations), which forms during summer months, has been monitored since the 1970s and has put major ecologic and economic stress on the area (Rabalais and Turner, 2019). Due to the warm, nutrient-rich waters, primary productivity is high on the shelf (Lohrenz et al., 1999), where also high abundances of dinocysts are found (Price et al., 2018; Yedema et al., 2023).
Vegetation in the MAR catchment is diverse and includes vast grasslands and hardwood forests (e.g., Delcourt and Delcourt, 1984, 1996; Barbour and Billings, 2000). The terrestrial coastal zone of Louisiana is characterized by extensive wetlands (Coleman et al., 1998), where freshwater to saline swamps and marshes occur (Fig. 1). These swamps and marshes are typically characterized by vegetation like cypress (Taxodium), cattail (Typha), grasses (Poaceae and Cladium and other Cyperaceae), willows (Salix), tupelo (Nyssa), herbs like Amaranthaceae and Asteraceae and other swamp and marsh taxa. Along the shore, red mangrove (Rhizophora) and saltwort (Batis) taxa are also present. In the last decades, coastal erosion, sea level transgressions, hurricane activity, and human activities have exerted more stress on the rapidly declining wetland areas and caused a gradual change from fresh to intermediate and brackish marshes (Couvillion et al., 2011). More inland, forests are more abundant, and vegetation includes Pinus, Quercus, Carya, Liquidambar, Morella, Morus, Betula, Acer, and Ulmus.
2.2 Sediment collection
Sediment samples were collected during a cruise to the northern Gulf of Mexico with the RV Pelagia in February 2020. In total, 21 multicores containing the sediment–water interface were retrieved, along land–sea transects from the Mississippi (15–600 m water depth) and Atchafalaya rivers (15–3200 m water depth) and a cross-shelf transect on the Louisiana shelf (20 m water depth) following the river plume. During sampling, on-board O2 micro-profiling revealed bottom water oxygen concentrations of 40–100 mg L−1 for all sites. Station names for the MR and AR transects refer to their transect and the water depth (Fig. 1). Similarly, the station names of the shelf transect also indicate the water depth. Sediments were subsampled in 0.5–1 cm slices on board and stored at 4 ∘C before palynological processing. Surface sediments used for this study represent the upper 2 cm. 210Pb analysis for the 80b and M100 multicores indicate sedimentation rates on the order of 0.5–1 cm yr−1 for the continental shelf and river mouth samples (Yedema et al., 2023).
2.3 Palynological analysis
Surface sediments (∼ 20 g dry weight) were processed following the standard palynological technique and method of Wood et al. (1996). A tablet of Lycopodium clavatum spores (containing 19 866 spores) was added to the samples prior to processing, enabling the calculation of the palynomorph concentrations according to Benninghoff (1962). Samples were treated with 10 % and 30 % HCl and 40 % cold HF to dissolve carbonates and silicates, respectively. Coarse and fine fractions were removed by sieving with a 250 and 10 µm mesh. The residues were mixed with glycerin jelly and mounted on a microscope slide. Dinocyst and pollen (excluding bisaccates) counts contained 116–245 (median 202) and 186–300 (median 256) specimens per sample and were counted using a light microscope with 400× magnification. Taxonomy follows Williams et al. (2017), and dinocysts were identified using Rochon et al. (1999) and Zonneveld and Pospelova (2015). Pollen and spore identification was done using the atlas of Lieux (1980) and Willard et al. (2004). Juniperus and Taxodium, both part of the Cupressaceae family, were distinguished based on the presence of a ligula in Taxodium pollen. Since Pinus pollen is often dominant in pollen assemblages and is more likely to be transported via wind (Rochon and Vernal, 1994; Mudie and McCarthy, 1994), it has not been included in the total pollen sum nor in the ratio. Relative abundances are calculated from the total dinocyst and pollen sums, while absolute abundances are presented in cysts and pollen per gram sediment. The ratio is calculated as the total number of cysts produced by heterotrophic dinoflagellates (H) divided by the total dinocysts counted (H+A), i.e., as a percentage of heterotrophic cysts in the assemblage. Similarly, the is calculated as a percentage of the total quantity of non-bisaccate pollen and spores (P) divided by the total pollen (P+B), while the ratio is defined as the total quantity of non-bisaccate pollen and spores (P) divided by the total dinocyst count (D) (in percent). The slides are stored at the slide repository of MPP Utrecht.
2.4 Environmental variables and statistical analysis
Data on the sea surface temperature (SST), sea surface salinity (SSS), surface dissolved oxygen (DO), and nutrient concentrations (nitrate, phosphate, silicate) were obtained from the World Ocean Atlas 2018 (Zweng et al., 2019; Garcia et al., 2019a, b) for both annual and seasonal timescales. Net primary productivity (NPP) data were downloaded from the Oregon State University website for the period of 2010–2020 (http://orca.science.oregonstate.edu/2160.by.4320.monthly.xyz.vgpm.m.chl.m.sst.php, last access: 21 November 2023). Here, primary productivity is calculated using the vertical generalized production model (VGPM) developed by Behrenfeld and Falkowski (1997), which uses chlorophyll a data from NASA's Moderate Resolution Imaging Spectroradiometer (MODIS) program. Geochemical data (total organic carbon, TOC; δ13Corg; ratio) were derived from Yedema et al. (2023). Distance to the coast and Mississippi and Atchafalaya rivers was calculated in kilometers using Google Earth Pro software. Water depth was measured on board during the cruise.
We performed multivariate analysis using the R vegan package 2.6–4 (Oksanen et al., 2013) to interpret the species distributions in the dataset. Pollen and dinocyst species that reached > 3 % and > 5 % of the total assemblage, respectively, as well as the palynological ratios were considered for analysis. The species data were first transformed by performing a square-root transformation (Legendre and Birks, 2012). A preliminary detrended correspondence analysis (DCA) resulted in a first gradient of < 1 SD (Hill and Gauch, 1980), justifying the use of a redundancy analysis (RDA) for the constrained ordination. All environmental variables were log-transformed to ensure they show a unimodal distribution.
3.1 Palynological assemblages
3.1.1 Marine assemblages
Surface sediments of the northern GoM contained well-preserved marine and terrestrial palynomorphs. Besides dinocysts, pollen, and spores, several other palynomorphs including foraminifera lining, copepod eggs, Concentricystes, and green algae like Pediastrum and Botryococcus were found. Most of these palynomorphs were present in low abundances except for the foraminifer linings and copepod eggs, which reached the highest abundances along the Atchafalaya transect. The total concentration of dinocysts ranged from 230–3600 cysts g−1 and was highest on the Louisiana shelf (Fig. 2a). Close to shore, assemblages were characterized by high amounts of the heterotrophic taxa Brigantedinium spp. (up to 47 %), cysts of Polykrikos spp. (0 %–38 %), Echinidinium spp., (2 %–13 %), cysts of Archaeperidinium minutum (0 %–11 %), and Selenopemphix quanta (0 %–9 %) (see Table 2 and Fig. A1). Dominant autotrophic taxa near the MR mouth include Lingulodinium machaerophorum (0 %–12 %) and diverse Spiniferites species (including S. ramosus, S. hyperacanthus, and S. mirabilis; 2 %–10 %). Other taxa that reach abundances > 5 % here include Polysphaeridium zoharyi, Dapsilidinium pastielsii, Gymnodinium microreticulatum, Lejeunecysta sabrina, and Trinovantedinium pallidifulvum (cysts of Protoperidinium pallidifulvum; Mertens et al., 2017).
Table 2List of selected (> 5 %) dinoflagellate taxa and their abbreviations used in the RDA. Their trophic level (A: autotrophic; H: heterotrophic) and their occurrence in the number of samples as a percentage are listed.
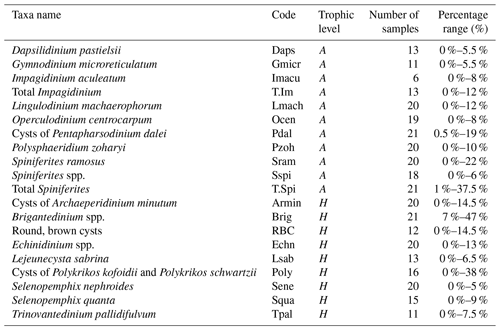
Further offshore, dinocyst concentrations decreased to 600 cysts g−1 at A600 (Fig. 1) and were dominated by autotrophic species: Spiniferites species (7 %–37 %, mainly S. ramosus), cysts of Pentapharsodinium dalei (1 %–19 %), Impagidinium species (1 %–12 %, mainly I. aculeatum and I. striatum), L. machaerophorum (1 %–10 %), Operculodinium centrocarpum (0 %–8 %), and P. zoharyi (0 %–10 %) (Fig. 3). Brigantedinium spp. was also abundant here (7 %–39 %). At the westernmost shelf (site 20f), dinocyst concentrations were the lowest (230 cysts g−1), and the assemblages were characterized by high amounts of Selenopemphix spp.; Brigantedinium spp.; T. pallidifulvum; and round, brown cysts that were often folded and degraded. Furthermore, low occurrences of Melitasphaeridium choanophorum were found throughout the transects, without a clear spatial trend. An overview of dinocyst percentages is listed in Fig. A1.
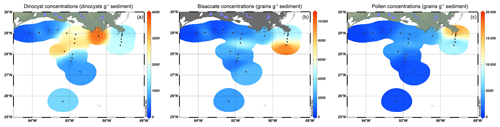
Figure 2Isosurface plots showing concentrations (cysts or grains g−1) of (a) dinoflagellate cysts, (b) bisaccate pollen, and (c) non-bisaccate pollen in the northern Gulf of Mexico.
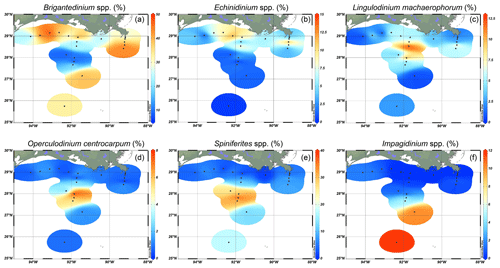
Figure 3Relative abundances of selected dinocysts, indicating their preferred niches along a land–sea transect. (a) Brigantedinium spp., (b) Echinidinium spp., (c) Lingulodinium machaerophorum, (d) Operculodinium centrocarpum, (e) Spiniferites spp. (including S. ramosus, S. hyperacanthus, and S. mirabilis), and (f) Impagidinium spp. (including I. aculateum and I. striatum).
3.1.2 Terrestrial assemblages
The pollen assemblages were mainly dominated by Pinus pollen, which had the highest absolute abundances in the deeper waters of the MR transect (Fig. 2b). Total pollen concentrations, including bisaccate pollen, ranged from 750 grains g−1 at the western shelf to 9500–25 000 grains g−1 along the MR transect. Non-bisaccate pollen concentrations showed a decreasing trend with the highest concentrations near the MR mouth (20 000 grains g−1) and lowest concentrations in deeper waters (∼ 940 grains g−1 at site A3200; Fig. 2c). The relative abundances of pollen taxa vary spatially (Fig. 4). Of the non-bisaccate pollen, Quercus was most abundant in the assemblages (21 %–41 %). Close to shore, the main pollen taxa were Amaranthaceae (5 %–16 %), Asteraceae subf. Asteroideae (5 %–11 %), Poaceae (3 %–7 %), Cyperaceae (1 %–6 %), Ambrosia (2 %–7 %), Juniperus (1 %–6 %), Taxodium (1 %–5 %), Carya (1 %–5 %), Typha (1 %–5 %), and Ulmus (0 %–4 %) (Table 3). The offshore assemblages also contain abundant Quercus (22 %–40 %), as well as Cyperaceae (7 %–11 %), Amaranthaceae (2 %–10 %), Poaceae (2 %–8 %), Asteraceae subf. Asteroideae (2 %–6 %), Juniperus (1 %–6 %), Taxus (1 %–4 %), Typha (0 %–4 %), and high abundances of fern spores (2 %–19 %). Taxa that generally occur in upland or montane regions of the US are grouped together in Fig. 4e and include Abies, Picea, Tsuga, Taxus, and Tilia. Other taxa that occur in smaller (≤ 3 %) abundances in the northern GoM include Betula, Alnus, Fraxinus, Liquidambar, Salix, Artemisia, Morella, and Nyssa (Fig. A2).
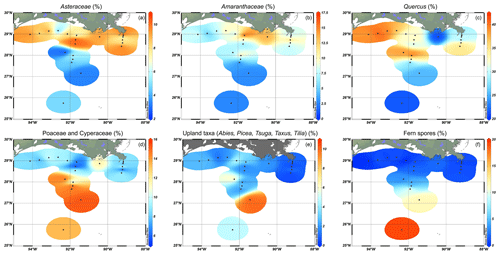
Figure 4Relative abundances of selected pollen, indicating their distributions in the northern Gulf of Mexico. (a) Asteraceae, including mainly Asteraceae subf. Asteroideae with small abundances of Asteraceae subf. Cichorioideae; (b) Amaranthaceae; (c) Quercus; (d) Poaceae and Cyperaceae; (e) upland taxa, which includes Abies, Picea, Tsuga, Taxus, and Tilia; and (f) fern spores (trilete and monolete spores).
Table 3List of selected (> 3 %) pollen and spores and their abbreviations used in the RDA. Their vegetation group and occurrence in the number of samples as a percentage are listed. Groups are based on the prevalent habitat of taxa in the coastal zone (see Fig. 1).
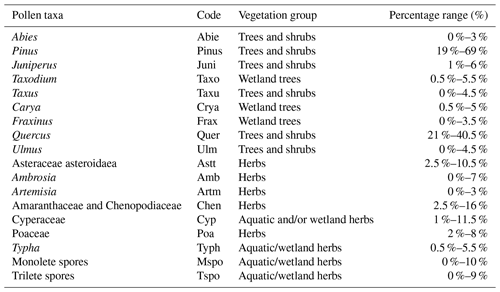
The spatial distribution of dinocysts and pollen is captured in the palynological ratios (Fig. 5). On the shelf and close to the river mouths, heterotrophic dinocyst taxa dominated the assemblages, while autotrophic taxa were dominant further offshore. This is reflected in the ratio, with the highest values on the shelf (64 %–83 %) and along the MR transect (64 %–77%; Fig. 5a). The ratio decreases offshore from the AR transect to 43 %, with the lowest values found on the shelf break (13 %). The ratio (Fig. 5b) decreases from 81 %–63 % on the shelf, 70 %–39 % on the AR transect, and 81 %–45 % on the MR transect. The highest values were found near the mouths of the MAR, while the lowest values occurred towards the open ocean. Finally, the ratio decreased similarly (Fig. 5c). High values of 72 %–92 % were found along the MR transect, with a decreasing trend towards the shelf (71 %–53 %) and offshore from the AR transect (65 %–43 %).
3.2 Ordination analysis
An RDA was performed on the pollen and dinocyst assemblages, including the palynological ratios (Fig. 6). The constrained axes are representative of 91.9 % of the total variance, where the first two constrained RDA axes explain 44.9 % and 12.3 %, respectively. On RDA1, the net primary productivity has a negative score, as do the nutrient concentrations of nitrate, silicate, and phosphate; dissolved oxygen concentration; the summer sea surface temperature; and the sedimentary parameters δ13Corg and ratio. Distance from the coast, distance to both the Mississippi and Atchafalaya river mouths, water depth, annual sea surface salinity, annual and winter sea surface temperatures, and sediment TOC all plot positive on RDA1. Almost all the heterotrophic dinocysts plot with the NPP and nutrients, while most of the autotrophic dinocysts are associated with salinity, temperature, distance from the coast, and water depth. Most pollen taxa plot close to the center of the graph, except for Pinus and the monolete and trilete spores, which plot in the direction of water depth and distance from the coast. The ratio plots negative on RDA1, together with the NPP and phosphate concentration. Both the and ratios also plot negative on RDA1, opposite of the distance from the coast and water depth, where especially the ratio plots further away from the center of the graph. The ratio is separated from the and ratios on RDA2.
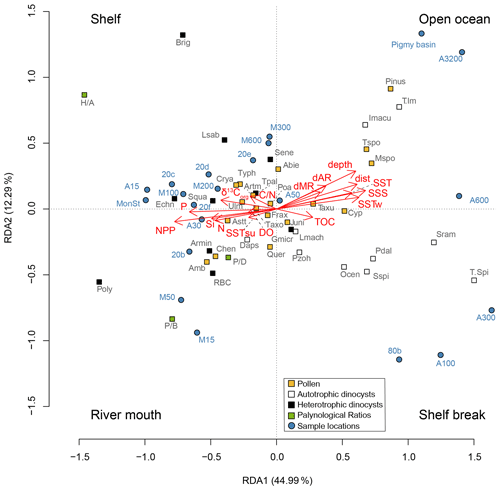
Figure 6Redundancy analysis on selected dinocysts and pollen, together with the palynological proxies. Dinocyst and pollen abbreviations are listed in Tables 2 and 3. Heterotrophic and autotrophic dinocysts are represented as black and white boxes, respectively. Log-transformed environmental variables represent depth: water depth (m); dAR: distance to the Atchafalaya river (km); dMR: distance to the Mississippi river (km); dist: distance to the coast (km); DO: dissolved oxygen concentration (µmol kg−1); NPP: net primary productivity (gC m−2 yr−1); N: nitrate concentration (µmol kg−1); P: phosphate concentration (µmol kg−1); Si: silicate concentration (µmol kg−1); SSS: annual sea surface salinity; SST, SSTsu, and SSTw: annual, summer and winter sea surface temperature (∘C); δ13Corg; ratio: carbon nitrogen ratio; TOC: total organic carbon. Environmental interpretations have been provided for the four quadrants.
4.1 Distributions of palynomorphs
4.1.1 Dinocyst distributions in the northern GoM
Our results indicate that heterotrophic taxa (i.e., Echinidinium spp., cysts of A. minutum, cysts of Polykrikos spp., and S. quanta) are generally more abundant along the shelf and MR transects, while autotrophic taxa dominate shelf break and open-ocean settings. Interestingly, within the autotrophic dinocyst assemblage, individual taxa also seem to inhabit specific niches along a land–sea gradient in the northern GoM (Fig. 3). For example, most autotrophic taxa (e.g., L. machaerophorum, P. zoharyi, O. centrocarpum, cysts of P. dalei, Spiniferites spp.) are relatively more abundant along the AR transect at 100–600 m water depth around the continental slope, while taxa from the genus Impagidinium are most abundant further offshore. Although L. machaerophorum is often linked to riverine nutrient input, here it occupies a niche on the edge of the nutrient-rich area, but outside the influence of the suspended sediment associated with the river plume. On the other hand, the occurrence of O. centrocarpum at 100–600 m depth along the AR transect fits with its supposed niche at the boundary between nutrient-rich coastal waters and oligotrophic open ocean waters (Dale, 1996).
The dinocyst assemblages found here are similar to those found in previous studies of the GoM, where heterotrophic taxa like Brigantedinium and cysts of Polykrikos and A. minutum were most abundant on the Louisiana shelf (Price et al., 2018), while high abundances of Impagidinium spp. (50 %), O. centrocarpum (20 %), and S. ramosus (>30 %) were found further offshore (Limoges et al., 2013). More east of the MR, sediments contained O. centrocarpum, L. machaerophorum, Spiniferites spp., P. zoharyi, and Brigantedinium spp. Sediments in the Mississippi Sound were mostly dominated by P. zoharyi (Edwards and Willard, 2001), a taxon commonly associated with lagoons and warm, euryhaline environments that has been attributed to enhanced influx of the Loop Current (Limoges et al., 2014). It also dominated assemblages in Mexican lagoons of the southern Caribbean (Limoges et al., 2013). Price et al. (2018) found that L. machaerophorum was the dominant autotrophic taxon on the Louisiana shelf, where high nutrient concentrations are present. However, it did not dominate the assemblages in this study, possibly due to unfavorable conditions caused by the freshwater and suspended-sediment discharge from the MAR.
The RDA results reflect specific niches for the dinocysts as well. Based on the scores of the sample locations, the RDA plot can be separated into four quadrants, in which shelf (0–100 m water depth), open ocean (> 500 m water depth), shelf break, and river mouth environments can be recognized (Fig. 6). Most heterotrophic taxa plot in the quadrants associated with the shelf or close to the Mississippi river mouth, and most autotrophic taxa are found in the quadrant representing the shelf break, while Impagidinium taxa show an affinity for open-ocean conditions. Forward-selection analysis on the environmental parameters showed that the most significant variable influencing the dinocyst and pollen distributions in the northern GoM was the net primary productivity, followed by sea surface salinity, winter SSTs, and distance to the coast. The same results were achieved when the RDA was performed on dinocysts separately (data not shown). RDA1, which explains most variation in the data (∼ 45 %), indicates that the dinocyst and pollen distributions are mostly controlled by a strong onshore–offshore gradient. It should be noted that most of the environmental parameters included in the RDA are (in)directly related, as both the water depth and SSS generally increase with increasing distance from the coast, while similarly, NPP is fueled by nutrient concentrations and is therefore collineated with those as well. Therefore, the onshore–offshore gradient here mostly reflects the contrast in high nutrient levels and primary productivity on the shelf and the increasing SSS and SST towards the open ocean.
Interestingly, the summer and winter sea surface temperatures plot differently on the RDA; where the winter SST closely resembles the annual SST, summer SST plots in the opposite direction. In summer, the SST gradient from land to sea is much less pronounced than in winter due to an extended formation of the Loop Current that transports warm, tropical waters further north (Sturges and Evans, 1983). In winter, the position of the Loop Current is more retracted, resulting in a stronger SST gradient due to a relatively higher influence of MAR that discharges cooler waters to the GoM. Temperature is often considered the most important factor controlling dinocyst distributions, also in the GoM (Limoges et al., 2013; Zonneveld et al., 2013). However, other environmental parameters may also be of major importance for the spatial distribution of dinocysts, as studies from the West African coast and the Mediterranean Sea reported nutrient levels and/or primary productivity as the main driver (Dale et al., 2002; Coussin et al., 2022). Similarly, a study on an Iberian estuary reported a strong inshore–offshore trend in the dinocyst distributions, with high chlorophyll a and sea surface nitrate concentrations inshore and high sea surface salinity and annual bottom oxygen saturation percentages further offshore (Iria et al., 2023). With this study, we confirm the presumed importance of primary productivity on the distribution of the dinocysts in the northern GoM, in addition to the onshore–offshore gradients in SSS and (winter) SSTs.
4.1.2 Pollen distributions in the northern GoM
The high concentrations of pollen close to the MR mouth indicate that the river is likely the primary source of pollen in the northern GoM as opposed to aeolian input but also that inputs from the AR and direct inputs from coastal vegetation seem less significant. In previous studies, pollen distributions from the GoM indeed closely mirrored pollen assemblages from downstream MR and thus provided a record of the Mississippi basin, while the assemblages of the AR represented more local vegetation input (Chmura et al., 1999). In our study, this observation is supported by the occasional presence of pollen from Abies, Picea, and Tsuga, which are taxa that strictly occur upland in the zone of the Mississippi headwaters, and their occurrence implies that the MR carries pollen from throughout its drainage basin to the GoM (Fig. 4e herein; Chmura and Liu, 1990). However, despite being low in total abundance, these upland taxa are most abundant near the Pigmy basin, instead of close to the MR mouth or in the plume direction (Fig. 4e). This can be explained by the fact that the Pigmy basin is a known accumulation site for terrestrial organic matter discharged by the Mississippi river (Jasper and Gagosian, 1990; Montero-Serrano et al., 2009), and consequently, high pollen concentrations were found here (Limoges et al., 2013). Therefore, the upland taxa found in the Pigmy basin could still be derived from the MR, while closer to the coast, inputs from local vegetation might have overprinted this upland pollen signal.
Several taxa including Pinus, Quercus, Salix, Fraxinus, Ulmus, and Ambrosia can represent both upstream and local sources, rendering it likely that some of this pollen is sourced from higher up in the MAR catchment. This pollen was abundantly found in the northern GoM, especially Pinus and Quercus pollen. A study by Chmura et al. (1999) reported similar trends, where the Louisiana shelf was typically characterized by high amounts of Pinus, TCT (i.e., Taxodiaceae, Cupressaceae, and Taxaceae family), Quercus, and Amaranthaceae pollen. In addition, pollen assemblages from the Mississippi Sound contained large amounts of Pinus and Quercus as well (Edwards and Willard, 2001), where Pinus pollen concentrations increased further offshore, while Quercus pollen did not show a spatial trend.
Pinus concentrations in the northern GoM increased especially along the MR transect, decreased along the shelf, and remained relatively stable along the AR transect (Fig. 2b). The relative abundance of Pinus compared to the total pollen sum increases seaward along all three transects; pine pollen represented ∼ 20 % of the total pollen sum close to the MR mouth but increased to 37 % on the shelf and 70 % in deeper waters (Fig. 5b), as is canon in the literature (Mudie, 1982; Mudie and McCarthy, 1994; Chmura et al., 1999; Luo et al., 2014; Dai et al., 2014).
Besides Pinus, fern spores also increase in abundance from 0 %–2 % close to the coast to ∼ 20 % of the pollen assemblage in offshore sediments (Fig. 4f), indicating the possibility that pollen grains are sorted during transport (Brush and Brush, 1972). A similar trend in fern spores has also been reported from the South China Sea (Sun et al., 1999; Luo et al., 2014; Dai et al., 2014). These spores might be carried long distances due to their low density and consequent high flotation ability, which improves their dispersal compared to other terrestrial palynomorphs (Heusser, 1988; Sun and Li, 1998; Sun et al., 1999; Li et al., 2017). Early experiments on the flotation ability of pollen have shown that Pinus and fern pollen is more likely to float compared to non-bisaccate pollen (Hopkins, 1950; Florin, 1963). This would facilitate its transport further offshore, although a more recent study found no evidence for a larger floating ability of Pinus pollen (Zheng et al., 2011). Moreover, a study monitoring conifer pollen transport showed that Pinus pollen was proportionally better represented in airborne samples than in rivers and local vegetation (Brown et al., 2007). The higher abundance of Pinus pollen further offshore can thus be the result of wind transport and offshore currents, as is supported by the increased concentrations along the MR transect further offshore and decreased concentrations along shelf, the primary discharge direction of the MR (Fig. A2).
Inputs from typical wetland taxa, thereby likely representing local southern Louisiana vegetation, are also recognized in the northern GoM. Typical wetland taxa found in pollen studies from Louisiana, although not necessarily restricted to wetlands, include Taxodium, Salix, Amaranthaceae, Typha, Asteraceae, Poaceae, and Cyperaceae (Kiage, 2020; Yao et al., 2020; Ryu et al., 2021). Pollen from most of these taxa is present in the northern GoM sediments, often close to the coast (Fig. 4), except for the common mangrove taxa Rhizophora and Avicennia, which we did not find in the sediments studied here. Although mangrove vegetation is abundant on the Louisiana coast (Day et al., 2020), pollen from these mangrove taxa is not dominant in pollen studies from Louisiana, especially in the last 100 years (Kiage, 2020; Yao et al., 2020). Moreover, some of these taxa (Amaranthaceae, Typha, and Asteraceae) are relatively more abundant just west of the MR and close to the AR, suggesting that they are sourced from local inputs rather than discharged by the river. Nevertheless, these local pollen inputs seem to remain close to the coast and are not transported far into the open ocean (Brush and DeFries, 1981).
4.2 Palynology-based environmental interpretations and indices
4.2.1 Palynology as an indicator for marine productivity
In the northern GoM, heterotrophic dinocysts dominate the assemblages on the shelf and along the coast, decreasing further into the open ocean. Because heterotrophic dinocysts feed on organic matter, their relative abundances provide a good indication of marine production and have been often used to study past nutrient availability and eutrophication in coastal oceans (Matsuoka, 1999; Sangiorgi and Donders, 2004; de Vernal and Marret, 2007; Dale, 2009). The high abundances of heterotrophic dinocysts on the shelf match with high concentrations of lipid biomarkers derived from primary producers such as diatoms, haptophyte algae, and dinoflagellates in the same sediments (Yedema et al., 2023), which are fueled by the input of nutrients from the MAR (Lohrenz et al., 1990, 1999). The dominance of heterotrophic dinocysts in areas where primary production is high is reflected in the ratio, which is high along the shelf and decreases towards the open ocean (Fig. 5a).
Previous studies from the GoM also indicate that coastal assemblages were dominated by heterotrophic dinocysts (Price et al., 2018), while autotrophic dinocysts were more dominant in assemblages both offshore and east of the MR (Limoges et al., 2013). One exception in their study is a site close to the Pigmy basin (Fig. 1), where high abundances of Brigantedinium spp. were reported. As mentioned before, this basin serves as an accumulation site for the organic matter from the Mississippi river, and it is therefore likely that this basin provides food resources for heterotrophic taxa. Overall, all studies agree that heterotrophically dominated assemblages, and hence high values, are found in the high-nutrient environments close to shore. Since the ratio also correlates well with the NPP in the GoM (r=0.67, p<0.005), it is, therefore, a reliable proxy for marine primary productivity.
Nevertheless, the use of the ratio as a (paleo)productivity indicator does warrant some caution. Besides a high ratio on the nutrient-rich Louisiana shelf, the ratio is also high on the western shelf, where net primary productivity is lower. Similarly high ratios have also been found in different dinocyst assemblages from areas with varying nutrient levels, indicating that using the ratio as a sole proxy can be misleading (Ramírez-Valencia et al., 2021). Also, in regions with varying oxygen levels, the use of relative abundances of heterotrophs may be influenced by the differential preservation potential for autotrophic and heterotrophic dinocysts (Zonneveld et al., 2001, 2008, 2010; Gray et al., 2017). Heterotrophic taxa such as Brigantedinium spp. and Echinidinium spp. are particularly sensitive to oxygen availability, while most autotrophic taxa including Impagidinium spp., cysts of P. dalei, and P. zoharyi are more resistant (Zonneveld et al., 2001). This difference likely derives from chemical variations in their cyst structures as a result of their nutrition strategies (Bogus et al., 2014). In the GoM, this differentiation in preservation potential is especially complex, as much of the shelf is subjected to hypoxic conditions during summers, while bottom waters are generally well oxygenated during winter months. Heterotrophic dinocyst concentrations seem mostly controlled by bottom water oxygen levels in the seasonally hypoxic Quiberon Bay (Lambert et al., 2022). A similar control in the GoM could create a preservation bias in the dinocyst assemblage and result in higher relative abundances of heterotrophic dinocysts within the hypoxic zone, rendering the ratio less reliable. Other studies have linked low abundances of heterotrophic dinocysts to coarse-grained sediments, as these porous sediments generally contain higher oxygen levels (Lambert et al., 2022). Although the coarse-grained sediments that are present at the westernmost shelf locations in the GoM had low dinocyst concentrations (∼ 230 cysts g−1), the heterotrophic dinocysts remained dominant, suggesting that the ratio was not affected by preferential degradation, despite being located outside the seasonally hypoxic zone (Figs. 5a, 6). Hence, the distribution of heterotrophic taxa in the northern GoM seems to be mainly controlled by the net primary productivity and the availability of energy resources. Absolute dinocyst concentrations and fluxes are less affected by oxygen levels and potential preservation biases between autotrophic and heterotrophic dinocysts and could therefore provide a clearer link with primary production (Zonneveld et al., 2009). Indeed, dinocyst concentrations have been linked to high nutrient levels and eutrophication in estuaries and coastal waters (Radi et al., 2007; Dale, 2009; Zonneveld et al., 2009), although this correlation is not always found (de Vernal et al., 2020). In the GoM, the highest dinocyst concentrations occur on the shelf between the MR and AR mouths, concurrent with the highest ratio (Fig. 5a). However, the total dinocyst concentrations decrease on the western shelf and along the MR transect, while the ratio remains high in these regions (68 %–80 % and 63 %–77 %, respectively). This indicates a discrepancy between total dinocyst concentrations and the ratio (r=0.13, p=>0.05), although the highest dinocyst concentrations and ratio values both occur close to the MAR, where primary production is most pronounced (Lohrenz et al., 1990; Yedema et al., 2023). We, therefore, propose that a combination of the ratio and total dinocyst concentration is the best way to explore marine productivity in modern and past oceans. In addition, individual dinocyst taxa can be used as an indicator for nutrient availability. For example, L. machaerophorum and Polykrikos are traditionally linked to eutrophic conditions (Wall and Dale, 1969; Matsuoka et al., 2003; Sangiorgi and Donders, 2004; Dale, 2009), although some studies oppose these views (Godhe and McQuoid, 2003). In the GoM, the predominant presence of L. machaerophorum around the shelf break and its underrepresentation on the shelf, where most primary production takes place (Limoges et al., 2013; this study), suggest that L. machaerophorum is not linked to high marine productivity or nutrient availability here. This is likely caused by the turbid outflow of the MAR, which creates unfavorable conditions for this species. On the other hand, cysts of Polykrikos were very abundant on the shelf between the MR and AR rivers, where also the highest ratio and total dinocyst concentrations were found. On the other hand, the RDA results show that Polykrikos plots in the same direction as the ratio and NPP, opposite to L. machaerophorum (Fig. 6). Therefore, we suggest that cysts of Polykrikos are better indicators for marine productivity in the GoM compared to L. machaerophorum.
4.2.2 Palynology as a proxy for coastal proximity
The ratio between pollen (excluding bisaccates) and dinocysts, quantified in the ratio, decreases both along the shelf and further offshore in the northern GoM, although the ratio values remain relatively high offshore from the MR transect (Fig. 5b). This trend fits with the general interpretation of the ratio that high values indicate high contributions of fluvially discharged pollen and thus proximity to land (De Vernal et al., 1991; McCarthy and Mudie, 1998; van Soelen et al., 2010; Donders et al., 2018). Accordingly, the ratio plots within the river mouth quadrant in the RDA plot, in the opposite direction to both the Mississippi and Atchafalaya river mouths (Fig. 6).
The ratio between pollen and bisaccates ( ratio) shows a similar trend to the ratio (Fig. 5b, c), implying that both proxies could indicate the distance to the coast and/or river. As Pinus pollen dominates the bisaccate assemblages in the northern GoM, and contributions of other bisaccates (i.e., Abies and Picea) are minor, the concentration of Pinus and total bisaccates can be used interchangeably here. The interpretation of the ratio as an indicator for distance to the coast is based on the assumption that Pinus pollen is more susceptible to wind transport than non-bisaccate pollen and is therefore suspected to be transported further offshore (Mudie and McCarthy, 2006, and references therein). The ratio has been applied as such in Cenozoic and Mesozoic paleoreconstructions (e.g., Tribovillard and Gorin, 1991; Pieñkowski and Waksmundzka, 2009), and more recently, this ratio has also been proposed to indicate relative sea level rise during glacial–interglacial cycles in the East China Sea (Zheng et al., 2011). Here, we show that this ratio can indeed be an independent indicator for distance to the coast. The ratio decreases from 81 %–63 % on the shelf, 70 %–39 % on the AR transect, and 81 %–45 % on the MR transect. This shows that bisaccates become more dominant further from the river mouth, regardless of total pollen or bisaccate concentrations, although the largest decrease in ratio values occurs along the land–sea transects with increasing distance from the coast, while along the shelf transect, which follows the direction of the river plume, the decrease in ratio values is less pronounced. A study in the South China Sea suggested that pollen assemblages at < 200 m water depth were dominated by river-transported pollen, while deeper waters (> 200 m) were characterized by wind-transported pollen (Luo et al., 2014). However, the same trend was seen in coastal areas without significant river input (Mudie and McCarthy, 1994), suggesting that the ratio, in comparison to the ratio, primarily reflects distance to the coast rather than river input. The difference between the two ratios is most clear along the shelf transect, where the ratio shows a more prominent decrease than the ratio. Further offshore, changes in the ratio are more pronounced, which is also evident from the RDA results, where the ratio plots further in the opposite direction to the water depth and distance to the coast and MAR parameters than the ratio (Fig. 6). As a result, the ratio is a more robust indication of distance to shore and can, therefore, be used in sea level and paleoenvironmental reconstructions. The ratio on the other hand seems better suited as a proxy for river input.
It should be noted that these proxies might be less reliable in areas with frequent hurricane passages such as the GoM (Conner et al., 1989). Hurricanes and tropical storms can disrupt coastal vegetation and affect sediment distributions in the GoM, with increasing transport of finer material further offshore and towards the Mississippi Canyon (Goñi et al., 2006; Sampere et al., 2008). In downcore pollen studies from southern Louisiana, hurricane events are related to coarse sediment layers and higher abundances of disturbance (i.e., Amaranthaceae) pollen taxa (e.g., Kiage, 2020). Since this study only analyzed surface sediments and has no downcore record, we cannot directly verify the effect of hurricanes on our sediment distributions. However, pollen from the indicated disturbance taxa is not dominant in the surface sediments but remains most abundant close to the coast (Fig. 4a). Furthermore, pollen derived from the Mississippi river is most abundant close to the river mouth, with little evidence of enhanced offshore transport. This is also supported by the distributions of terrestrial biomarkers in the northern GoM, which are also mostly found close to shore (Yedema et al., 2023). Overall, there is no clear indication of sediment and/or vegetation disruption by hurricanes in the studied surface sediments.
Palynological analysis of surface sediments along three transects in the northern Gulf of Mexico indicates that pollen and dinocyst concentrations vary spatially. Total dinocyst concentrations were highest on the Louisiana shelf and are dominated by heterotrophic taxa close to the coast and by autotrophic taxa further offshore. Redundancy analysis revealed specific niches for individual dinocyst taxa (i.e., coastal, shelf break, and open ocean) which are primarily influenced by high marine productivity, linked to high nutrient levels. The spatial changes in dinocyst assemblages support the use of the ratio to trace marine productivity. However, especially in areas where seasonal hypoxia occurs, such as the northern GoM, the ratio can be best used in combination with total dinocyst concentrations to avoid bias due to dinocyst differential preservation.
Pollen concentrations peaked close to the Mississippi river mouth and decreased rapidly further offshore. Bisaccate (Pinus) concentrations were highest offshore from the MR and became more dominant compared to total pollen concentrations further offshore. The ratio decreases mostly along the land–sea transects and less along the shelf, indicating that it best reflects the distance to the coast. The ratio decreases more towards the shelf in the direction of the river plume and, therefore, better represents river input.
Microscope slides from the surface sediments from R/V Pelagia cruise 64PE467 are stored at Utrecht University. Datasets for this article can be found at https://doi.org/10.5281/zenodo.8164437 (Yedema et al., 2023) and at https://doi.org/10.1594/PANGAEA.944838 (Yedema et al., 2022).
The research was designed by FS and FP. FS and FP led the research expedition that collected the sediments. Slides were prepared and counted by YWY, while FS and TD checked the counts. YWY wrote the article, while all authors helped with the data interpretation and provided feedback on the manuscript.
At least one of the (co-)authors is a member of the editorial board of Journal of Micropalaeontology. The peer-review process was guided by an independent editor, and the authors also have no other competing interests to declare.
Publisher’s note: Copernicus Publications remains neutral with regard to jurisdictional claims made in the text, published maps, institutional affiliations, or any other geographical representation in this paper. While Copernicus Publications makes every effort to include appropriate place names, the final responsibility lies with the authors.
We thank the editor, Debra Willard, and one anonymous reviewer for their comments and feedback, which improved the manuscript. This work was carried out under the program of the Netherlands Earth System Science Centre (NESSC). We thank the scientists and crew aboard the R/V Pelagia cruise 64PE467. Lab technicians at Utrecht University (Natasja Welters, Giovanni Dammers, and Helen de Waard) are thanked for their support with preparing the microscope slides.
This research has been supported by the Netherlands Earth System Science Centre (grant no. 024.002.001).
This paper was edited by Luke Mander and reviewed by Debra A. Willard and one anonymous referee.
Barbour, M. G. and Billings, W. D.: North American terrestrial vegetation, Cambridge University Press, ISBN: 0521559863, 9780521559867, 2000.
Beaudouin, C., Suc, J.-P., Escarguel, G., Arnaud, M., and Charmasson, S.: The significance of pollen signal in present-day marine terrigenous sediments: the example of the Gulf of Lions (western Mediterranean Sea), Geobios, 40, 159–172, https://doi.org/10.1016/j.geobios.2006.04.003, 2007.
Behrenfeld, M. J. and Falkowski, P. G.: Photosynthetic rates derived from satellite-based chlorophyll concentration, Limnol. Oceanogr., 42, 1–20, https://doi.org/10.4319/lo.1997.42.1.0001, 1997.
Benninghoff, W. S.: Calculation of pollen and spore density in sediments by addition of exotic pollen in known quantities, Science, 106, 325–326, 1962.
Birks, H. J. B.: Contributions of Quaternary botany to modern ecology and biogeography, Plant Ecol. Div., 12, 189–385, https://doi.org/10.1080/17550874.2019.1646831, 2019.
Bogus, K., Mertens, K. N., Lauwaert, J., Harding, I. C., Vrielinck, H., Zonneveld, K. A., and Versteegh, G. J.: Differences in the chemical composition of organic-walled dinoflagellate resting cysts from phototrophic and heterotrophic dinoflagellates, J. Phycol., 50, 254–266, https://doi.org/10.1111/jpy.12170, 2014.
Brown, A., Carpenter, R., and Walling, D.: Monitoring fluvial pollen transport, its relationship to catchment vegetation and implications for palaeoenvironmental studies, Rev. Palaeobot. Palyno., 147, 60–76, https://doi.org/10.1016/j.revpalbo.2007.06.005, 2007.
Brush, G. S. and Brush, L. M.: Transport of pollen in a sediment-laden channel; a laboratory study, Am. J. Sci., 272, 359–381, https://doi.org/10.2475/ajs.272.4.359, 1972.
Brush, G. S. and DeFries, R. S.: Spatial distributions of pollen in surface sediments of the Potomac estuary 1, Limnol. Oceanogr., 26, 295–309, https://doi.org/10.4319/lo.1981.26.2.0295, 1981.
Bujak, J. P.: Cenozoic dinoflagellate cysts and acritarchs from the Bering Sea and northern North Pacific, DSDP Leg 19, Micropaleontology, 30, 180–212, https://doi.org/10.2307/1485717, 1984.
Chaloner, W. and Muir, M.: Spores and floras, in: Coal and Coal bearing strata, edited by: Westoll, T. S. and Murchison, D. G., Oliver and Boyd Ltd., Edinburgh, Vol. 14, 127–146, 1968.
Chmura, G. L. and Liu, K.-B.: Pollen in the lower Mississippi River, Rev. Palaeobot. Palyno., 64, 253–261, https://doi.org/10.1016/0034-6667(90)90140-E, 1990.
Chmura, G. L., Smirnov, A., and Campbell, I. D.: Pollen transport through distributaries and depositional patterns in coastal waters, Palaeogeogr. Palaeocl., 149, 257–270, https://doi.org/10.1016/S0031-0182(98)00205-3, 1999.
Cochrane, J. D. and Kelly, F. J.: Low-frequency circulation on the Texas-Louisiana continental shelf, J. Geophys. Res., 91, 10645, https://doi.org/10.1029/JC091iC09p10645, 1986.
Cohen, M. C. L., Rossetti, D. de F., Pessenda, L. C. R., Friaes, Y. S., and de Oliveira, P. E.: Late Pleistocene glacial forest of Humaitá – Western Amazonia, Palaeogeogr. Palaeocl., 415, 37–47, https://doi.org/10.1016/j.palaeo.2013.12.025, 2014.
Coleman, J. M., Roberts, H. H., and Stone, G. W.: Mississippi River delta: an overview, J. Coast. Res., 14, 699–716, 1998.
Conner, W. H., Day, J. W., Baumann, R. H., and Randall, J. M.: Influence of hurricanes on coastal ecosystems along the northern Gulf of Mexico, Wetl. Ecol. Manag., 1, 45–56, https://doi.org/10.1007/BF00177889, 1989.
Coussin, V., Penaud, A., Combourieu-Nebout, N., Peyron, O., Schmidt, S., Zaragosi, S., de Vernal, A., and Babonneau, N.: Distribution of modern dinocysts and pollen in the western Mediterranean Sea (Algerian margin and Gulf of Lion), Mar. Micropaleontol., 175, 102157, https://doi.org/10.1016/j.marmicro.2022.102157, 2022.
Couvillion, B. R., Barras, J. A., Steyer, G. D., Sleavin, W., Fischer, M., Beck, H., Trahan, N., Griffin, B., and Heckman, D.: Land area change in coastal Louisiana from 1932 to 2010: U.S. Geological Survey Scientific Investigations Map 3164, scale 1:265 000, 12 pp., 2011.
Dai, L., Weng, C., Lu, J., and Mao, L.: Pollen quantitative distribution in marine and fluvial surface sediments from the northern South China Sea: new insights into pollen transportation and deposition mechanisms, Quaternary Int., 325, 136–149, https://doi.org/10.1016/j.quaint.2013.09.031, 2014.
Dale, B.: Dinoflagellate cyst ecology: modeling and geological applications, Palynology: Principles and Applications, American Association of Stratigraphic Palynologists Foundation, Dallas, edited by: Jansonius, J. and McGregor, DC, 1249–1275, ISBN 0-931871-03-4, 1996.
Dale, B.: Eutrophication signals in the sedimentary record of dinoflagellate cysts in coastal waters, J. Sea Res., 61, 103–113, https://doi.org/10.1016/j.seares.2008.06.007, 2009.
Dale, B., Dale, A. L., and Jansen, J. F.: Dinoflagellate cysts as environmental indicators in surface sediments from the Congo deep-sea fan and adjacent regions, Palaeogeogr. Palaeocl., 185, 309–338, https://doi.org/10.1016/S0031-0182(02)00380-2, 2002.
Day, R., Michot, T., Twilley, R., and From, A.: Geographic distribution of black mangrove (Avicennia germinans) in coastal Louisiana in 2009, US Geoglogical Survey data release, https://doi.org/10.5066/P9RC8EIE, 2020.
Delcourt, P. A. and Delcourt, H. R.: Late Quaternary paleoclimates and biotic responses in eastern North America and the western North Atlantic Ocean, Palaeogeogr. Palaeocl., 48, 263–284, https://doi.org/10.1016/0031-0182(84)90048-8, 1984.
Delcourt, P. A. and Delcourt, H. R.: Quaternary paleoecology of the Lower Mississippi Valley, Eng. Geol., 45, 219–242, https://doi.org/10.1016/S0013-7952(96)00015-4, 1996.
De Vernal, A., Giroux, L., and Hillaire-Marcel, C.: Distribution des palynomorphes et flux de matière organique dans un milieu de transition, Exemple de L'estuaire et du golfe du Saint-Laurent, Palynosciences, 1, 145–157, 1991.
de Vernal, A.: Marine palynology and its use for studying nearshore environments, IOP Conference Series: Earth and Environmental Science, Vol. 5, From Deep-Sea To Coastal Zones: Methods And Techniques For Studying Paleoenvironments, 25–29 February 2008, Faro, Portugal, 012002, https://doi.org/10.1088/1755-1307/5/1/012002, 2009.
de Vernal, A. and Marret, F.: Chapter nine organic-walled dinoflagellate cysts: tracers of sea-surface conditions, Dev. Mar. Geol., 1, 371–408, https://doi.org/10.1016/S1572-5480(07)01014-7, 2007.
de Vernal, A., Radi, T., Zaragosi, S., Van Nieuwenhove, N., Rochon, A., Allan, E., De Schepper, S., Eynaud, F., Head, M. J., Limoges, A., Londeix, L., Marret, F., Matthiessen, J., Penaud, A., Pospelova, V., Price, A., and Richerol, T.: Distribution of common modern dinoflagellate cyst taxa in surface sediments of the Northern Hemisphere in relation to environmental parameters: The new n=1968 database, Mar. Micropaleontol., 159, 101796, https://doi.org/10.1016/j.marmicro.2019.101796, 2020.
Donders, T. H., Van Helmond, N. A., Verreussel, R., Munsterman, D., ten Veen, J., Speijer, R. P., Weijers, J. W., Sangiorgi, F., Peterse, F., and Reichart, G.-J.: Land–sea coupling of early Pleistocene glacial cycles in the southern North Sea exhibit dominant Northern Hemisphere forcing, Clim. Past, 14, 397–411, https://doi.org/10.5194/cp-14-397-2018, 2018.
Edwards, L. and Willard, D.: Dinoflagellate cysts and pollen from sediment samples, Mississippi Sound and Gulf of Mexico, Stratigraphic Framework of the Neogene and Quaternary Sediments of the Mississippi Coastal Zone, Jackson County, Mississippi, US Geological Survey Open-file Report, 1–415, 2001.
Florin, R.: The distribution of conifer and taxad genera in time and space, Acta Horti Bergiani, 20, 121–324, 1963.
Garcia, H., Weathers, K., Paver, C., Smolyar, I., Boyer, T., Locarnini, M., Zweng, M., Mishonov, A., Baranova, O., and Seidov, D.: World ocean atlas 2018, Vol. 4, Dissolved inorganic nutrients (phosphate, nitrate and nitrate + nitrite, silicate), NOAA Atlas NESDIS, 84, 35 pp., https://archimer.ifremer.fr/doc/00651/76336/ (last access: 24 November 2023), 2019a.
Garcia, H., Weathers, K., Paver, C., Smolyar, I., Boyer, T., Locarnini, M., Zweng, M., Mishonov, A., Baranova, O., and Seidov, D.: World Ocean Atlas 2018, Vol. 3, Dissolved Oxygen, Apparent Oxygen Utilization, and Dissolved Oxygen Saturation, NOAA Atlas NESDIS, 83, 38 pp., https://archimer.ifremer.fr/doc/00651/76337/ (last access: 24 November 2023), 2019b.
Godhe, A. and McQuoid, M. R.: Influence of benthic and pelagic environmental factors on the distribution of dinoflagellate cysts in surface sediments along the Swedish west coast, Aquat. Microb. Ecol., 32, 185–201, https://doi.org/10.3354/ame032185, 2003.
Goñi, M. A., Gordon, E. S., Monacci, N. M., Clinton, R., Gisewhite, R., Allison, M. A., and Kineke, G.: The effect of Hurricane Lili on the distribution of organic matter along the inner Louisiana shelf (Gulf of Mexico, USA), Cont. Shelf Res., 26, 2260–2280, https://doi.org/10.1016/j.csr.2006.07.017, 2006.
Gray, D. D., Zonneveld, K. A., and Versteegh, G. J.: Species-specific sensitivity of dinoflagellate cysts to aerobic degradation: A five-year natural exposure experiment, Rev. Palaeobot. Palyno., 247, 175–187, https://doi.org/10.1016/j.revpalbo.2017.09.002, 2017.
Groot, J. J. and Groot, C. R.: Marine palynology: possibilities, limitations, problems, Mar. Geol., 4, 387–395, https://doi.org/10.1016/0025-3227(66)90007-7, 1966.
Head, M.: Modern dinoflagellate cysts and their biological affinities, Palynology, 3, 1197–1248, https://doi.org/10.2307/1485834, 1996.
Heusser, L.: Pollen in Santa Barbara Basin, California: a 12,000-yr record, Geol. Soc. Am. Bull., 89, 673–678, https://doi.org/10.1130/0016-7606(1978)89<673:PISBBC>2.0.CO;2, 1978.
Heusser, L. E.: Pollen distribution in the bottom sediments of the western North Atlantic Ocean, Mar. Micropaleontol., 8, 77–88, https://doi.org/10.1016/0377-8398(83)90006-3, 1983.
Heusser, L. E.: Pollen distribution in marine sediments on the continental margin off northern California, Mar. Geol., 80, 131–147, https://doi.org/10.1016/0025-3227(88)90076-X, 1988.
Hill, M. O. and Gauch, H. G.: Detrended correspondence analysis: an improved ordination technique, Classification and ordination: Symposium on advances in vegetation science, Nijmegen, the Netherlands, May 1979, 47–58, https://doi.org/10.1007/978-94-009-9197-2_7, 1980.
Hooghiemstra, H.: Palynological records from northwest African marine sediments: a general outline of the interpretation of the pollen signal, Philos. T. R. Soc. Lond. B, 318, 431–449, https://doi.org/10.1098/rstb.1988.0018, 1988.
Hooghiemstra, H., Lézine, A.-M., Leroy, S. A., Dupont, L., and Marret, F.: Late Quaternary palynology in marine sediments: a synthesis of the understanding of pollen distribution patterns in the NW African setting, Quaternary Int., 148, 29–44, https://doi.org/10.1016/j.quaint.2005.11.005, 2006.
Hopkins, J. S.: Differential flotation and deposition of coniferous and deciduous tree pollen, Ecology, 31, 633–641, 1950.
Hoppenrath, M., Elbrächter, M., and Drebes, G.: Marine phytoplankton, ISBN 978-3-510-61392-2, 2009.
Iria, G.-M., Costas, S. V., García-Gil, S., and Sobrino, C. M.: Organic-walled dinoflagellate cyst assemblages in surface sediments of the Ría de Vigo (Atlantic margin of NW Iberia) in relation to environmental gradients, Mar. Micropaleontol., 18, 102217, https://doi.org/10.1016/j.marmicro.2023.102217, 2023.
Jacobson, D. M. and Anderson, D. M.: Thecate heterophic dinoflagellates: feeding behavior and mechanisms 1, J. Phycol., 22, 249–258, https://doi.org/10.1111/j.1529-8817.1986.tb00021.x, 1986.
Jasper, J. P. and Gagosian, R. B.: The sources and deposition of organic matter in the Late Quaternary Pigmy Basin, Gulf of Mexico, Geochim. Cosmochim. Ac., 54, 1117–1132, https://doi.org/10.1016/0016-7037(90)90443-O, 1990.
Jeong, H. J., Yoo, Y. D., Kim, J. S., Seong, K. A., Kang, N. S., and Kim, T. H.: Growth, feeding and ecological roles of the mixotrophic and heterotrophic dinoflagellates in marine planktonic food webs, Ocean Sci. J., 45, 65–91, https://doi.org/10.1007/s12601-010-0007-2, 2010.
Kiage, L. M.: A 1200-year history of environmental changes in Bay Jimmy area, coastal Louisiana, USA, Holocene, 30, 201–209, https://doi.org/10.1177/0959683619875801, 2020.
Lambert, C., Penaud, A., Poirier, C., and Goubert, E.: Distribution of modern dinocysts in surface sediments of southern Brittany (NW France) in relation to environmental parameters: Implications for paleoreconstructions, Rev. Palaeobot. Palyno., 297, 104578, https://doi.org/10.1016/j.revpalbo.2021.104578, 2022.
Legendre, P. and Birks, H. J. B.: From classical to canonical ordination, Tracking environmental change using lake sediments: Data handling and numerical techniques, 201–248, https://doi.org/10.1007/978-94-007-2745-8_8, 2012.
Leroy, S. A., Marret, F., Gibert, E., Chalié, F., Reyss, J.-L., and Arpe, K.: River inflow and salinity changes in the Caspian Sea during the last 5500 years, Quaternary Sci. Rev., 26, 3359–3383, https://doi.org/10.1016/j.quascirev.2007.09.012, 2007.
Li, Z., Pospelova, V., Liu, L., Zhou, R., and Song, B.: High-resolution palynological record of Holocene climatic and oceanographic changes in the northern South China Sea, Palaeogeogr. Palaeoclimatol., 483, 94–124, https://doi.org/10.1016/j.palaeo.2017.03.009, 2017.
Lieux, M.: An atlas of pollen of trees, shrubs, and woody vines of Louisiana and other southeastern States, Part 1, Ginkgoaceae to Lauraceae, Pollen Spores, 22, 17–57, 1980.
Limoges, A., Londeix, L., and de Vernal, A.: Organic-walled dinoflagellate cyst distribution in the Gulf of Mexico, Mar. Micropaleontol., 102, 51–68, https://doi.org/10.1016/j.marmicro.2013.06.002, 2013.
Limoges, A., de Vernal, A., and Van Nieuwenhove, N.: Long-term hydrological changes in the northeastern Gulf of Mexico (ODP-625B) during the Holocene and late Pleistocene inferred from organic-walled dinoflagellate cysts, Palaeogeogr. Palaeocl., 414, 178–191, https://doi.org/10.1016/j.palaeo.2014.08.019, 2014.
Locarnini, R. A., Mishonov, A. V., Antonov, J. I., Boyer, T. P., Garcia, H. E., Baranova, O. K., Zweng, M. M., Paver, C. R., Reagan, J. R., Johnson, D. R., Hamilton, M., and Seidov, D.: World Ocean Atlas 2013, Vol. 1, Temperature, edited by: Levitus, S., Technical edited by: A. Mishonov, NOAA Atlas NESDIS 73, 40 pp., 2013.
Lohrenz, S. E., Dagg, M. J., and Whitledge, T. E.: Enhanced primary production at the plume/oceanic interface of the Mississippi River, Cont. Shelf Res., 10, 639–664, https://doi.org/10.1016/0278-4343(90)90043-L, 1990.
Lohrenz, S. E., Fahnenstiel, G. L., Redalje, D. G., Lang, G. A., Dagg, M. J., Whitledge, T. E., and Dortch, Q.: Nutrients, irradiance, and mixing as factors regulating primary production in coastal waters impacted by the Mississippi River plume, Cont. Shelf Res., 19, 1113–1141, https://doi.org/10.1016/S0278-4343(99)00012-6, 1999.
Luo, C., Chen, M., Xiang, R., Liu, J., Zhang, L., Lu, J., and Yang, M.: Modern pollen distribution in marine sediments from the northern part of the South China Sea, Mar. Micropaleontol., 108, 41–56, https://doi.org/10.1016/j.marmicro.2014.03.001, 2014.
Marret, F., Bradley, L. R., Tarasov, P. E., Ivanova, E. V., Zenina, M. A., and Murdmaa, I. O.: The Holocene history of the NE Black Sea and surrounding areas: An integrated record of marine and terrestrial palaeoenvironmental change, Holocene, 29, 648–661, https://doi.org/10.1177/0959683618824769, 2019.
Matsuoka, K.: Eutrophication process recorded in dinoflagellate cyst assemblages – a case of Yokohama Port, Tokyo Bay, Japan, Sci. Total Environ., 231, 17–35, https://doi.org/10.1016/S0048-9697(99)00087-X, 1999.
Matsuoka, K., Joyce, L. B., Kotani, Y., and Matsuyama, Y.: Modern dinoflagellate cysts in hypertrophic coastal waters of Tokyo Bay, Japan, J. Plank. Res., 25, 1461–1470, 2003.
McCarthy, F. M. and Mudie, P. J.: Oceanic pollen transport and pollen: dinocyst ratios as markers of late Cenozoic sea level change and sediment transport, Palaeogeogr. Palaeocl., 138, 187–206, 1998.
Mertens, K. N., Gu, H., Takano, Y., Price, A. M., Pospelova, V., Bogus, K., Versteegh, G. J. M., Marret, F., Turner, R. E., Rabalais, N. N., and Matsuoka, K.: The cyst-theca relationship of the dinoflagellate cyst Trinovantedinium pallidifulvum, with erection of Protoperidinium lousianensis sp. nov. and their phylogenetic position within the Conica group, Palynology, 41, 183–202, https://doi.org/10.1080/01916122.2016.1147219, 2017.
Milliman, J. D. and Syvitski, J. P. M.: Geomorphic/Tectonic Control of Sediment Discharge to the Ocean: The Importance of Small Mountainous Rivers, J. Geol., 100, 525–544, https://doi.org/10.1086/629606, 1992.
Montero-Serrano, J. C., Bout-Roumazeilles, V., Tribovillard, N., Sionneau, T., Riboulleau, A., Bory, A., and Flower, B.: Sedimentary evidence of deglacial megafloods in the northern Gulf of Mexico (Pigmy Basin), Quaternary Sci. Rev., 28, 3333–3347, https://doi.org/10.1016/j.quascirev.2009.09.011, 2009.
Mudie, P.: Pollen distribution in recent marine sediments, eastern Canada, Can. J. Earth Sci., 19, 729–747, https://doi.org/10.1139/e82-062, 1982.
Mudie, P. J. and McCarthy, F. M.: Marine palynology: potentials for onshore – offshore correlation of Pleistocene – Holocene records, T. Roy. Soc. S. Afr., 61, 139–157, https://doi.org/10.1080/00359190609519964, 2006.
Mudie, P. J. and McCarthy, F. M. G.: Late quaternary pollen transport processes, western North Atlantic: Data from box models, cross-margin and N-S transects, Mar. Geol., 118, 79–105, https://doi.org/10.1016/0025-3227(94)90114-7, 1994.
Mudie, P. J., Yanko-Hombach, V. V., and Mudryk, I.: Palynomorphs in surface sediments of the North-Western Black Sea as indicators of environmental conditions, Quaternary Int., 590, 122–145, https://doi.org/10.1016/j.quaint.2020.05.014, 2021.
Muller, J.: Palynology of Recent Orinoco delta and shelf sediments; reports of the Orinoco shelf expedition, Micropaleontology, 5, 1–32, https://doi.org/10.2307/1484153, 1959.
Munoz Sobrino, C., García-Gil, S., Iglesias, J., Martinez Carreno, N., Ferreiro Da Costa, J., Diaz Varela, R. A., and Judd, A.: Environmental change in the R ía de V igo, NW I beria, since the mid-H olocene: new palaeoecological and seismic evidence, Boreas, 41, 578–601, https://doi.org/10.1111/j.1502-3885.2012.00255.x, 2012.
Nyman, J. A., Reid, C. S., Sasser, C. E., Linscombe, J., Hartley, S. B., Couvillion, B. R., and Villani, R. K.: Vegetation Types in Coastal Louisiana in 2021 (ver. 2.0, April 2023): US Geological Survey data release, https://doi.org/10.5066/P9URYLMS, 2021.
Oksanen, J., Blanchet, F. G., Kindt, R., Legendre, P., Minchin, P. R., O'hara, R., Simpson, G. L., Solymos, P., Stevens, M. H. H., and Wagner, H.: Package “vegan”, Community ecology package, version, 2, 1–295, 2013.
Omernik, J. M. and Griffith, G. E.: Ecoregions of the conterminous United States: evolution of a hierarchical spatial framework, Environ. Manag., 54, 1249–1266, https://doi.org/10.1007/s00267-014-0364-1, 2014.
Pieńkowski, A. J., Mudie, P. J., England, J. H., Smith, J. N., and Furze, M. F.: Late Holocene environmental conditions in Coronation Gulf, southwestern Canadian Arctic Archipelago: evidence from dinoflagellate cysts, other non-pollen palynomorphs, and pollen, J. Quaternary Sci., 26, 839–853, https://doi.org/10.1002/jqs.1503, 2011.
Pieñkowski, G. and Waksmundzka, M.: Palynofacies in Lower Jurassic epicontinental deposits of Poland: tool to interpret sedimentary environments, Episod. J. Int. Geosci., 32, 21–32, https://doi.org/10.18814/epiiugs/2009/v32i1/004, 2009.
Price, A. M., Baustian, M. M., Turner, R. E., Rabalais, N. N., and Chmura, G. L.: Melitasphaeridium choanophorum – a living fossil dinoflagellate cyst in the Gulf of Mexico, Palynology, 41, 351–358, https://doi.org/10.1080/01916122.2016.1205676, 2017.
Price, A. M., Baustian, M. M., Turner, R. E., Rabalais, N. N., and Chmura, G. L.: Dinoflagellate Cysts Track Eutrophication in the Northern Gulf of Mexico, Estuar. Coast., 41, 1322–1336, https://doi.org/10.1007/s12237-017-0351-x, 2018.
Pross, J. and Brinkhuis, H.: Organic-walled dinoflagellate cysts as paleoenvironmental indicators in the Paleogene; a synopsis of concepts, Palaontol. Z., 79, 53–59, https://doi.org/10.1007/BF03021753, 2005.
Rabalais, N. N. and Turner, R. E.: Gulf of Mexico hypoxia: Past, present, and future, Limnol. Oceanogr. Bull., 28, 117–124, https://doi.org/10.1002/lob.10351, 2019.
Rabalais, N. N., Turner, R. E., and Scavia, D.: Beyond Science into Policy: Gulf of Mexico Hypoxia and the Mississippi River, BioScience, 52, 129–142, https://doi.org/10.1641/0006-3568(2002)052[0129:BSIPGO]2.0.CO;2, 2002.
Rabalais, N. N., Turner, R. E., Sen Gupta, B. K., Boesch, D. F., Chapman, P., and Murrell, M. C.: Hypoxia in the northern Gulf of Mexico: Does the science support the Plan to Reduce, Mitigate, and Control Hypoxia?, Estuar. Coast., 30, 753–772, https://doi.org/10.1007/BF02841332, 2007.
Radi, T. and de Vernal, A.: Dinocysts as proxy of primary productivity in mid–high latitudes of the Northern Hemisphere, Mar. Micropaleontol., 68, 84–114, https://doi.org/10.1016/j.marmicro.2008.01.012, 2008.
Radi, T., Pospelova, V., de Vernal, A., and Barrie, J. V.: Dinoflagellate cysts as indicators of water quality and productivity in British Columbia estuarine environments, Mar. Micropaleontol., 62, 269–297, https://doi.org/10.1016/j.marmicro.2006.09.002, 2007.
Ramírez-Valencia, V., Paez-Reyes, M., Salgado, J., Sangiorgi, F., Zúñiga-González, A. C., Amézquita, A., Ibarra-Ávila, H., and González-Arango, C.: Distribution of organic-walled dinoflagellate cysts in surface sediments of the southern Caribbean and the eastern tropical Pacific and its environmental implications, Mar. Micropaleontol., 167, 102000, https://doi.org/10.1016/j.marmicro.2021.102000, 2021.
Reuss, M.: Designing the bayous: The control of water in the Atchafalaya Basin, 1800–1995, Texas A&M University Press, https://doi.org/10.2307/27649250, 2004.
Rochon, A. and Vernal, A. de: Palynomorph distribution in recent sediments from the Labrador Sea, Can. J. Earth Sci., 31, 115–127, https://doi.org/10.1139/e94-010, 1994.
Rochon, A., Vernal, A. de, Turon, J.-L., Matthiessen, J., and Head, M. J.: Distribution of recent dinoflagellate cysts in surface sediments from the North Atlantic Ocean and adjacent seas in relation to sea-surface parameters, American Association of Stratigraphic Palynologists Contribution Series, 35, 1–146, 1999.
Ryu, J., Liu, K., Bianchette, T. A., and McCloskey, T.: Identifying forcing agents of environmental change and ecological response on the Mississippi River Delta, Southeastern Louisiana, Sci. Total Environ., 794, 148730, https://doi.org/10.1016/j.scitotenv.2021.148730, 2021.
Sampere, T. P., Bianchi, T. S., Wakeham, S. G., and Allison, M. A.: Sources of organic matter in surface sediments of the Louisiana Continental margin: effects of major depositional/transport pathways and Hurricane Ivan, Cont. Shelf Res., 28, 2472–2487, https://doi.org/10.1016/j.csr.2008.06.009, 2008.
Sangiorgi, F. and Donders, T. H.: Reconstructing 150 years of eutrophication in the north-western Adriatic Sea (Italy) using dinoflagellate cysts, pollen and spores, Estuar. Coast. Shelf Sci., 60, 69–79, https://doi.org/10.1016/j.ecss.2003.12.001, 2004.
Schiller, R., Kourafalou, V., Hogan, P., and Walker, N.: The dynamics of the Mississippi River plume: Impact of topography, wind and offshore forcing on the fate of plume waters, J. Geophys. Res.-Ocean., 116, C06029, https://doi.org/10.1029/2010JC006883, 2011.
Sturges, W. and Evans, J.: On the variability of the Loop Current in the Gulf of Mexico, J. Mar. Res., 41, 639–653, https://doi.org/10.1357/002224083788520487, 1983.
Sun, X. and Li, X.: Different dynamics and routes of modern pollen transport in the northern and southern parts of the South China Sea, Sci. China Ser. D, 41, 57–61, https://doi.org/10.1007/BF02932421, 1998.
Sun, X., Li, X., and Beug, H.-J.: Pollen distribution in hemipelagic surface sediments of the South China Sea and its relation to modern vegetation distribution, Mar. Geol., 156, 211–226, https://doi.org/10.1016/S0025-3227(98)00180-7, 1999.
Thöle, L. M., Nooteboom, P. D., Hou, S., Wang, R., Nie, S., Michel, E., Sauermilch, I., Marret, F., Sangiorgi, F., and Bijl, P. K.: An expanded database of Southern Hemisphere surface sediment dinoflagellate cyst assemblages and their oceanographic affinities, J. Micropalaeontol., 42, 35–56, https://doi.org/10.5194/jm-42-35-2023, 2023.
Tian, H., Xu, R., Pan, S., Yao, Y., Bian, Z., Cai, W., Hopkinson, C. S., Justic, D., Lohrenz, S., and Lu, C.: Long-term trajectory of nitrogen loading and delivery from Mississippi River Basin to the Gulf of Mexico, Global Biogeochem. Cy., 34, e2019GB006475, https://doi.org/10.1029/2019GB006475, 2020.
Tribovillard, N.-P. and Gorin, G. E.: Organic facies of the early Albian Niveau Paquier, a key black shales horizon of the Marnes Bleues formation in the Vocontian Trough (Subalpine Ranges, SE France), Palaeogeogr. Palaeocl., 85, 227–237, https://doi.org/10.1016/0031-0182(91)90162-K, 1991.
van Helmond, N. A., Hennekam, R., Donders, T. H., Bunnik, F. P., de Lange, G. J., Brinkhuis, H., and Sangiorgi, F.: Marine productivity leads organic matter preservation in sapropel S1: palynological evidence from a core east of the Nile River outflow, Quaternary Sci. Rev., 108, 130–138, https://doi.org/10.1016/j.quascirev.2014.11.014, 2015.
van Soelen, E. E., Lammertsma, E. I., Cremer, H., Donders, T. H., Sangiorgi, F., Brooks, G. R., Larson, R. A., Sinninghe Damsté, J. S., Wagner-Cremer, F., and Reichart, G. J.: Late Holocene sea-level rise in Tampa Bay: Integrated reconstruction using biomarkers, pollen, organic-walled dinoflagellate cysts, and diatoms, Estuar. Coast. Shelf Sci., 86, 216–224, https://doi.org/10.1016/j.ecss.2009.11.010, 2010.
Versteegh, G. J. M., Zonneveld, K. A. F., and de Lange, G. J.: Selective aerobic and anaerobic degradation of lipids and palynomorphs in the Eastern Mediterranean since the onset of sapropel S1 deposition, Mar. Geol., 278, 177–192, https://doi.org/10.1016/j.margeo.2010.10.007, 2010.
Walker, N. D., Wiseman Jr., W. J., Rouse Jr., L. J., and Babin, A.: Effects of river discharge, wind stress, and slope eddies on circulation and the satellite-observed structure of the Mississippi River plume, J. Coast. Res., 21, 1228–1244, https://doi.org/10.2112/04-0347.1, 2005.
Wall, D. and Dale, B.: The “Hystrichosphaerid” resting spore of the dinoflagellate Pyrodinium bahamense, Platf, 1906, J. Phycol., 5, 140–149, https://doi.org/10.1111/j.1529-8817.1969.tb02595.x, 1969.
Willard, D. A., Bernhardt, C. E., Weimer, L., Cooper, S. R., Gamez, D., and Jensen, J.: Atlas of pollen and spores of the Florida Everglades, Palynology, 28, 175–227, https://doi.org/10.2113/28.1.175, 2004.
Williams, G., Fensome, R., and McRae, R.: The Lentin and Williams Index of Fossil Dinoflagellates, 2017 Edn., American Association of Stratigraphic Palynologists Foundation , Contribution Series, AASP Contributions Series Number 48, ISSN 0160-8843, 2017.
Wood, G.: Palynological techniques-processing and microscopy, Chap. 3, in: Palynology: Principles and Application, American association of stratigraphic palynologists foundation, edited by: Jasonius, J. and McGregor, D. C., Dallas, TX, 1, 29–50, 1996.
Yao, Q., Liu, K., Aragón-Moreno, A. A., Rodrigues, E., Xu, Y. J., and Lam, N. S.: A 5200-year paleoecological and geochemical record of coastal environmental changes and shoreline fluctuations in southwestern Louisiana: Implications for coastal sustainability, Geomorphology, 365, 107284, https://doi.org/10.1016/j.geomorph.2020.107284, 2020.
Yedema, Y. W., Sangiorgi, F., Sluijs, A., Sinninghe Damsté, J. S., and Peterse, F.: Soil-, fluvial-, plant- and marine derived organic carbon from surface sediments (0–2 cm) from the northern Gulf of Mexico, PANGAEA [data set], https://doi.org/10.1594/PANGAEA.944838, 2022.
Yedema, Y. W., Sangiorgi, F., Sluijs, A., Sinninghe Damsté, J. S., and Peterse, F.: The dispersal of fluvially discharged and marine, shelf-produced particulate organic matter in the northern Gulf of Mexico, Biogeosciences, 20, 663–686, https://doi.org/10.5194/bg-20-663-2023, 2023.
Zheng, Z., Yang, S., Deng, Y., Huang, K., Wei, J., Berne, S., and Suc, J.-P.: Pollen record of the past 60 ka BP in the Middle Okinawa Trough: Terrestrial provenance and reconstruction of the paleoenvironment, Palaeogeogr. Palaeocl., 307, 285–300, https://doi.org/10.1016/j.palaeo.2011.05.026, 2011.
Zonneveld, K. A. and Pospelova, V.: A determination key for modern dinoflagellate cysts, Palynology, 39, 387–409, https://doi.org/10.1080/01916122.2014.990115, 2015.
Zonneveld, K. A., Versteegh, G. J., and de Lange, G. J.: Palaeoproductivity and post-depositional aerobic organic matter decay reflected by dinoflagellate cyst assemblages of the Eastern Mediterranean S1 sapropel, Mar. Geol., 172, 181–195, https://doi.org/10.1016/S0025-3227(00)00134-1, 2001.
Zonneveld, K. A., Versteegh, G., and Kodrans-Nsiah, M.: Preservation and organic chemistry of Late Cenozoic organic-walled dinoflagellate cysts: A review, Mar. Micropaleontol., 68, 179–197, https://doi.org/10.1016/j.marmicro.2008.01.015, 2008.
Zonneveld, K. A., Chen, L., Möbius, J., and Mahmoud, M. S.: Environmental significance of dinoflagellate cysts from the proximal part of the Po-river discharge plume (off southern Italy, Eastern Mediterranean), J. Sea Res., 62, 189–213, https://doi.org/10.1016/j.seares.2009.02.003, 2009.
Zonneveld, K. A. F., Versteegh, G. J. M., Kasten, S., Eglinton, T. I., Emeis, K.-C., Huguet, C., Koch, B. P., de Lange, G. J., de Leeuw, J. W., Middelburg, J. J., Mollenhauer, G., Prahl, F. G., Rethemeyer, J., and Wakeham, S. G.: Selective preservation of organic matter in marine environments; processes and impact on the sedimentary record, Biogeosciences, 7, 483–511, https://doi.org/10.5194/bg-7-483-2010, 2010.
Zonneveld, K. A. F., Marret, F., Versteegh, G. J. M., Bogus, K., Bonnet, S., Bouimetarhan, I., Crouch, E., de Vernal, A., Elshanawany, R., Edwards, L., Esper, O., Forke, S., Grøsfjeld, K., Henry, M., Holzwarth, U., Kielt, J.-F., Kim, S.-Y., Ladouceur, S., Ledu, D., Chen, L., Limoges, A., Londeix, L., Lu, S.-H., Mahmoud, M. S., Marino, G., Matsouka, K., Matthiessen, J., Mildenhal, D. C., Mudie, P., Neil, H. L., Pospelova, V., Qi, Y., Radi, T., Richerol, T., Rochon, A., Sangiorgi, F., Solignac, S., Turon, J.-L., Verleye, T., Wang, Y., Wang, Z., and Young, M.: Atlas of modern dinoflagellate cyst distribution based on 2405 data points, Rev. Palaeobot. Palyno., 191, 1–197, https://doi.org/10.1016/j.revpalbo.2012.08.003, 2013.
Zweng, M., Seidov, D., Boyer, T., Locarnini, M., Garcia, H., Mishonov, A., Baranova, O., Weathers, K., Paver, C., and Smolyar, I.: World ocean atlas 2018, Vol. 2, Salinity, NOAA Atlas NESDIS 82, 50 pp., https://archimer.ifremer.fr/doc/00651/76339/ (last access: 24 November 2023), 2019.
Zwiep, K. L., Hennekam, R., Donders, T. H., Van Helmond, N. A., De Lange, G. J., and Sangiorgi, F.: Marine productivity, water column processes and seafloor anoxia in relation to Nile discharge during sapropels S1 and S3, Quaternary Sci. Rev., 200, 178–190, https://doi.org/10.1016/j.quascirev.2018.08.026, 2018.