the Creative Commons Attribution 4.0 License.
the Creative Commons Attribution 4.0 License.
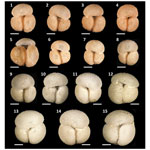
Globigerinoides rublobatus – a new species of Pleistocene planktonic foraminifera
Marcin Latas
Paul N. Pearson
Christopher R. Poole
Alessio Fabbrini
Bridget S. Wade
We describe Globigerinoides rublobatus n. sp., a new morphospecies of fossil planktonic foraminifera, from the Pleistocene sediments (∼810 ka) of the Indian Ocean and Pacific Ocean. We use image analysis and morphometry of 860 specimens from International Ocean Discovery Program Site U1483 in the tropical Indian Ocean to document morphological variability in the new morphospecies and related taxa, and we also report it from Pacific Ocean Site U1486 for the first time. The new morphospecies combines characteristics typical of Globigerinoides conglobatus (Brady, 1879) and Globigerinoides ruber (d'Orbigny, 1839), with which it co-occurs, but is distinct from both. Morphometric data indicate that G. rublobatus n. sp. is closer to G. conglobatus, potentially signalling an evolutionary affinity. We find that Globigerinoides rublobatus n. sp. occurs as two variants, a pigmented (pink) form and a non-pigmented (white) form. The non-pigmented forms are on average ∼50 % larger than the pigmented forms. This is so far only the third instance of fossil planktonic foraminifera known to exhibit this pink pigmentation. We regard the pink and white forms as variants of a single morphospecies and suggest the pink form may represent a later evolutionary adaptation.
- Article
(21087 KB) - Full-text XML
- BibTeX
- EndNote
Planktonic foraminifera are free-floating, heterotrophic marine protists that live in the open ocean where they populate the upper part of the water column (Hemleben et al., 1989; Schiebel and Hemleben, 2005, 2017). They produce calcium carbonate shells (tests) which on death sink through the water column and can accumulate on the sea floor. The evolution and extinction events of fossil species are extensively used by biostratigraphers to date and correlate marine sediments (e.g. Berggren et al., 1995; Wade et al., 2011; King et al., 2020; Raffi et al., 2020), and the tests are frequently used in palaeoceanography because they hold information about past oceanic environments. Systematic taxonomy within the group relies almost exclusively on shell morphology. Early micropalaeontological studies carried out by the nineteenth century pioneers (e.g. d'Orbigny, 1826, 1839; Brady, 1879) became a foundation for a wide field of research, with extensive and ongoing efforts to revise, unify and standardise the taxonomy (e.g. Kennett and Srinivasan, 1983; Pearson et al., 2006a; Huber et al., 2016; Wade et al., 2018a).
The genus Globigerinoides was erected by Cushman (1927) for species of planktonic foraminifera possessing multiple and supplementary apertures on the spiral side and has been greatly revised since. The most recent revision restricted the genus to the three modern species G. ruber, G. conglobatus and G. elongatus together with their ancestral clade back to the latest Oligocene when supplementary apertures in the group first appeared (Spezzaferri et al., 2015). It is one of the most common and diverse genera throughout most of this range (Spezzaferri et al., 2018). Species of Globigerinoides tend to live in the mixed layer and host algal photosymbionts, and isotopic evidence suggests that most or all fossil species had similar modes of life (Keller, 1985). The type species G. ruber (d'Orbigny, 1839) ranges from the late Miocene to the modern day, characterised by three subspherical chambers in the final whorl and a large, symmetrical high-arched umbilical aperture centred over the two preceding chambers. A more asymmetrical form, Globigerinoides elongatus, which in the past was frequently confused with G. ruber, is now known to be a distinct species based on combined genetic and morphological evidence (Aurahs et al., 2011; Brummer and Kucera, 2022). Globigerinoides conglobatus (Brady, 1879) ranges from the late Miocene to the modern day, possessing 3.5 to 4 chambers in the final whorl with a distinctive flattened, bean-shaped final chamber, resulting in a low-arched aperture. Stainbank et al. (2018) recently named a new morphospecies G. eoconglobatus for the ancestral form which possesses looser coiling, a higher-arched aperture and a more inflated final chamber. The genus also encompasses a number of extinct lineages including Miocene to Pleistocene G. obliquus and G. extremus, which both exhibit a degree of oblique compression and flattening of the final chamber (Kennett and Srinivasan, 1983).
It is well known that Globigerinoides ruber occurs as both pink and white forms, which are genetically distinct (Darling et al., 1997, 1999; Darling and Wade, 2008; Aurahs et al., 2009, 2011; Morard et al., 2019). Thompson et al. (1979) showed that the pink chromotype is geographically restricted to the Atlantic Ocean and its marginal seas (Caribbean and Mediterranean Sea), having disappeared from the Indo-Pacific at approximately 120 ka. However, Bhattacharjee et al. (2013) reported pink specimens from surface sediments in the north-western Bay of Bengal (Indian Ocean), attributing its re-emergence to the number of climate-driven environmental changes in the area. Morard et al. (2019) recently assigned the pink and white variants to G. ruber ruber and G. ruber albus, respectively. We have chosen not to follow this taxonomic division at subspecies level for three main reasons. Firstly, while the two types do appear genetically distinct, it is conceivable that some members of the G. ruber ruber genotype may be found that lack pigmentation, and vice versa for G. ruber albus. Second, the pink pigmentation does not always survive in the fossil record, making the distinction operationally difficult in fossil samples. Third, the division would appear to set a precedent for other species that have pink and white forms, including that described herein. Hence, for simplicity we refer to the two chromotypes by their more traditional designation as G. ruber (pink) and G. ruber (white).
Amongst widely recognised planktonic foraminiferal taxa, only Globigerinoides ruber and Globoturborotalita rubescens are known to exhibit pink test pigmentation in some specimens (Hemleben et al., 1989; Saito et al., 1981; Huber et al., 2016; Schiebel and Hemleben, 2017). The substance responsible for the pink test pigmentation is still not well understood (Schiebel and Hemleben, 2017). Ecological studies on modern Globigerinoides ruber from plankton tows in the North Atlantic have revealed the presence of pheophytin pigment within analysed tests of the pink chromotype and determined that it tolerates higher temperatures (up to 28 ∘C) than the white form (Bé and Hamlin, 1967). Liquid chromatography of chlorophyll and carotenoid pigments detected a high concentration of peridinin (carotenoid pigment) in Globigerinoides ruber, which in conjunction with the identified presence of chlorophyll a and only a minor amount of its degradation product indicates that symbiotic dinoflagellates are the main source of pigments in this taxon (Knight and Mantoura, 1985). Genetic analyses of the small subunit ribosomal DNA (srDNA) material from the photosymbiotic dinoflagellates found in the pink chromotype of Globigerinoides ruber indicate that they belong to a single pelagic species (Pelagodinium béii); the same is also found in the white chromotype, as well as in other taxa like Globigerinoides conglobatus, Trilobatus sacculifer and Orbulina universa (Gast and Caron, 1996). Further analysis of rDNA in photosymbionts from hundreds of globally sampled individual hosts (Globigerinoides ruber, Orbulina universa and Trilobatus sacculifer) revealed great diversity within pelagic symbiotic dinoflagellates (Shaked and de Vargas, 2006). Study of photosymbiosis in Globigerinoides ruber by measuring active chlorophyll fluorescence with fast-repetition-rate fluorometry has shown that the pink chromotype is characterised by significantly higher potential photochemical efficiency (Fv Fm), which might indicate a higher level of host–symbiont interaction and a stronger symbiotic relationship than its white counterpart (Takagi et al., 2019).
On International Ocean Discovery Program (IODP) Expedition 363, two of us (PNP and CRP) served as shipboard biostratigraphers and observed an unusual pink-pigmented planktonic foraminifer in Pleistocene sediments from Indian Ocean Sites U1482 and U1483, the morphology of which does not fit within the description of any established morphospecies (Rosenthal et al., 2018b, 2018c). We referred to it as Globigerinoides sp. cf. conglobatus (pink) and pointed out that it combines characteristics typical of Globigerinoides conglobatus and Globigerinoides ruber (Fig. 1), suggesting a potential evolutionary relationship with either taxon (Rosenthal et al., 2018b, c). We use the term “mosaic morphology” for the mixture of traits typically associated with more than one distinct taxon. Here we present detailed morphometric and imaging analysis of foraminifera deposited during a short (∼10 kyr) interval of time at IODP Site U1483 focusing on three taxa (Globigerinoides conglobatus, Globigerinoides ruber and Globigerinoides sp. cf. conglobatus). Our aim is to establish morphological variability of this enigmatic form, document its pigmentation, and investigate its potential relationship with co-occurring Globigerinoides conglobatus and Globigerinoides ruber. We formally name the new taxon Globigerinoides rublobatus n. sp. in the “Systematic palaeontology” section below and use this name throughout the rest of this paper.
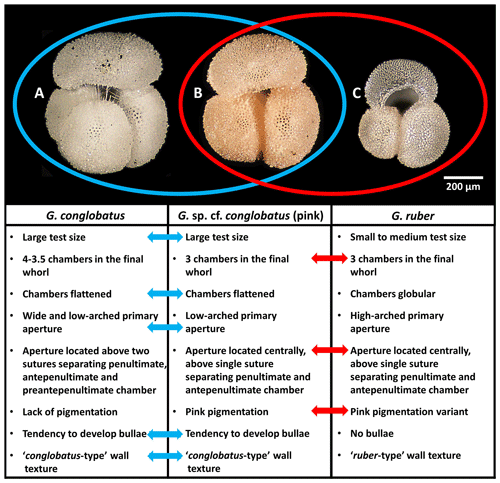
Figure 1Morphological comparison of Globigerinoides conglobatus, Globigerinoides sp. cf. conglobatus (Globigerinoides rublobatus n. sp. herein) (pink) and Globigerinoides ruber. All three specimens were picked from the same sample from Indian Ocean sample U1483A/9H/2/50–52 cm. Images of the specimens represent the following: (a) Globigerinoides conglobatus, (b) Globigerinoides sp. cf. conglobatus (pink), (c) Globigerinoides ruber. All presented specimens are curated at the Natural History Museum, London: (a) PF 75397; (b) PF 75381; (c) PF 75405.
2.1 Sample location
Taxonomic work was conducted on multiple specimens of Globigerinoides conglobatus, Globigerinoides ruber and Globigerinoides rublobatus n. sp. from three localities in the Indo-Pacific region (Fig. 2) drilled by IODP Expedition 363 (Rosenthal et al., 2018a). Pleistocene sediments from both Indian Ocean sites (U1482 and U1483) were reported by Rosenthal et al. (2018b, c) to contain an unusual-looking pink planktonic foraminifera (Fig. 1). These strange forms were identified in two consecutive samples from Site U1482 and only in a single horizon at Site U1483, providing a useful tie point between the two sedimentary sequences (Rosenthal et al., 2018b, c). In this study, we found the same species at a correlative level in Pacific Site U1486, greatly extending the known geographic range. Our morphometric analysis was conducted on the material from the IODP Site U1483, as it provided a continuous sedimentary record and the most reliable age control. Nine consecutive sediment samples from Pleistocene Subzone PT1a were selected in 10 cm increments from the core section with confirmed occurrences of this new pink planktonic foraminifera (Table 1).
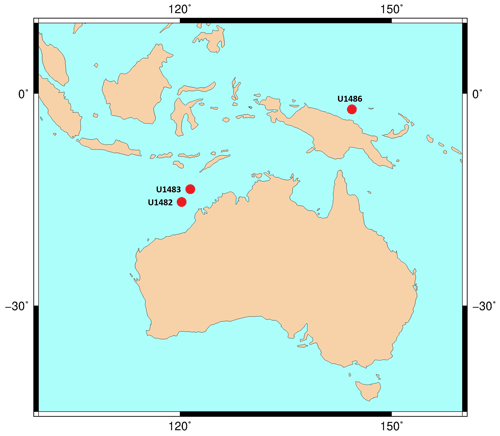
Figure 2Map of IODP sites from Expedition 363 and drill cores used for taxonomic description of G. rublobatus n. sp. The base contour map was produced using the Ocean Drilling Stratigraphic Network at https://www.odsn.de/ (last access: 7 June 2022).
2.2 Laboratory techniques
Sediments were washed over a 63 µm mesh to extract microfossil content and then oven-dried at temperatures below 40 ∘C. Sample residues were dry-sieved through 250 µm mesh to separate full-grown adult forms from smaller juvenile versions of larger taxa. This size selection approach ensured maximum confidence in species identification; however, it came at cost of sacrificing some adult forms of Globigerinoides ruber, which would normally represent the lower end (125–249 µm) of its typical adult size spectrum. This factor needs to be considered during morphometric analysis as it can potentially bias further interpretation of size-related data for this particular species.
Table 1List of sample material used in the morphometric analysis with their depths in metres below sea floor and sample age calibrations, calculated through linear interpolation between tie points of palaeomagnetic reversals at Hole U1483A from Rosenthal et al. (2018c) with updated chron ages after Raffi et al. (2020).
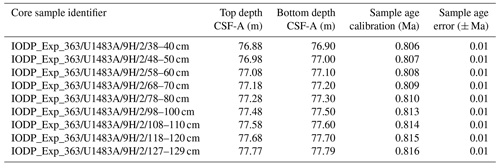
2.3 Data acquisition
A total of 860 specimens, representing the new species Globigerinoides rublobatus n. sp. (284 specimens) and its two potential sister taxa Globigerinoides conglobatus (288 specimens) and Globigerinoides ruber (288 specimens), were selected for morphometric analysis. Specimens of Globigerinoides rublobatus n. sp. were additionally split into pink and white varieties based on visual inspection of their test colour. Pink-pigmented specimens of Globigerinoides ruber occur only rarely in analysed sample residues and were not found amongst groups of randomly selected specimens of this taxon. All picked foraminifera were positioned in umbilical view and photographed with the use of Olympus SZX7 light microscope, equipped with a QImaging Retiga 2000R digital camera. Only well-preserved specimens were picked for analysis, and any broken or highly deformed forms such as extreme kummerform cases were ignored. Following recent studies (e.g. Brombacher et al., 2017, 2018; Poole and Wade, 2019; Pearson and Penny, 2021), morphometric data were acquired in Image Pro software using its semi-automatic functions for extracting dimensional data from images of photographed objects. No apertural measurements from imaged specimens were taken due to shell geometry. Although the high-arched primary aperture of G. ruber is easy to measure, performing equivalent analysis on G. rublobatus n. sp. and G. conglobatus would be very challenging because their primary aperture is effectively concealed within the umbilicus. Any attempt to acquire apertural shape data on those species would require internal data such as micro-CT scans, which are not feasible to conduct on a large number of specimens. Morphometric measurements were collected in eight different categories (area, mean diameter, perimeter, mean caliper, radius ratio, aspect ratio, circularity and roundness), which based on their specific character can be generally grouped into either size- or shape-related categories (Fig. 3). Any manual measurement of traits on the photographed or physical specimens leaves space for subjective human interpretation and as such is prone to influencing results. This issue can be addressed through application of automatic specimen detection and trait measurements, which should be carried out under fixed magnification and uniform light conditions (Brombacher et al., 2017). We reduced the risk of potential human-driven bias by using the adjustable brightness threshold function in Image Pro software, which allows automatic detection and delamination of bright objects that contrast with the darker background. In order to eliminate unwanted noise artefacts from the heterogenous cardboard background of standard micropalaeontological slides, we followed the technique of Brombacher et al. (2017), who proposed mounting foraminifera specimens on clear glass slides, which provides a homogenous dark background when subjected to direct light from above.
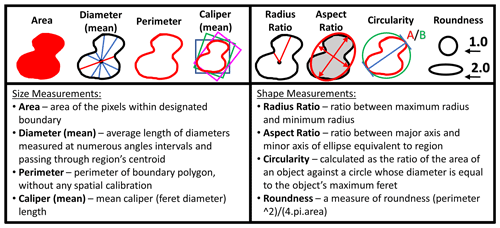
Figure 3Measurements taken in Image Pro software for morphometric analysis of picked planktonic foraminifera specimens.
Photographs were taken in moderate light to document the taxonomic identification of the analysed foraminifera, and then each specimen was also imaged in maximum light intensity, leading to overexposure to allow for precise tracing of test outlines and ensuring the consistency of measurements in Image Pro. The software was configured to ignore any internal holes (e.g. apertures) and automatically extract all required measurements (e.g. area, aspect ratio) from the highlighted objects (Fig. 3). Four specimens of the same taxon were photographed at one time in order to ensure efficiency of data collection and capture the relative morphological variability. Uniform magnification was used to ensure measurement consistency. A single specimen of a circular benthic foraminifera was repeatedly photographed and measured together with all the analysed specimens as a double-check to ensure consistency of magnification. Collected morphometric data were analysed in Microsoft Excel software, with the three-variable scatter diagrams plotted using the Scatterplot3D_2.1 Excel workbook by Doka (2013).
3.1 Pigmentation data analysis
Globigerinoides rublobatus n. sp. occurs as both pink and white forms (Figs. 4 and 5). Initial microscope observations of multiple specimens suggest that the two pigmentation variants also differ in size, with white forms being on average ∼50 % larger than their pink counterparts. Examination of nine consecutive core samples containing G. rublobatus n. sp. at IODP Site U1483 revealed that both chromotypes co-occur in the older part of the analysed interval. However, this pattern changes further up the core interval where the white forms gradually disappear, leaving only the pink variant present in the younger part of studied section (Fig. 6).
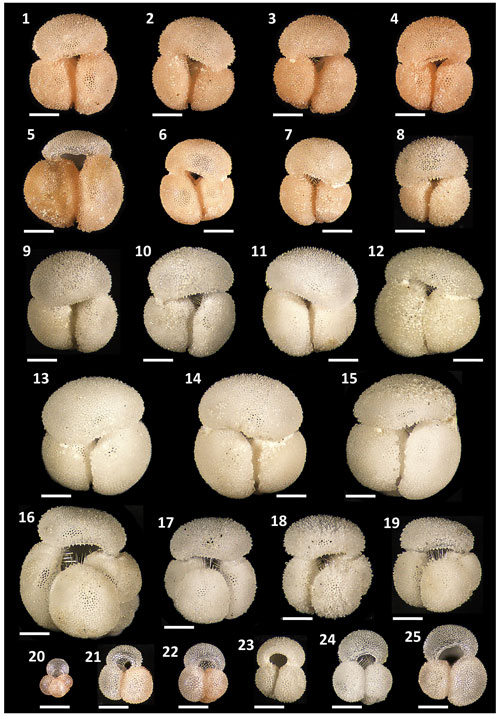
Figure 4Pigmentation variants and morphological diversity within Globigerinoides rublobatus n. sp. (1–15) in comparison to Globigerinoides conglobatus (Brady, 1879) (16–19), Globigerinoides ruber (d'Orbigny, 1839) (21–25) and Globoturborotalita rubescens (Hofker, 1956) (20). Specimens (1–8) represent G. rublobatus n. sp. (pink) and specimens (9–15) represent G. rublobatus n. sp. (white). Specimen sample origin: (1–7, 10, 16–25) sample U1483A/9H/2/50–52 cm; (8–9) sample U1486B/6H/4/7–9 cm; (11–15) sample U1482B/5H/CC/30–35 cm. All specimen photos are presented in relative proportions, with scale bars representing 200 µm. All presented specimens are curated at the Natural History Museum in London: (1) PF 75381; (2) PF 75382; (3) PF 75383; (4) PF 75384; (5) PF 75385; (6) PF 75386; (7) PF 75387; (8) PF 75388; (9) PF 75389; (10) PM PF 75390; (11) PF 75391; (12) PF 75392; (13) PF 75393; (14) PF 75394; (15) PF 75395; (16) PF 75396; (17) PF 75397; (18) PF 75398; (19) PF 75399; (20) PF 75400; (21) PF 75401; (22) PF 75402; (23) PF 75403; (24) PF 75404; (25) PF 75405.
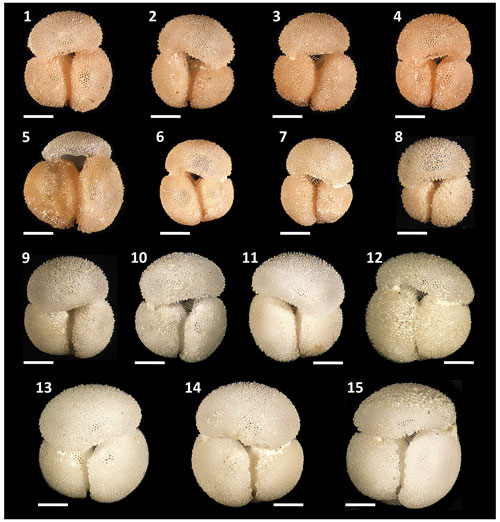
Figure 5Morphological diversity and pigmentation variants of Globigerinoides rublobatus n. sp. Specimens (1–8) represent G. rublobatus n. sp. (pink) and specimens (9–15) represent G. rublobatus n. sp. (white). Specimen sample origin: (1–7, 10) sample U1483A/9H/2/50–52 cm; (8–9) sample U1486B/6H/4/7–9 cm; (11–15) sample U1482B/5H/CC/30–35 cm. All specimen photos are presented in relative proportions, with scale bars representing 200 µm. All presented specimens are curated at the Natural History Museum, London: (1) PF 75381; (2) PF 75382; (3) PF 75383; (4) PF 75384; (5) PF 75385; (6) PF 75386; (7) PF 75387; (8) PF 75388; (9) PF 75389; (10) PF 75390; (11) PF 75391; (12) PF 75392; (13) PF 75393; (14) PF 75394; (15) PF 75395.
3.2 Morphometric data analysis
Three-dimensional data visualisations of the morphometric data are shown in Figs. 7 and 8, representing each type of measurement displayed on the three-variable (X–Y–Z) scatter plots. The cumulative dataset for each studied species presented in the form of box plot diagrams (Fig. 9) provides more detailed insight into the statistical distribution of collected data in every measured category. A further breakdown of this dataset, presented on the series of diagrams with each box plot representing individual samples (Figs. 10 and 11), allows better visual changes in data distribution and any potential evolutionary trends of studied taxa. This three-tier system applied for data visualisation is functionally complementary and reveals different aspects of the collected dataset, presenting it in a logical and easy-to-follow order.
Shape- and size-related measurements plotted against each other in various configurations always show similar patterns (Figs. 7 and 8), with a data cluster representing G. rublobatus n. sp. (pink) able to be distinguished from partly overlapping G. ruber and G. conglobatus and generally falling between these two datasets. At the same time G. rublobatus n. sp. (white) data seem to have a better overall fit with the cluster representing G. conglobatus. The same relationships can be observed when examining combined morphometric data within each of the separate measurement categories (Fig. 9). The collection of size-related measurements taken from 284 specimens of G. rublobatus n. sp. confirms initial microscope observations and shows significant size disparity between the two colour variants; white specimens are consistently larger than their pink counterparts and match co-occurring specimens of G. conglobatus in size (Fig. 9). Mean size values for the pink chromotype of G. rublobatus n. sp. are lowest in the oldest part of the analysed sedimentary sequence and increase further up the section, approaching mean values recorded for G. conglobatus (Fig. 10). Analysis of combined shape-related data in each category shows no major differences between the two colour variants of G. rublobatus n. sp. and G. conglobatus (Fig. 9). Further breakdown into individual samples analysed throughout the record presents an initial degree of overlap of G. rublobatus n. sp. and G. conglobatus morphometric data, followed by progressing slow separation of the two datasets in the later part of the section (Fig. 11).
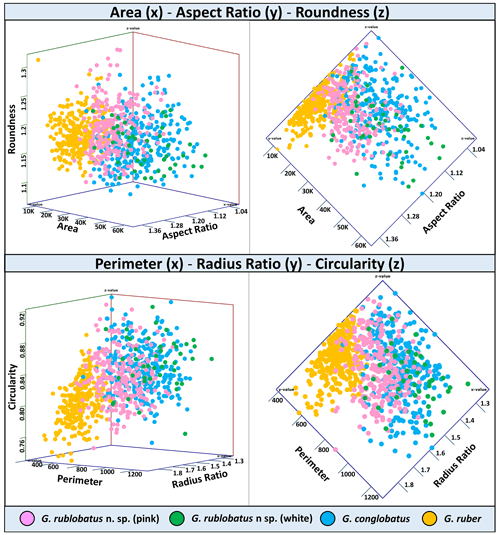
Figure 7Three-variable scatter plots with morphometric measurements of analysed taxa from the IODP Site U1483.
Co-occurrence of pink forms of both G. rublobatus n. sp. and G. ruber throughout the studied record could theoretically suggest the former to be a local ecophenotypic variant of the latter. We rule out such a possibility as our data show significant differences between them, while at the same time indicating a good morphological match between the two colour variants of G. rublobatus n. sp., suggesting their close affinity. Both chromotypes of G. rublobatus n. sp. have typically large compacted tests with flattened ultimate and penultimate chambers, wide and low-arched architecture of the primary aperture, and a tendency to develop bullae. This shared matrix of morphological characteristics makes G. rublobatus n. sp. distinctively different from G. ruber (Figs. 1 and 4), which typically exhibits a medium-sized test with subspherical chambers and characteristic high-arched primary aperture. These qualitative morphological observations are in many respects supported by the quantitative evidence from morphometric analysis, showing consecutively good separation between plotted clusters for both taxa (Figs. 7 and 8). Size data distribution shows that some individual specimens of G. ruber, representing the maximum end of its size range, approach mean size values for G. rublobatus n. sp. (pink) in all measured categories (Fig. 9), but the size difference between the two taxa is striking when comparing their mean area size values (227 417 µm2 for the pink variant of G. rublobatus n. sp. as opposed to only 113 233 µm2 for G. ruber). Furthermore, maximum size values of G. rublobatus n. sp. (pink) plot well outside any of our recorded data for G. ruber and at the same time fall within the upper 25 % data range of size measurements for G. rublobatus n. sp. (white) and G. conglobatus (Fig. 9). This agrees with the size distinction for G. ruber and G. conglobatus of Kennett and Srinivasan (1983), who described the former as medium and the latter as large. Analysis of shape-related data also indicates that both chromotypes of G. rublobatus n. sp. and G. conglobatus differ significantly from G. ruber (Fig. 9). This is no surprise as the subspherical architecture of G. ruber chambers results in a more lobate test outline compared to that of both G. rublobatus n. sp. and G. conglobatus, which are characterised by radially compressed chambers and consequentially by a more compacted test.
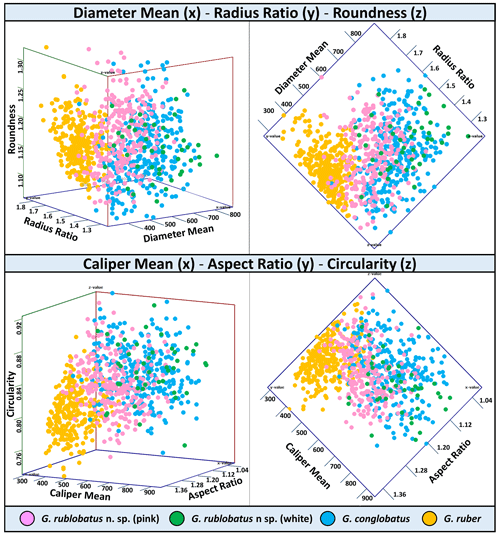
Figure 8Three-variable scatter plots with morphometric measurements of analysed taxa from the IODP Site U1483.
Combined evidence from morphological observations and morphometric data indicates that the planktonic foraminifera formerly described as Globigerinoides sp. cf. conglobatus (Rosenthal et al., 2018b, c) are distinct from both G. conglobatus and G. ruber and require erecting a new morphospecies, Globigerinoides rublobatus n. sp. In our opinion, the observed size disparity between pink and white morphotypes of G. rublobatus n. sp. might suggest that both colour variants could represent two coexisting but distinctively separate genotypes or even separate evolutionary lineages. Modern G. ruber is a good analogue where there is a slight size disparity between pink and white variants, and the former grows about 50 µm larger than the latter (Schiebel and Hemleben 2017). A recent study by Morard et al. (2019) has shown that despite general morphological similarity, the “chromotypes” of G. ruber significantly diverge genetically. As genetic information is not available for fossil species, we regard the pink and white forms as variations within a single morphospecies. Morphometric data (Figs. 7–11) indicate that G. rublobatus n. sp. is most closely related to G. conglobatus, potentially indicating evolutionary affinity, whereas the pink variant of G. rublobatus n. sp. may represent a later evolutionary adaptation. Further studies on G. rublobatus n. sp. are necessary to test this hypothesis.
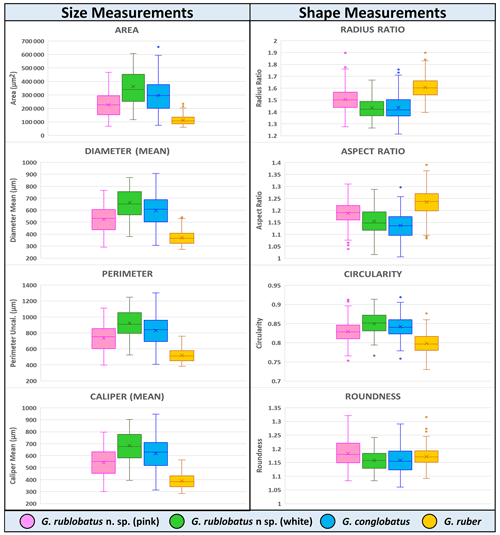
Figure 9Collective morphometric data for individual measurements representing analysed taxa from the IODP Site U1483. Mean values marked with X; dots are outliers.
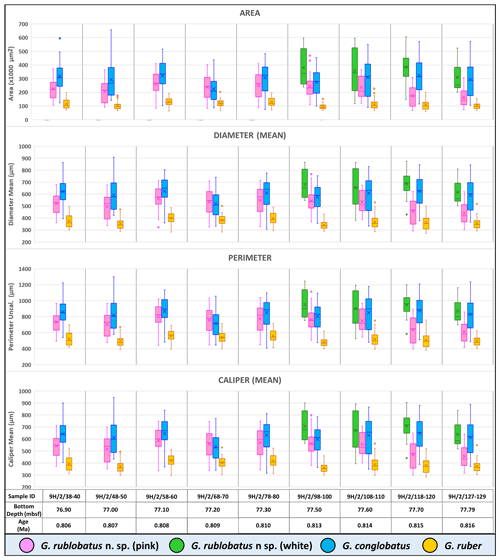
Figure 10Box plots of size-related morphometric measurements taken from analysed species from Site U1483 samples. Mean values marked with X; dots are outliers.
-
Order Foraminiferida d'Orbigny, 1826
-
Superfamily Globigerinoidea Carpenter, Parker and Jones, 1862
-
Family Globigerinidae Carpenter, Parker and Jones, 1862
-
Genus Globigerinoides Cushman 1927, amended by Spezzaferri et al., 2015
-
Type species: Globigerina rubra d'Orbigny, 1839
-
Globigerinoides rublobatus n. sp.
(Fig. 1b referred to as Globigerinoides. sp. cf. conglobatus (pink), Figs. 4.1–4.15, Figs. 5.1–5.15 and Figs. 12–15)
-
? Globigerinoides gomitulus (Seguenza) – Kaneps, 1973, p. 734 (no figures).
-
? Globigerinoides gomitulus (Seguenza) – (plesiotype) – Saito, Thompson and Breger, 1981 p. 57, pl. 14, fig. 2a–d.
-
Globigerinoides gomitulus (Seguenza) pink-coloured variety – Beiersdorf, Bickert, Cepek, Fenner, Petersen, Schönfeld, Weiss and Won, 1995, p. 48, pl. 3, figs. 4–6.
-
Globigerinoides sp. cf. conglobatus (pink) – Rosenthal, Holbourn, Kulhanek, Aiello, Babila, Bayon, Beaufort, Bova, Chun, Dang, Drury, Dunkley Jones, Eichler, Fernando, Gibson, Hatfield, Johnson, Kumagai, Li, Linsley, Meinicke, Mountain, Opdyke, Pearson, Poole, Ravelo, Sagawa, Schmitt, Wurtzel, Xu, Yamamoto, and Zhang, 2018b, p. 18, fig. 17.
-
Not Globigerinoides gomitulus Seguenza, 1880, pl. XVII, fig. 16 (Pliocene section in Riace, Calabria region, southern Italy) (inadequate illustration; no type specimen designated; neotype designated by Mistretta 1962, p. 202, fig. 1 (Globigerinoides elongatus) subsequently lost from the micropalaeontological collection of the Institute of Geology and Paleontology at the University of Palermo).
5.1 Etymology
The species name rublobatus reflects the reddish pigmentation seen in some specimens (ruber is Latin for “red”) and the lobate outline; it is also a contraction of two other Globigerinoides species names, ruber and conglobatus, to reflect the mosaic morphology of the newly erected species. We thank IODP Expedition 363 scientist Kelly Gibson for the suggestion.
5.2 Diagnosis
Large test with distinctly flattened ultimate and penultimate chambers. Primary aperture elongated, low-arched and positioned over the single suture, which separates the penultimate and antepenultimate chamber. The morphospecies occurs in two pigmentation variants (chromotypes) represented by pink- and white-coloured forms.
5.3 Holotype
Holotype specimen of Globigerinoides rublobatus n. sp. (Figs. 1b, 4.1, 5.1 and 12) is curated at the Natural History Museum (NHM), London, United Kingdom (PF 75381). Before deposition in the museum collection, holotype was CT-scanned to produce its detailed digital 3D model, allowing for extraction of comprehensive morphological data and providing an efficient method for future data curation of the newly designated species. Regrettably, the original pink pigmentation of the holotype specimen was accidently removed in the scanning process, most likely due to prolonged exposure to X-ray radiation, resulting in the classic white colouration of a calcite foraminifera test. Fortunately, the true nature of the holotype pink pigmentation is preserved in the form of photographic evidence presented in this paper (Figs. 1b, 4.1, 5.1 and 12).
5.4 Paratypes
We have selected both pink and white specimens as paratypes as we consider them to be variants within the same morphospecies. All 14 paratype specimens of Globigerinoides rublobatus n. sp. are curated at the Natural History Museum (NHM), London, United Kingdom.
Figures 4.2, 5.2 and 13.1a–d – PF 75382; Figs. 4.3, 5.3 and 13.2a–d – PF 75383; Figs. 4.4, 5.4 and 13.3a–d – PF 75385; Figs. 4.5, 5.5 and 13.4a–d – PF 75385; Figs. 4.6, 5.6 and 13.5a–d – PF 75386; Figs. 4.7, 5.7 and 14.1a–d – PF 75387; Figs. 4.8, 5.8 and 14.2a–d – PF 75388; Figs. 4.9, 5.9 and 14.3a–d – PF 75389; Figs. 4.10, 5.10 and 14.4a–d – PF 75390; Figs. 4.11, 5.11 and 14.5a–d – PF 75391; Figs. 4.12, 5.12 and 15.1a–d – PF 75392; Figs. 4.13, 5.13 and 15.2a–d – PF 75393; Figs. 4.14, 5.14 and 15.3a–d – PF 75394; Figs. 4.15, 5.15 and 15.4a–d – PF 75395.
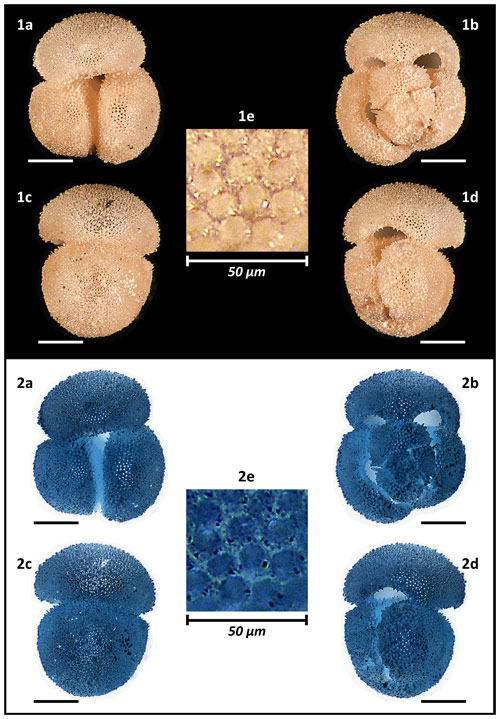
Figure 12Holotype of Globigerinoides rublobatus n. sp. (NHM collection number PF 75381). The specimen comes from Indian Ocean IODP Site U1483 and was picked from the sample U1483A/9H/2/50–52 cm, Planktonic Foraminifera Subzone PT1a. All images representing the whole specimen (1a–d and 2a–d) are presented in the same scale, with associated scale bars representing 200 µm. Images (1e and 2e) show the magnified test wall structure of the specimen. (1a–e) Light microscope photos, (2a–e) negative images.
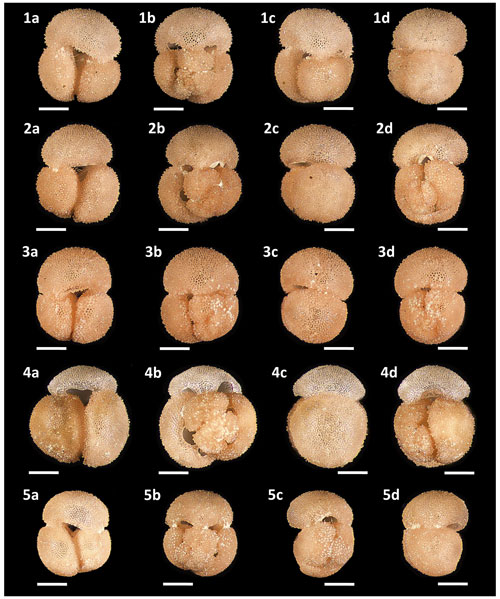
Figure 13Light microscope images of paratypes of Globigerinoides rublobatus n. sp. Each specimen (1–5) is presented in four positions: (a) apertural view, (b) spiral view and (c, d) side views (left and right profile, respectively). All shown specimens were picked from the same sample (U1483A/9H/2/50–52 cm) and specimen photos are presented in relative proportions, with all scale bars representing 200 µm. All presented specimens are curated at the Natural History Museum, London: (1a–d) PF 75382; (2a–d) PF 75383; (3a–d) PF 75384; (4a–d) PF 75385; (5a–d) PF 75386.
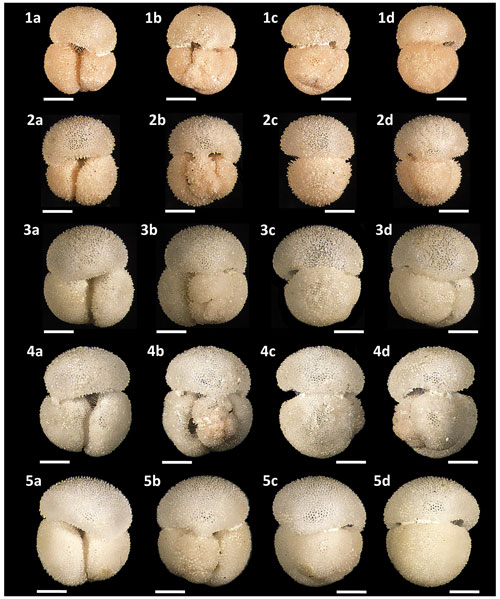
Figure 14Light microscope images of paratypes of Globigerinoides rublobatus n. sp. Each specimen (1–5) is presented in four positions: (a) apertural view, (b) spiral view and (c, d) side views (left and right profile, respectively). All specimen photos are presented in relative proportions, with all scale bars representing 200 µm. Specimen sample origin: (1, 4) sample U1483A/9H/2/50–52 cm; (2, 3) sample U1486B/6H/4/7–9 cm; (5) sample U1482B/5H/CC/30–35 cm. All presented specimens are curated at the Natural History Museum, London: (1a–d) PF 75387; (2a–d) PF 75388; (3a–d) PF 75389; (4a–d) PF 75390; (5a–d) PF 75391.
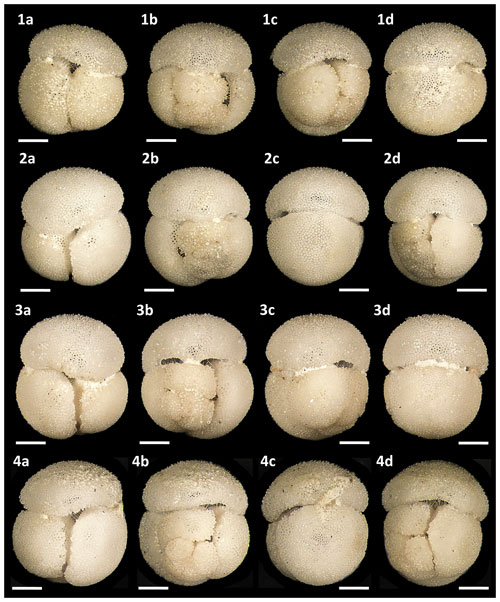
Figure 15Light microscope images of paratypes of Globigerinoides rublobatus n. sp. Each specimen (1–4) is presented in four positions: (a) apertural view, (b) spiral view and (c, d) side views (left and right profile, respectively). All shown specimens were picked from the same sample (U1482B/5H/CC/30–35 cm) and specimen photos are presented in relative proportions, with all scale bars representing 200 µm. All presented specimens are curated at the Natural History Museum, London: (1a–d) PF 75392; (2a–d) PF 75393; (3a–d) PF 75394; (4a–d) PF 75395.
5.5 Material
The collection of 15 type specimens representing the adult form of both pink and white variants of G. rublobatus n. sp. was selected from core samples recovered from IODP Sites U1482, U1483 and U1486. Morphometric study of G. rublobatus n. sp. was carried out on a series of 284 specimens picked from nine consecutive core samples from the IODP Site U1483 (Table 1).
5.6 Type locality and horizon
All 15 type specimens were obtained from the sedimentary cores recovered from the Indo-Pacific region by IODP Expedition 363. The holotype (Fig. 12) and seven paratypes (Figs. 13.1a–d–13.5a–d, 14.1a–d and 14.4a–d) were all picked from the sample U1483A/9H/2/50–52 cm, which derives from the sedimentary core recovered from a locality on the Scott Plateau off the north-western margin of Australia (eastern Indian Ocean) (13∘05.24′ S, 121∘48.24′ E). Five specimens from the paratype series (Figs. 14.5a–d and 15.1a–d–15.4a–d) were sourced from the sample U1482B/5H/CC/30–35 cm, which came from the sedimentary core recovered from the IODP Hole U1482B, also located on the Scott Plateau (15∘03.31′ S, 120∘26.10′ E) and positioned ∼142 nmi south-west from the IODP Site U1483. The remaining two paratypes (Fig. 14.2a–d–14.3a–d) were picked from the sample U1486B/6H/4/7–9 cm, derived from the IODP Hole U1486B (02∘22.34′ S, 144∘36.08′ E) located in the Manus Basin off the Papua New Guinea northern margin (western Pacific Ocean).
5.7 Age
The holotype specimen and series of paratypes are all of Pleistocene age (Planktonic Foraminifera Subzone PT1a).
5.8 Description
The test is large, compact and tightly coiled in a trochospiral manner, giving it a globular to subglobular overall morphology. The outline in the equatorial view ranges in shape from subquadrate to subcircular. The final whorl consists of three chambers, gradually increasing in size as added, each progressively more radially compressed than the previous one. The umbilicus is narrow, and sutures are slightly curved, deeply incised and well defined. The primary aperture is umbilical, elongated and low-arched with a thin lip. Usually, it is positioned centrally over the suture separating penultimate and antepenultimate chambers, but in some cases it is off-centre, which tends to be more pronounced in the largest specimens of the white variant. Several supplementary apertures are located at sutural junctions of the spiral side. The test is spinose with the surface perforated and a cancellate wall texture. Globigerinoides rublobatus n. sp. occurs in two pigmentation variants, represented by pink and white forms. Apart from the obvious difference in colouration, some other morphological differences can be observed between the variants. White forms are generally around 50% larger than their pink counterparts; the mean area size value for the former is 362 701 µm2 as opposed to 227 417 µm2 in the case of the latter (Fig. 9). Qualitative observations of broken specimens suggest that their tests may also have thicker walls, with well-developed gametogenic calcite crust in some of the largest specimens (Figs. 4.13–4.15, 15.2–15.4 and 5.13–5.15), which Hemleben et al. (2018) define as “conglobatus-type” wall texture. Accessory apertures on the spiral side of the white specimens are typically narrower and more elongated than those in pink specimens, in which they are more rounded and widely open. We regard these minor morphological differences as representing variation within a single morphospecies.
5.9 Dimensions
The holotype has a maximum diameter of 685 µm, which falls within the typical size range for an adult form of the pink-pigmented variant. White-coloured forms of this morphospecies are on average ∼50 % larger, with some extremely large specimens reaching almost 1000 µm in diameter.
5.10 Distribution
Morphospecies presence confirmed so far in the core samples from the eastern Indian Ocean (IODP Sites U1482 and U1483) and western Pacific Ocean (IODP Site U1486) (Fig. 2).
5.11 Remarks
Globigerinoides rublobatus n. sp. differs from Globigerinoides ruber (d'Orbigny, 1839) by having on average a much larger test (Fig. 9) with distinctively flattened chambers (especially ultimate and penultimate chambers) and by possessing a low-arched and elongated primary aperture, which can in some cases be slightly offset from the centre. It can be distinguished from Globigerinoides conglobatus (Brady, 1879) by having only 3 chambers in the final whorl, with a primary aperture positioned over the single suture that separates the penultimate and antepenultimate chamber, and by also occurring as a pink variant in addition to regular white forms. Unexpected holotype specimen bleaching during laboratory analyses indicates that pigment is still chemically active and could be potentially extracted from fossil specimens, which provides the opportunity for future studies of its nature and chemical composition.
5.12 Phylogenetic relationship
Our study suggests that G. rublobatus n. sp. shares more similarities with G. conglobatus than with G. ruber, which is supported by combined evidence from morphological observations (e.g. large test, characteristic flattening of the final chamber and shape of the primary aperture) and collected morphometric data recording the size and shape of the test (Figs. 7–11). It is likely that G. rublobatus n. sp. evolved from G. conglobatus, but the transition has not been observed.
5.13 Taxonomic discussion
Specimens of Globigerinoides rublobatus n. sp. may have been seen by previous authors from across the Indo-Pacific region (Table 2; Fig. 16) but assigned to established morphospecies. Observations of specimens fitting within the taxonomic concept of G. rublobatus n. sp., but referred to as Globigerinoides gomitulus (Seguenza), were made from Plio-Pleistocene sediments of DSDP Sites 157 and 158 in the Panama Basin of the eastern equatorial Pacific (Kaneps, 1973) and from DSDP Site 264 on the south-western Australian margin (Kaneps, 1975). Although no images of these G. gomitulus specimens were provided, Kaneps (1973) described his specimens as having a large test and granular surface like G. conglobatus, but with the aperture positioned in a G. ruber manner and located over the suture separating penultimate and antepenultimate chamber, although often shifted to the left. This account of what we call mosaic morphology corresponds very well to our taxonomic concept of G. rublobatus n. sp. and agrees with our observation of the primary aperture being off-centred in some specimens, which is more pronounced in the large specimens of the white variant (e.g. Fig. 5.11, 5.13–5.15).
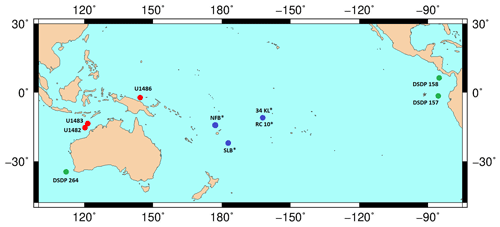
Figure 16Map showing IODP Exp. 363 sites (red marker) with a proven presence of G. rublobatus n. sp., sites drilled by the German vessel RV Sonne (blue marker) with the reported presence of G. gomitulus (pink variant) and DSDP sites with the described presence of G. gomitulus (green marker). An asterisk symbol (*) by the label name indicates the presence of multiple sites in the small area (e.g. NFB and SLB) or that two different sites are in close vicinity to each other (e.g. 34KL and RC 10). The base contour map was produced using the Ocean Drilling Stratigraphic Network: https://www.odsn.de/ (last access: 7 June 2022).
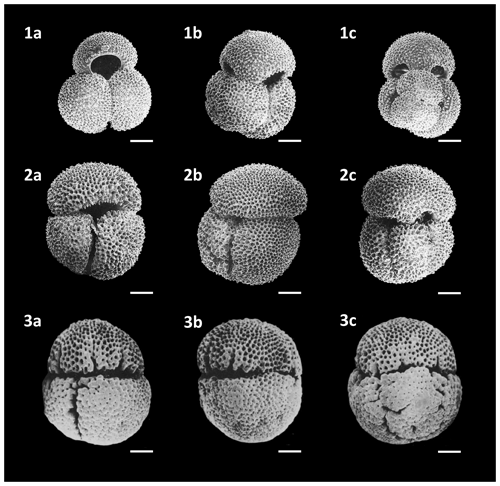
Figure 17(1a–c) G. ruber (d'Orbigny) (pink variety) illustrated by Beiersdorf et al. (1995), (2a–c) G. rublobatus n.sp. illustrated by Beiersdorf et al. (1995) and originally designated by the authors as G. gomitulus (Seguenza 1880) (pink variety). (3a–c) Plesiotype specimen of G. gomitulus (Seguenza, 1880) illustrated by Saito et al. (1981). The plesiotype specimen (3a–c) originally illustrated by Saito et al. (1981) was presented without the scale bar, stating the magnification as 71 times; we used this information to recalculate the original dimensions of the specimen and produce a scale bar. All specimen images on this plate are presented in relative proportions, with all scale bars representing 100 µm.
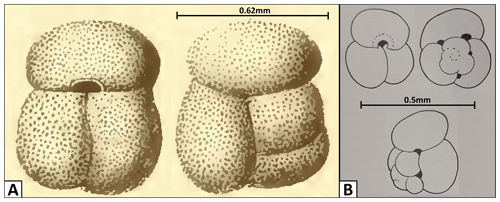
Figure 18Changes in the G. gomitulus taxonomic concept. (a) Holotype by Seguenza (1880), (b) neotype designated by Mistretta (1962). The holotype (a) illustrated by Seguenza (1880) was originally presented without the scale bar, but the author provided precise specimen measurements, allowing us to add the scale bar presented here.
Planktonic foraminifera referred to as a pink-coloured variant of Globigerinoides gomitulus were found in Pleistocene sediments of sites in the Lau and North Fiji basins (Riech, 1990; Von Daniels, 1990) and at the Manihiki Plateau (Beiersdorf et al., 1995), which were all drilled by the RV Sonne during scientific expeditions SO-35 and SO-67-1, respectively. These authors did not describe these forms, but Beiersdorf et al. (1995) photographed a pink-coloured variety of G. ruber (d'Orbigny) (Fig. 17.1a–c) alongside an exemplary specimen of what they called a pink-coloured variety of Globigerinoides gomitulus (Seguenza) (Fig. 17.2a–c), which we consider to be G. rublobatus n. sp. Von Daniels et al. (1990) and Beiersdorf et al. (1995) followed the diagnosis of G. gomitulus provided by Saito et al. (1981), who described his plesiotype specimen from Pleistocene sediments of the Manihiki Plateau (South Pacific). Saito et al. (1981) mentioned that specimens of G. gomitulus found at his plesiotype locality possess light pink colouration, which matches later observations by Beiersdorf et al. (1995) from Site 34KL, located just ∼75 km away. According to Saito et al. (1981), G. gomitulus (Seguenza) looks comparable to G. conglobatus but has closely packed chambers and smaller overall size. A similar opinion was also expressed by Kaneps (1973), who suggested that Globigerinoides gomitulus (Seguenza) is probably often combined with Globigerinoides conglobatus (Brady) due to the great similarities in test morphology and wall structure between the two species. Based on the presented cumulative evidence, we conclude that morphotypes reported as G. gomitulus in the mentioned studies (Kaneps, 1973; Beiersdorf et al., 1995) are consistent with G. rublobatus n. sp. described here. However, the plesiotype imaged by Saito et al. (1981), with its extremely compacted appearance and highly obscured primary aperture (Fig. 17.3a–c), is not typical of our taxonomic concept of G. rublobatus n. sp. At the same time, we doubt that these specimens could represent G. gomitulus (Seguenza), whose taxonomic concept itself is very problematic, as it appears that it has changed significantly over time.
The species Globigerina gomitulus was named by Seguenza (1880) from the Pliocene section in Riace (Calabria, Italy), who provided only a brief description, stating that it could be distinguished by having compressed non-globular chambers and separated by not very pronounced sutures, which are arranged orthogonally. Seguenza (1880) illustrated a specimen, giving a rough idea of the appearance, with three radially compressed chambers in the final whorl, but with the pattern of earlier chambers obscure or poorly depicted. Fornasini (1898) and Silvestri (1898) reported the species in Pliocene sediments from Siena (Italy) and in Pleistocene clays from Lecce (Italy), illustrating some specimens and their morphological variability. Since its description, G. gomitulus has been regarded as a species with intermediate features between G. conglobatus and G. ruber or a synonym of G. conglobatus itself (Fornasini, 1898). During the Messina earthquake of 1908, all the type specimens collected by Seguenza (1880) were destroyed. A neotype of G. gomitulus (Seguenza) was subsequently designated by Mistretta (1962), who considered this taxon to be a junior synonym of Globigerinoides elongatus (d'Orbigny), an evaluation that we agree with. Unfortunately, Mistretta's neotype is missing from its original repository at the University of Palermo and we have to rely solely on his published specimen description and series of simple line drawings. Comparison between the original sketches of Seguenza (1880) and the Mistretta (1962) neotype (Fig. 18) reveals clear differences in test size and appearance of the spiral side (e.g. non-matching sutural patterns and the presence of accessory apertures in the neotype which are absent in the holotype). The specimen of Seguenza (1880) is about 3 times larger than the neotype illustrated by Mistretta (1962). Considering that both specimens come from the same geological unit and area in Riace (southern Italy) and are assumed to be of similar age, they could either represent different ontogenetic stages of a single species (e.g. adult vs. juvenile) or, more plausibly, different taxa. This taxonomic issue requires further study with potential need to review the Globigerinoides gomitulus (Seguenza) concept and adequately locate and study Mistretta's neotype (1962). Nevertheless, the G. rublobatus n. sp. differs from both Seguenza (1880) and Mistretta (1962) type specimens of G. gomitulus in the shape and size of the primary aperture and the presence of two pigmentation variants.
Taxonomic study and morphometric analyses provide evidence for Globigerinoides rublobatus n. sp. to be recognised as a new morphospecies of fossil planktonic foraminifera. We find that Globigerinoides rublobatus n. sp. occurs as both pink- and white-coloured forms, but we consider both to be variants of a single morphospecies. We confirm the presence of both chromotypes in Pleistocene sediments from the eastern Indian Ocean (IODP Sites U1482 and U1483) and western Pacific Ocean (IODP Site U1486). Our image analysis, coupled with morphometry of 860 specimens, enables documentation of the morphological variability in Globigerinoides rublobatus n. sp. and determining its relationship with co-occurring Globigerinoides conglobatus and Globigerinoides ruber. We show that Globigerinoides rublobatus n. sp. combines features typical of Globigerinoides conglobatus and Globigerinoides ruber but is distinct from both, justifying the erection of a new species. Further analyses of deep-sea cores from other areas are required to determine the true extent of its geographic distribution and to effectively assess its biostratigraphic potential.
Imaged specimens are deposited at the Natural History Museum, London, UK (PF 75381–75405). The dataset Latas et al. (2022, https://doi.org/10.5285/adb5b0be-a357-4416-846f-777300d78240) produced during this study is available via publicly accessible virtual storage of the National Geoscience Data Centre (NGDC) of the British Geological Survey (BGS). Newly erected species Globigerinoides rublobatus n. sp is registered with ZooBank under the following Life Sciences Identifier (urn:lsid:zoobank.org:act:138D78D5-E2D1-4BE6-83A0-B83D03A1182F).
PNP and CRP discovered and first noted Globigerinoides sp. cf. conglobatus (pink) during the IODP Expedition 363. BSW granted research equipment and access to laboratory facilities for conducting this study. ML carried out sample preparation, laboratory analyses, data collection and interpretation, which took place under the supervision of BSW and PNP. ML, aided by AF, conducted the taxonomic description of the new morphospecies, the study of which was built upon CRP's initial research. AF aided ML in an extensive search of the taxonomic literature, including Italian manuscripts and their translation. The final paper was prepared by ML with contributions from all co-authors.
The contact author has declared that none of the authors has any competing interests.
Publisher's note: Copernicus Publications remains neutral with regard to jurisdictional claims in published maps and institutional affiliations.
We thank Tracy Aze and Silvia Spezzaferri for their insightful reviews. The authors are also grateful to Franco Mistretta for his assistance in recovering historical data and information about the type specimens he illustrated in his work.
This research was supported by the Natural Environment Research Council (NERC) studentship NE/L002485/1 to Marcin Latas. Bridget S. Wade and Christopher R. Poole were supported by NERC grant NE/P016642/1. Paul N. Pearson was supported by NERC grant NE/P016375/1. Alessio Fabbrini was supported by NERC grant NE/P019013/1 to Bridget S. Wade. This study used samples from the International Ocean Discovery Program (IODP) Expedition 363.
This paper was edited by Sev Kender and reviewed by Tracy Aze and Silvia Spezzaferri.
Aurahs, R., Grimm, G. W., Hemleben, V., Hemleben, C., and Kucera, M.: Geographical distribution of cryptic genetic types in the planktonic foraminifer Globigerinoides ruber, Mol. Ecol., 18, 1692–1706, 2009.
Aurahs, R., Treis, Y., Darling, K., and Kucera, M.: A revised taxonomic and phylogenetic concept for the planktonic foraminifer species Globigerinoides ruber based on molecular and morphometric evidence, Mar. Micropaleontol., 79, 1–14, 2011.
Bé, A. W. H. and Hamlin, W. H: Ecology of recent planktonic foraminifera: Part 3 Distribution in the North Atlantic during the Summer of 1962, Micropaleontology, 13, 87–106, https://doi.org/10.2307/1484808, 1967.
Beiersdorf, H., Bickert, T., Cepek, P., Fenner, J., Petersen, N., Schönfeld, J., Weiss, W., and Won, M.-Z.: High-resolution stratigraphy and the response of biota to Late Cenozoic environmental changes in the central equatorial Pacific Ocean (Manihiki Plateau), Mar. Geol., 125, 29–59, https://doi.org/10.1016/0025-3227(95)00021-P, 1995.
Berggren, W. A., Kent, D. V., Swisher III, C. C., and Aubry, M.-P.: A revised Cenozoic geochronology and chronostratigraphy, in: Geochronology, Time Scales and Global Stratigraphic Correlation, edited by: Berggren, W. A., Kent, D. V., Aubry, M.-P., and Hardenbol, J., SEPM (Society for Sedimentary Geology) Special Publication., 54, 129–212, https://doi.org/10.2110/pec.95.04.0129, 1995.
Bhattacharjee, D., Sharma, C., and Bhadury, P.: Chromotypes of Globigerinoides ruber in surface sediments from the north-western coast of the Bay of Bengal, Mar. Biodivers. Rec., 6, 1–5, https://doi.org/10.1017/S1755267213001097, 2013.
Brady, H. B.: Memoirs: Notes on some of the Reticularian Rhizopoda of the “Challenger” Expedition II – Additions to the knowledge of Porcellanous and Hyaline types, Q. J. Microsc. Sci., 19, 261–299, 1879.
Brombacher, A., Wilson, P. A., and Ezard, T. H. G.: Calibration of the repeatability of foraminiferal test size and shape measures with recommendations for future use, Mar. Micropaleontol., 133, 21–27, https://doi.org/10.1016/j.marmicro.2017.05.003, 2017.
Brombacher, A., Elder, L. E., Hull, P. M., Wilson, P. A., and Ezard, T. H. G.: Calibration of test diameter and rea as proxies for body size in the planktonic foraminifer Globoconella puncticulata, J. Foramin. Res., 48, 241–245, https://doi.org/10.2113/gsjfr.48.3.241, 2018.
Brummer, G.-J. A. and Kucera, M.: Taxonomic review of living planktonic foraminifera, J. Micropalaeontol., 41, 29–74, https://doi.org/10.5194/jm-41-29-2022, 2022.
Carpenter, W. B., Parker, W. K., and Jones, T. R.: Introduction to the study of the Foraminifera, Published for the Ray society by R. Hardwicke, London, 1–319, https://doi.org/10.5962/bhl.title.9133, 1862.
Cushman, J. A.: An outline of a re-classification of the Foraminifera, Cont. Cushman Lab. Foram Res., 3, 1–105, 1927.
Darling, K. F. and Wade, C. M.: The genetic diversity of planktic foraminifera and the global distribution of ribosomal RNA genotypes, Mar. Micropaleontol., 67, 216–238, 2008.
Darling, K. F., Wade, C. M., Kroon, D., and Brown, A. J. L.: Planktic foraminiferal molecular evolution and their polyphyletic origins from benthic taxa, Mar. Micropaleontol., 30, 251–266, 1997.
Darling, K. F., Wade, C. M., Kroon, D., Brown, A. J. L., and Bijma, J.: The diversity and distribution of modern planktonic foraminiferal small subunit ribosomal RNA genotypes and their potential as tracers of present and past ocean circulations, Paleoceanography, 14, 3–12, 1999.
Doka, G.: Excel 3-D scatter plot, Doka LCA, https://www.doka.ch/Excel3Dscatterplot.htm (last access: 3 August 2022), 2013.
d'Orbigny, A.: Tableau méthodique de la classe des Céphalopodes, Ann. Sci. Nat., 7, 245–314, 1826.
d'Orbigny, A.: Foraminifères, in: Histoire physique, politique et naturelle de l'ile de Cuba, edited by: de la Sagra, R. M., Arthus Bertrand, Paris, France, 1–224, 1839.
Fornasini, C.: Le Globigerine Fossili d'Italia, Studio Monografico, 4, 203–216, 1898.
Gast, R. J. and Caron, D. A.: Molecular phylogeny of symbiotic dinoflagellates from planktonic foraminifera and radiolaria, Molec. Biol. Evol., 13, 1192–1197, https://doi.org/10.1093/oxfordjournals.molbev.a025684, 1996.
Hemleben, C., Spindler, M., and Anderson, O. (Eds.): Modern Planktonic Foraminifera, Springer-Verlag, New York, United States, 363 pp., https://doi.org/10.1007/978-1-4612-3544-6, 1989.
Hemleben, C., Olsson, R. K., Premec Fucek, V., and Hernitz Kucenjak, M.: Wall textures of Oligocene normal perforate planktonic foraminifera, in: Atlas of Oligocene Planktonic Foraminifera, edited by: Wade, B. S., Olsson, R. K., Pearson, P. N., Huber, B. T., and Berggren, W. A., Cushman Foundation for Foraminiferal Research, Special Publication 46, 55–78, 2018.
Hofker, J.: Foraminifera Dentata, Foramonifera of Santa Cruz and Thatch-Island, Virginia Archipelago, West-Indies, Copenhagen, Spolia Zoologica Musei Haunensis, 15, 237 pp., 1956.
Huber, B. T., Petrizzo, M. R., Young, J. R., Falzoni, F., Gilardoni, S. E., Bown, P. R., and Wade, B. S.: Pforams@microtax: A new online taxonomic database for planktonic foraminifera, Micropaleontology, 62, 429–438, 2016.
Kaneps, A. G.: Cenozoic planktonic foraminifera from the eastern equatorial Pacific Ocean, in: Deep Sea Drilling Project Reports and Publications, DSDP 16, 713–745, https://doi.org/10.2973/dsdp.proc.16.127.1973, 1973.
Kaneps, A. G.: Cenozoic planktonic foraminifera from Antarctic deep-sea sediments, Leg 28, DSDP, in: Deep Sea Drilling Project Reports and Publications, DSDP, 28, 573–583, https://doi.org/10.2973/dsdp.proc.28.113.1975, 1975.
Keller, G.: Depth stratification of planktonic foraminifers in the Miocene Ocean, in: The Miocene Ocean: Paleoceanography and Biogeography, edited by: Kennett, J. P., GSA Memoir 163, The Geological Society of America, Boulder, Colorado, U.S., 177–195, https://geoweb.princeton.edu/archival/keller/Keller-1985-GSA.pdf (last access: 28 February 2023), 1985.
Kennett, J. P. and Srinivasan, M. S. (Eds.): Neogene Planktonic Foraminifera, Hutchinson Ross Publishing Co, Stroudsburg, Pennsylvania, 265 pp., 1983.
King, D. J., Wade, B. S., Liska, R. D., and Miller, C. G.: A review of the importance of the Caribbean region in Oligo-Miocene low latitude planktonic foraminiferal biostratigraphy and the implications for modern biogeochronological schemes, Earth-Sci. Rev., 202, 1–27, https://doi.org/10.1016/j.earscirev.2019.102968, 2020.
Knight, R. and Mantoura, R. F. C.: Chlorophyll and carotinoid pigments in Foraminifera and their symbiotic algae: analysis by high performance liquid chromatography, Mar. Ecol. Prog. Ser., 23, 241–249, https://doi.org/10.3354/MEPS023241, 1985.
Latas, M., Wade, B. S., and Pearson, P. N.: Morphometric data for a new species of fossil planktonic foraminifera, Globigerinoides rublobatus n. sp. from IODP Site U1483A, NERC EDS National Geoscience Data Centre [data set], https://doi.org/10.5285/adb5b0be-a357-4416-846f-777300d78240, 2022.
Mistretta, F.: Foraminiferi planctonici del Pliocene inferiore di Altavilla Milicia (Palermo, Sicilia), Riv. Ital. Pal. Strat., 68, 97–114, 1962.
Morard, R., Füllberg, A., Brummer, G-J. A., Greco, M., Jonker, L., Wizemann, A., Weiner, A. K. M., Darling, K., Sicccha, M., Ladevin, R., Kitazato, H., de Gardiel-Thoron, T., de Vargas, C., and Kucera, M.: Genetic and morphological divergence in the warm-water planktonic foraminifera genus Globigerinoides, PLoS ONE, 14, 1–30, https://doi.org/10.1371/journal.pone.0225246, 2019.
Pearson, P. N., Olsson, R. K., Hemleben, C., Huber, B. T., and Berggren, W. A., Atlas of Eocene Planktonic Foraminifera, Cushman Foundation for Foraminiferal Research, Special Publication 41, Allen Press, Lawrence, Kansas, 514 pp., 2006a.
Pearson, P. N., Olsson, R. K., Huber, B. T., Hemleben, C., Berggren, W. A. and Coxall, H. K.: Overview of Eocene planktonic foraminiferal taxonomy, paleoecology, phylogeny, and biostratigraphy, in: Atlas of Eocene Planktonic Foraminifera, edited by: Pearson, P. N., Olsson, R. K., Hemleben, C., Huber, B. T., and Berggren, W. A., Cushman Foundation for Foraminiferal Research, Special Publication 41, Allen Press, Lawrence, Kansas, 11–28, 2006b.
Pearson, P. N. and Penny, L.: Coiling directions in the planktonic foraminifer Pulleniatina: A complex eco-evolutionary dynamic spanning millions of years. PLoS ONE, 16, 1–29, https://doi.org/10.1371/journal.pone.0249113, 2021.
Poole, C. R. and Wade, B. S.: Systematic taxonomy of the Trilobatus sacculifer plexus and descendant Globigerinoidesella fistulosa (planktonic foraminifera), J. Syst. Palaeontol., 17, 1989–2030, https://doi.org/10.1080/14772019.2019.1578831, 2019.
Raffi, I., Wade, B. S., and Pälike, H.: The Neogene Period, in: Geologic Time Scale 2020, edited by: Gradstein, F. M., Ogg, J. G., Schmitz, M. D., Ogg, G. M., Vol. 2, Elsevier, 1141–1215, https://doi.org/10.1016/B978-0-12-824360-2.00029-2, 2020.
Riech, V.: Calcareous ooze, volcanic ash, and metalliferous sediments in the Quaternary of the Lau and North Fiji Basins, Goeol. Jahrb., Reihe D., 92, 109–162, 1990.
Rosenthal, Y., Holbourn, A. E., Kulhanek, D. K., Aiello, I. W., Babila, T. L., Bayon, G., Beaufort, L., Bova, S. C., Chun, J. -H., Dang, H., Drury, A. J., Dunkley Jones, T., Eichler, P. P. B., Fernando, A. G. S., Gibson, K., Hatfield, R. G., Johnson, D. L., Kumagai, Y., Li, T., Linsley, B. K., Meinicke, N., Mountain, G. S., Opdyke, B. N., Pearson, P. N., Poole, C. R., Ravelo, A. C., Sagawa, T., Schmitt, A., Wurtzel, J. B., Xu, J., Yamamoto, M., and Zhang, Y. G.: Expedition 363 summary, in: Proceedings of the International Ocean Discovery Program, edited by: Rosenthal, Y., Holbourn, A. E., Kulhanek, D. K., and the Expedition 363 Scientists, Western Pacific Warm Pool, College Station, TX (International Ocean Discovery Program), 363, 1–53, https://doi.org/10.14379/iodp.proc.363.101.2018, 2018a.
Rosenthal, Y., Holbourn, A. E., Kulhanek, D. K., Aiello, I. W., Babila, T. L., Bayon, G., Beaufort, L., Bova, S. C., Chun, J. -H., Dang, H., Drury, A. J., Dunkley Jones, T., Eichler, P. P. B., Fernando, A. G. S., Gibson, K., Hatfield, R. G., Johnson, D. L., Kumagai, Y., Li, T., Linsley, B. K., Meinicke, N., Mountain, G. S., Opdyke, B. N., Pearson, P. N., Poole, C. R., Ravelo, A. C., Sagawa, T., Schmitt, A., Wurtzel, J. B., Xu, J., Yamamoto, M., and Zhang, Y. G.: Site U1482, in: Proceedings of the International Ocean Discovery Program, edited by: Rosenthal, Y., Holbourn, A. E., Kulhanek, D. K., and the Expedition 363 Scientists, Western Pacific Warm Pool, College Station, TX (International Ocean Discovery Program), 363, 1–62, https://doi.org/10.14379/iodp.proc.363.103.2018, 2018b.
Rosenthal, Y., Holbourn, A. E., Kulhanek, D. K., Aiello, I. W., Babila, T. L., Bayon, G., Beaufort, L., Bova, S. C., Chun, J. -H., Dang, H., Drury, A. J., Dunkley Jones, T., Eichler, P. P. B., Fernando, A. G. S., Gibson, K., Hatfield, R. G., Johnson, D. L., Kumagai, Y., Li, T., Linsley, B. K., Meinicke, N., Mountain, G. S., Opdyke, B. N., Pearson, P. N., Poole, C. R., Ravelo, A. C., Sagawa, T., Schmitt, A., Wurtzel, J. B., Xu, J., Yamamoto, M., and Zhang, Y. G.: Site U1483, in: Proceedings of the International Ocean Discovery Program, edited by: Rosenthal, Y., Holbourn, A. E., Kulhanek, D. K., and the Expedition 363 Scientists, Western Pacific Warm Pool, College Station, TX (International Ocean Discovery Program), 363, 1–43, https://doi.org/10.14379/iodp.proc.363.104.2018, 2018c.
Saito, T., Thompson, P. R., and Breger, D. (Eds.): Systematic Index of Recent and Pleistocene Planktonic Foraminifera, University of Tokyo Press, Tokyo, ISBN 0-86008-280-6, 1981.
Schiebel, R. and Hemleben, Ch.: Modern planktic foraminifera, Paläontol. Z., 79, 135–148, https://doi.org/10.1007/BF03021758, 2005.
Schiebel, R. and Hemleben, C.: Classification and Taxonomy of Extant Planktonic Foraminifers, in: Planktic Foraminifers in the Modern Ocean, edited by: Schiebel, R. and Hemleben, C., Springer, Berlin, Heidelberg, Germany, 11–110, https://doi.org/10.1007/978-3-662-50297-6, 2017.
Seguenza, G.: Le foramazioni terziarie nella provincia di Reggio (Calabria), Memorie della Classe di Scienze Fisiche Matematiche e Naturali della Regia Accademia del Lincei., 3, 445 pp, http://www.biodiversitylibrary.org/item/89318 (last access: 11 November 2022), 1880.
Shaked, Y. and de Vargas, C.: Pelagic photosymbiosis: rDNA assessment of diversity and evolution of dinoflagellate symbionts and planktonic foraminiferal hosts, Mar. Ecol. Prog. Ser., 325, 59–71, https://doi.org/10.3354/meps325059, 2006.
Silvestri, A.: Foraminiferi Pliocenici della provincia di Siena, Memoria, II, 154–381, 1898.
Spezzaferri, S., Kucera M, Pearson, P. N., Wade, B. S., Rappo, S., Poole, C. R., Morard, R., and Stalder, C.: Fossil and Genetic Evidence for the Polyphyletic Nature of the Planktonic Foraminifera “Globigerinoides”, and Description of the New Genus Trilobatus, PLoS ONE, 10, 1–20, https://doi.org/10.1371/journal.pone.0128108, 2015.
Spezzaferri, S., Olsson, R. K., and Hemleben, C.: Taxonomy, biostratigraphy, and phylogeny of Oligocene to Lower Miocene Globigerinoides and Trilobatus, in: Atlas of Oligocene Planktonic Foraminifera, edited by: Wade, B. S., Olsson, R. K., Pearson, P. N., Huber, B. T., and Berggren, W. A., Cushman Foundation for Foraminiferal Research, Special Publication, 46, 269–306, 2018.
Stainbank, S., Spezzaferri, S., Kroon, D., de Leau, E. S., and Rüggeberg, A.: The Planktonic foraminifera Globigerinoides eoconglobatus n. sp. in a glacial–interglacial context: IODP 359 Sites U1467 and U1468, Swiss J. Geosci., 111, 511–522, https://doi.org/10.1007/s00015-018-0304-9, 2018.
Takagi, H., Kimoto, K., Fujiki, T., Saito, H., Schmidt, C., Kucera, M., and Moriya, K.: Characterizing photosymbiosis in modern planktonic foraminifera, Biogeosciences, 16, 3377–3396, https://doi.org/10.5194/bg-16-3377-2019, 2019.
Thompson, P. R., Bé, A. W. H., Duplessy, J. C., and Shackleton, N. J.: Disappearance of pink pigmented Globigerinoides ruber at 120 000 yr BP in the Indian and Pacific Oceans, Nature, 280, 554–558, https://doi.org/10.1038/280554a0, 1979.
Von Daniels, C. H.: Foraminifera and oxygen isotope stratigraphy in a core from the Lau Basin, southwest Pacific Ocean, Goeol. Jahrb., Reihe D., 92, 209–229, 1990.
Wade, B. S., Pearson, P. N., Berggren, W. A., and Pälike, H.: Review and revision of Cenozoic tropical planktonic foraminiferal biostratigraphy and calibration to the geomagnetic polarity and astronomical time scale, Earth-Sci. Rev., 104, 111–142, https://doi.org/10.1016/j.earscirev.2010.09.003, 2011.
Wade, B. S., Olsson, R. K., Pearson, P. N., Huber, B. T., and Berggren, W. A. (Eds.): Atlas of Oligocene Planktonic Foraminifera, Cushman Foundation for Foraminiferal Research, Special Publication 46, 524 pp., 2018a.
Wade, B. S., Pearson, P. N., Olsson, R. K., Premoli Silva, I., Berggren, W. A., Spezzaferri, S., Huber, B. T., Coxall, H. K., Premec-Fucek, V., Hernitz Kucenjak, M., Hemleben, C., Leckie, M. R., and Smart, C. W.: Taxonomy, biostratigraphy, phylogeny, and diversity of Oligocene and early Miocene planktonic foraminifera, in: Atlas of Oligocene Planktonic Foraminifera, edited by: Wade, B. S., Olsson, R. K., Pearson, P. N., Huber, B. T., and Berggren, W. A., Cushman Foundation for Foraminiferal Research, Special Publication, 46, 11–28, 2018b.