the Creative Commons Attribution 4.0 License.
the Creative Commons Attribution 4.0 License.
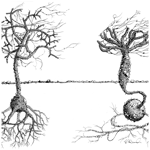
Distribution of two notodendrodid foraminiferal congeners in McMurdo Sound, Antarctica: an example of extreme regional endemism?
Andrea Habura
Stephen P. Alexander
Steven D. Hanes
Andrew J. Gooday
Jan Pawlowski
Samuel S. Bowser
We used morphological and molecular surveys to determine the presence or absence of Notodendrodes antarctikos and its congener, Notodendrodes hyalinosphaira, at diverse sites within McMurdo Sound, Antarctica. Morphological surveys were performed using shipboard box-core sampling, as well as handheld coring and visual inspection by divers in shallow (< 23 m) waters. Concurrent molecular analyses were performed using species- and genus-specific PCR primers on environmental DNA extracts. Both survey methods show that N. hyalinosphaira is widely distributed in the region but that N. antarctikos was not detected outside its originally reported range. The survey methods show complementary strengths and weaknesses, with morphological detection being more sensitive in areas where large and distinctive adult forms are present and with molecular detection being more effective for identification of presumed juvenile or propagule stages. Our results suggest that N. antarctikos is a highly endemic protist and may have one of the most restricted ranges ever reported for an Antarctic organism.
- Article
(2586 KB) - Full-text XML
- BibTeX
- EndNote
Larger (> 1 mm) agglutinated foraminifera are diverse and ecologically important protists in modern marine settings, notably in polar and deep-sea habitats (reviewed in Murray, 2006). Many species belong to the single-chambered Monothalamea (“monothalamids”), a polyphyletic group that includes genera such as Astrammina spp., Astrorhiza spp., and Rhabdammina spp. Some deep-sea members of this group have morphologically complex tests, among them the macrofaunal Komokioidea and the megafaunal Xenophyophoroidea. In addition to being notable and often visually prominent members of modern benthic communities, monothalamids have a long geological history and include the earliest (lower Cambrian) foraminifera (e.g., Platysolenites, McIlroy et al., 1996) to appear in the fossil record.
Among the morphologically most striking large monothalamids found in coastal Antarctic waters are two arborescent epifaunal species belonging to the genus Notodendrodes, namely N. antarctikos and N. hyalinosphaira (Fig. 1). These species are superficially similar to tree-like foraminifera known from many other parts of the world, for example Arborammina hilaryi, Luffammina atlantica, and Pelosina arborescens (Cedhagen, 1993; Shires et al., 1994; Kamenskaya et al., 2002; see also Fig. 5 of DeLaca et al., 1980). Notodendrodids are distinguished from these and other monothalamids, however, by the presence of an allogromiid-like cell body encased by an agglutinated test (Bowser et al., 1995a).
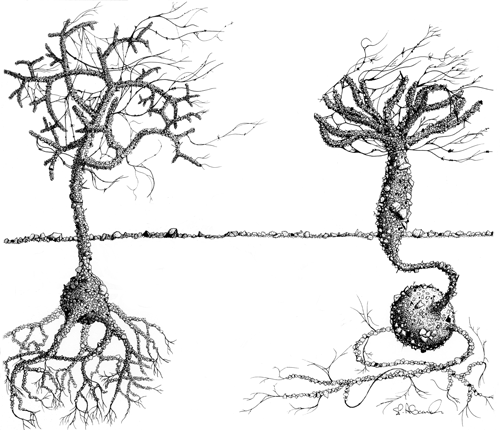
Figure 1Notodendrodes species. Notodendrodes antarctikos (left) has a much more gracile morphology than N. hyalinosphaira (right). “Tree” morphs of both species may project more than 2 cm above the seafloor and are readily observed by divers. Drawing by Stephen P. Alexander.
In this paper we describe the regional distribution of N. antarctikos and N. hyalinosphaira in McMurdo Sound, Antarctica. As originally reported (DeLaca et al., 1980, 2002), notodendrodids occurred exclusively in the New Harbor region on the western side of McMurdo Sound, Antarctica, with N. antarctikos only found within Explorers Cove. These restricted occurrences contrast with the more broad circumpolar distributions of many Antarctic foraminifera, including some that are reported from Explorers Cove – e.g., Astrononion antarcticus, Cibicides antarcticus, Globocassidulina biora, Pseudobolivina antarctica, Textularia earlandi, T. wiesneri (summarized in Gooday et al., 2014). Broad distributions for some Antarctic benthic species are also suggested by molecular surveys (Majewski et al., 2015).
Notodendrodids are well suited for addressing issues in foraminiferal biogeography. The macroscopic, arborescent test structure renders them amenable to visual assessments, including direct observation by scuba divers (DeLaca et al., 1980, 2002), and their distinctive morphology correlates well with molecular identification based on ribosomal small subunit sequence data (Pawlowski et al., 2002a, b). The molecular screening approaches used here for detecting the presence of foraminiferal species in environmental DNA are now well established (e.g., Habura et al., 2004a; Pawlowski et al., 2011, 2012). In addition, secondary structural predictions (Habura et al., 2004b) have helped to inform the development of the group-specific molecular probes that were used in the present study.
Our new results expand the known range of Notodendrodes hyalinosphaira and confirm the restricted occurrence of N. antarctikos to Explorers Cove and nearby Cape Bernacchi. To date, notodendrodids have not been found elsewhere in Antarctic waters, or indeed anywhere globally, despite their visual prominence and the extensive cataloging of benthic foraminifera by protistologists and micropaleontologists for almost 2 centuries, supplemented in more recent years by molecular surveys. We also review and revise observations on the Explorers Cove benthos as it pertains to the distribution of these distinctive Antarctic “tree forams”. Our aim is to provide a roadmap for future investigations of the modern distribution of notodendrodid foraminifera, with particular emphasis on their occurrence in deeper areas of McMurdo Sound that were presumably ice-free during the Last Glacial Maximum.
As opportunities arose during 2 decades of fieldwork (1998–2016), various nearshore sites along western and eastern McMurdo Sound, as well as several deeper sites in the sound, were sampled by us and others to examine the range of notodendrodid foraminifera (Fig. 2). Shallow-water sampling involved scuba diving as detailed in Pollock and Bowser (1995) or the use of a small box corer deployed from the ice surface. Deep-water sampling was conducted aboard the US Coast Guard icebreaker Polar Star using a Soutar box corer. Sampling site locations are given in Table 1.
Table 1Summary of sampling sites (in some cases named for a nearby geographic feature), sampling methods, and results. V: visual detection by scuba divers; HC: handheld cores taken by divers; BC: box-core surface sediment; SG: surface grab sampler; n.d.: not determined.
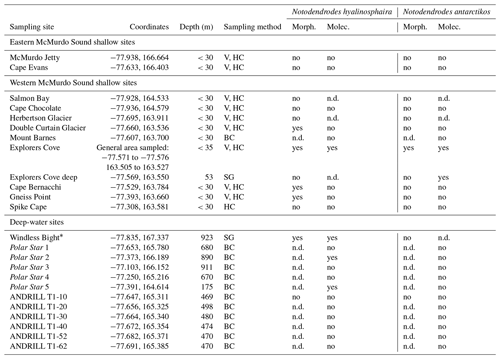
* Windless Bight (WB) was analyzed in Pawlowski et al. (2005).
Surface (top ∼ 1 cm) sediment was sieved through a 1 mm mesh, and residues were picked using a stereomicroscope. For molecular analysis, either the unsieved sediment was snap-frozen in liquid nitrogen and returned to the lab frozen, or sieved residues were preserved in 95 % ethanol for further processing.
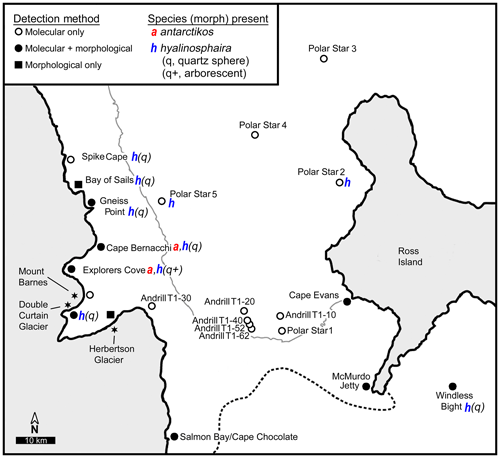
Figure 2Sampling sites. Map showing sampling locations within McMurdo Sound, Antarctica. Typical boundaries of seasonal ice (thin gray line) and the Ross Ice Shelf (dotted line) are indicated.
2.1 DNA extraction from sediment and individual organisms
Total DNA was extracted from 10 mL sediment samples as previously described (Habura et al., 2004a). DNA equivalent to the extract from 1.5 mL of sediment was further purified for species-specific panels with the DNeasy kit (QIAGEN Inc. Valencia, CA). DNA from 30 isolated cells of Notodendrodes hyalinosphaira was purified by a small-scale version of the sediment preparation.
2.2 PCR and sequencing
Sediment extracts were diluted 1:50 to serve as PCR templates. Sediment samples used for species-specific panels were adjusted so that each reaction contained template representing the total yield from 30 mm3 of sediment. Amplification of total foraminiferal SSU rDNA was performed using the primers s14F3A, s14F1, s20R, and B as described previously (Habura et al., 2004a). In addition, we designed primer sets specific for the genus Notodendrodes and for the individual species N. hyalinosphaira and N. antarctikos using published sequences (AJ311213 and AJ311214) as well as several unpublished sequences derived from isolated individuals. These primers exploit a variable region in Domain III of the foraminiferal SSU, which has been shown to be useful for distinguishing between closely related foraminiferal species (Bowser et al., 2006). Primer sequences for the genus-specific set are Notfor:TATGGCWCGYAYGTGTTTTATRCTAATAC and Notrev:TGCATCAGCCMGCTAACACYAACAGG. Sets for the individual species are Notohyal1:GGTGTTTCGTTTTSATATGGTAT and Notohyalrev:GGCAAAAACCGAAAGCATAACACA as well as Notoant1:GGTGTTTCGTTTTGATGCAGTAC and Notoantrev:GGCAAAACTGAAAGCGTAACATG.
PCR reactions were performed using ExTaq proofreading Taq polymerase premix (TaKaRa Bio Inc., Madison, WI) in a Techne Genius thermocycler (Techne Inc., Princeton, NJ). All samples were amplified with primers s14F3a and sB with the following parameters: 1 cycle (94 °C 2 min, 48 °C 1 min, 70 °C 2 min); 35 cycles (94 °C 30 s, 48 °C 1 min, 70 °C 2 min); 1 cycle (94 °C 30 s, 48 °C 1 min, 70 °C 15 min). For total foraminiferal DNA detection, 0.1 µL of the reaction mixture was used as a template for amplification with primers s14F1 and s20r (20 cycles, annealing temperature set at 51 °C). For detection of notodendrodid forams, the primer pair Notfor/Notrev was used, with annealing temperature set at 55 °C. For specific detection of notodendrodid forams, the primer pairs Notfor/Notrev, Notohyal1/Notohyalrev, and Notoant1/Notoantrev were used, with annealing temperature set at 55 °C.
Products were cloned into the sequencing vector pGEM®-T Easy (Promega Corp., Madison, WI) and replicated in E. coli strain JM109. Individual clones were purified using the SpinPrep Mini Kit (QIAGEN) and sequenced in both directions using primers M13 and M13 reverse with a PE-Biosystems ABI PRISM 377XL automated DNA sequencer. Sequences were compared with the BLAST utility (NCBI) to known sequences in GenBank and manually aligned with an existing 200-sequence alignment containing known sequences from notodendrodids and other foraminifera.
3.1 Visual identification of notodendrodid species
Because the arborescent structures constructed by notodendrodids protrude a centimeter or more above the surface of the seafloor, both species are readily visible to scuba divers. The “trees” can be distinguished from each other macroscopically (Fig. 1), as the arborescent form of N. antarctikos' is much more gracile than that of N. hyalinosphaira. In this study, surveys of wide areas of the seafloor were accomplished by divers visually scanning the surface. This visual assessment was confirmed by microscopic analysis of wet-picked specimens recovered from the top 2 cm of sediment cores. Neither species was found at depths where anchor ice was present (e.g., 0–10 m at New Harbor; 0–22 m at the Herbertson Glacier dive site); N. antarctikos was encountered deeper – from approximately 20 m to our maximum diving depth (35 m).
In deep-water (> 50 m) samples, the entire box-core surface was visually assessed and then sieved, and the preserved > 1 mm residues were examined using a stereomicroscope. Our broad survey in McMurdo Sound revealed a very restricted distribution of N. antarctikos, whereas its congener N. hyalinosphaira was more widely distributed (Table 1). However, at certain sites there was no morphological evidence for either species.
3.2 Identification of Notodendrodes DNA in sediment samples
Total environmental DNA was extracted from sediment samples taken from several localities within McMurdo Sound (see Table 1). All samples gave a positive signal when probed with PCR primers specific for all foraminifera. A suite of primers specific for foraminiferal groups (Habura, unpublished) indicates that several different species are readily detected in these samples (Fig. 3). This result is consistent with previous environmental DNA analyses (e.g., Habura et al., 2004a), which show that Antarctic sediments are rich in foraminiferal DNA.
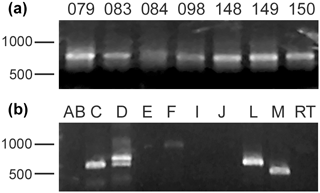
Figure 3Foraminiferal DNA in Antarctic samples. (a) Foraminiferal DNA is present in Antarctic samples. Shown are SSU rDNA PCR amplimers using foraminifera-specific primers from several sites in the Ross Sea, including Explorers Cove. (b) Molecular foraminiferal assemblages at sites contain multiple species. Amplification of an extract from the ANDRILL T1-20 site with primers specific for individual foraminiferal groups shows that members of several clades (allogromiids and multilocular foraminifera) are present. “Clade F” allogromiids, which include the notodendrodids as well as other Antarctic species such as Rhabdammina cf. cornuta and Hemisphaerammina bradyi, are detectable in this sample.
To identify notodendrodid DNA in these environmental extracts, we designed three pairs of primers specific for the SSU rDNA of notodendrodids. One pair is targeted against both members of the genus (Notfor and Notrev), and the other two are specific for N. hyalinosphaira alone (Notohyal1/Notohyalrev) and N. antarctikos alone (Notoant1/Notoantrev). All three sets efficiently amplify SSU rDNA from a purified cell template and do not cross-react with DNA from other foraminifera (Fig. 4a).
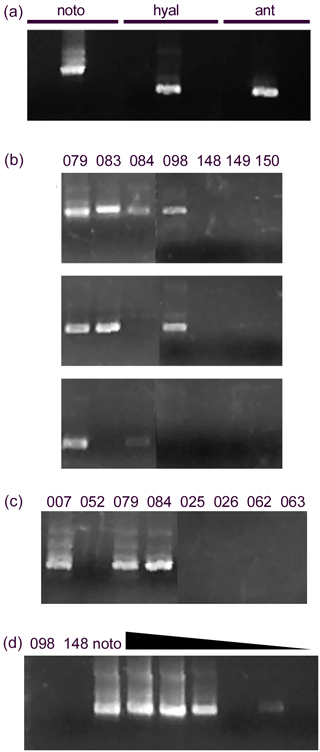
Figure 4Detection of notodendrodids with specific primers. (a) Primers designed to be specific for notodendrodid SSU rDNA yield product from a mixed sample of isolated N. hyalinosphiara and N. antarctikos DNA (visible as a prominent band within the center lanes of each set) but not from DNA from other allogromiid foraminifera. Sequencing of products shows that Notfor/Notrev amplimers (left lanes) contain products from both species, and the Notohyal and Notoant pairs contain products from only the species in question. (b) Notodendrodid DNA is detectable in some samples, especially those from Explorers Cove (079, 083, 084, 098), but other samples do not respond to the primers. Top: Notfor/notrev. Center: Notohyal1/Notohyalrev. Bottom: Notoant1/Notoantrev. (c) “Boosting” of signal. The use of nested PCR with multiple notodendrodid-specific primer sets results in improved detection at some sites (e.g., 084), but other samples still do not contain amplifiable DNA. Primer pairs: Notfor/Notrev followed by Notohyal1/Notohyalrev. (d) Lack of detection is not due to PCR inhibition. Left lanes: sediment samples (098, 148) and cell extracts (noto) are amplified with the Notohyal1/Notohyalrev pair. Right lanes: dilution series of noto extract mixed with sediment sample 148. All reactions contain 1 µL of sediment extract; noto extract is added at different concentrations (1 to 0.0001 µL). Amplification of noto extract is not impaired by the presence of 148 extract.
The notodendrodid-specific primer sets identify members of this genus in Explorers Cove and at two other sites within the sound (Fig. 4b). Sequence analysis of the PCR products from the Notfor/Notrev pair showed that amplimers matching the sequences of both N. hyalinosphaira and N. antarctikos were derived from samples taken within the cove. Another sample, taken from a deeper, slightly downslope site (Explorers Cove deep, 53 m, Table 1) produced amplimers identical in sequence to that of N. antarctikos. Finally, an N. hyalinosphaira signal was detected at two deep-water sites (Polar Star 2, Polar Star 5) near Ross Island. No amplimers corresponding to non-notodendrodid foraminiferal SSU rDNA sequences were identified with any of the primer sets, and no additional member of the genus was identified in screens using the genus-specific primer set. None of the Notodendrodes-specific primer sets amplified product in sediment samples derived from low-latitude sites (e.g., Habura et al., 2008) which are known to contain foraminiferal DNA (data not shown).
Notodendrodes hyalinosphaira was identified morphologically in some areas of the sound (Cape Bernacchi, Gneiss Point, and Windless Bight), where environmental DNA screens failed to detect their presence (this study and Pawlowski et al., 2005). We therefore attempted to improve detection efficiency using nested amplification with different pairs of notodendrodid primers (Fig. 4c). While this method improved detection in some Explorers Cove samples, it did not detect N. hyalinosphaira DNA at sites such as Cape Bernacchi.
PCR reactions can also be inhibited by the presence of trace compounds or by overabundance of template. To determine whether these factors influenced our ability to detect notodendrodid DNA, we “spiked” purified sediment samples with known amounts of N. hyalinosphaira DNA (Fig. 4d). Even at spiking quantities equivalent to the extract from less than a single cell, the primers efficiently detected the notodendrodid DNA in the sample. Therefore, the lack of detection in these templates cannot be due to inhibition of amplification.
4.1 General aspects of foraminiferal biogeography
Most adult foraminifera lie within the size range 100 µm to 1 cm, which spans the transition between predominantly cosmopolitan (< 1 mm) and predominantly endemic (> 10 mm) distributions of protists (Finlay and Fenchel, 2004). However, the reproductive propagules of foraminifera, which are perhaps their main agents of dispersal, are < 32 µm (Alve and Goldstein, 2003, 2010) and therefore comparable in size to microbial protists. We would, therefore, expect environmental factors such as currents, food availability, and sediment characteristics to strongly influence their biogeographic ranges – an expectation largely supported by our findings on notodendrodids and the numerous surveys over the past century (reviewed in Murray, 2006).
Despite their potential to disperse broadly, some foraminiferal species living in coastal and continental shelf settings appear to have restricted distributions (Gooday and Jorissen, 2012; Hayward et al., 2021; Hayward and Holzmann, 2023). For example, large agglutinated foraminifera of the genera Jullienella and Schizammina (family Schizamminidae) are confined to particular regions of the shelf in different parts of the world (Nørvang, 1961; Hayward and Gordon, 1984; Tendal and Cedhagen 2007; Langer et al., 2022). Indeed, more than half (57 %) of the 2329 species recorded by Culver and Buzas (1998) around North and Central America were endemic in the sense of being confined to one of five regions (Caribbean, Gulf of Mexico, Atlantic, Arctic, and Pacific) around this continent. When global distribution records are considered, however, levels of endemism may be much lower; for example, 4 % of the 987 foraminiferal species known from the Gulf of Mexico have not been found elsewhere in the world (Gupta and Smith, 2010). Foraminiferal distributions are much wider in the deep sea and particularly at abyssal depths (Gooday and Jorissen, 2012), a pattern that extends to many other taxa and reflects the generally more uniform environmental conditions and the presence of fewer barriers to dispersal in the abyss (McClain and Hardy, 2010).
4.2 Distribution of notodendrodids
Based on both morphological and molecular detection methods, N. antarctikos is a very narrowly distributed species. Morphological methods detected it only within Explorers Cove, a relatively oligotrophic, silty, and hydrodynamically low-energy embayment of New Harbor on the western Ross Sea, and somewhat northward toward Cape Bernacchi. Its molecular signature was also detected in the single deeper sample in the cove, indicating that its range might extend deeper; additional mapping of its bathymetric range seems warranted.
We also sampled comparable nearshore settings along western McMurdo Sound (Fig. 2 and Table 1) and were unable to identify the presence of N. antarctikos in these locales. In contrast, its congener N. hyalinosphaira was more widely distributed but is still generally confined to southern regions within the Ross Sea. We are not convinced that N. cf. antarctikos of Violanti (1996) from 163–1010 m at Terra Nova Bay belongs to this species; the description and corresponding photograph do not correspond to the known morphology of either member of the genus. Apart from this one dubious record, these distinctive species have not been reported anywhere in the extensive literature on benthic foraminifera from Antarctic continent surveys of the Weddell Sea and the Antarctic peninsula (Cornelius and Gooday, 2004; Gooday et al., 2007). Superficially similar tree-like foraminiferal species, such as Arborammina hilaryi and Pelosina arborescens, are known from many other parts of the world (Cedhagen, 1993; Shires et al., 1994) but are genetically and anatomically distinct from Notodendrodes.
As mentioned above, few of the species of foraminifera that live in coastal waters are truly cosmopolitan (Murray, 2006; Pawlowski and Holzmann, 2008). On the other hand, species with highly restricted distributions appear to be very unusual (Murray, 2013). Some large monothalamids in the family Schizamminidae appear to be confined to the continental shelf in different parts of the world (Nørvang, 1961; Hayward and Gordon, 1984; Tendal and Cedhagen, 2007), but their distributions still span considerably larger areas than Explorers Cove. Based on available evidence, N. antarctikos may be one of the most locality-restricted foraminifera, or even protist, in the world.
4.3 Possible factors restricting the range of Notodendrodes antarctikos
Murray (2006, p. 53) posed the following question: why is a given species absent from one area or environment while it is present in an adjacent area or environment? A comparison of life habits of the two congeners may suggest some possibilities or at least constrain them. A clear-cut explanation for the apparently highly restricted range of N. antarctikos remains, however, a subject for future studies.
4.3.1 Trophic considerations
Food availability and trophic mechanisms are fundamental aspects of species survivorship. Both notodendrodids construct arborescent test appendages that project into the water column. In N. antarctikos, the arborescent structure is obligatory, whereas in N. hyalinosphaira it is expressed in only a few individuals (DeLaca et al., 2002). Notodendrodid pseudopodial networks are deployed from the surfaces of these arborescent structures (Suhr et al., 2008), enabling them to capture suspended food (particulate organic matter, microbes, etc.) through particle interception (Vogel, 1994). They can also capture and subsequently rend (see Bowser, 1985) small metazoans (Suhr et al., 2008). The agglutinated root-like anchoring structures have also been implicated in osmotrophic uptake of nutrients from sediment pore waters (DeLaca et al., 1981; DeLaca, 1982). Both notodendrodids are therefore capable of exploiting a wide range of trophic resources and can potentially switch between feeding strategies on a seasonal basis (Suhr et al., 2008). Moreover, Altenbach et al. (1999) showed that, although many species of foraminifera are most common where organic matter fluxes (food supply) are optimal, they also occur at lower abundances across a much wider range of flux values. It seems unlikely, therefore, that food availability is a major factor limiting the range of N. antarctikos.
4.3.2 Mechanical disturbance
Disturbances caused by hydrodynamic (currents) and biological (bioturbation by megafauna and macrofauna) activity could potentially limit the distribution of N. antarctikos to Explorers Cove and its immediate vicinity. As mentioned above (Sect. 4.3.1), the “tree” in N. antarctikos is an obligatory portion of the agglutinated test and is firmly cemented to the bulbous quartz sphere (DeLaca et al., 1980), whereas in N. hyalinosphaira it is found in relatively few specimens, where it is loosely attached to the bulbous sphere by a thin, loosely agglutinated umbilicus (DeLaca et al., 2002). Compared to elsewhere in the McMurdo Sound, Explorers Cove is distinguished by the absence of strong currents and the low abundance of predatory macrofauna (for descriptions of Explorers Cove see, e.g., Dayton and Oliver, 1990; DeLaca, 1982; Gooday et al., 1996; Miller et al., 2015), so mechanical disturbance is likely to be a minor problem. The movements of the two primary bioturbators (the bivalve Adamussium colbecki and ophiuroid Ophionotus victoriae) disturb the surface sediments and probably play an important role in resuspending surface particulates (e.g., McClintock et al., 2010; Broach et al., 2016), although they seem unlikely to break or damage tests. Outside the cove, however, where there is more current activity and larger deposit feeders like holothurians are more abundant (Dayton and Oliver, 1980), relatively delicate structures such as notodendrodid “trees” may be more exposed to mechanical damage and predation.
Preliminary studies of test reconstruction following microsurgical removal of the agglutinated test (“shucking” – see Bowser et al., 1995b) found that, unlike positive controls (Astrammina rara, Astrammina triangularis), notodendrodids died shortly after the shell was removed from the cell body (unpublished observations). Structurally, the cell bodies of the two Astrammina species are encased by thick, multi-laminated, fibrous thecas that permit shucking without damage (Bowser et al., 1995a, 2002). In contrast, the cell bodies of notodendrodids are covered by a thin, felt-like layer that is very easy to rupture when micromanipulated using polished needles (Bowser et al., 1995b, and unpublished observations). Notodendrodids are therefore more likely to suffer mechanical damage and subsequent mortality than astramminids, particularly since they have a rather large (centimeter-scale) cross-sectional area exposed above the sediment–water interface. We anticipate that N. antarctikos is likely to be prone to damage and/or death because the arborescent extension would probably yield when subjected to mechanical or hydrodynamic forces. The portion of the test that surrounds the cell body (i.e., the basal sphere) of N. antarctikos is firmly cemented to the arborescent structure; indeed, intact specimens can be pulled out of the sediment using tweezers. Outside the low-energy environs of Explorers Cove, higher currents might therefore uproot N. antarctikos and displace it to less favorable habitats. By contrast, the flimsier arborescent structure of N. hyalinosphaira behaves as an “anchored buoy” under stress, as observed directly by divers (SSB, SPA) during in situ manipulations. Breakage of the umbilicus would only sever pseudopodia from the cell body. Based on microsurgical studies in other foraminifera (e.g., Travis et al., 2002), this would be unlikely to result in death. Unlike that of N. antarctikos, the agglutinated sphere in which most of the cell body of N. hyalinosphaira resides would remain in the sediment and be relatively safe from displacement.
4.4 Differences between morphological and molecular detection methods
Why did morphological and molecular detection methods sometimes give different results in this survey? We believe that each of the methods can fail under different circumstances. It has been demonstrated (Habura et al., 2004a, 2008) that foraminiferal DNA from a given species can be detected in samples in which morphologically identifiable specimens of that species are absent. This can be caused by several factors, including the presence of “propagule” stages (Alve and Goldstein 2003, 2009), which are most likely the primary dispersal method for many benthic foraminifera. In cases where propagules or morphologically ambiguous juveniles dominate the assemblage (e.g., “the bewildering assortment of …spherical and domed agglutinated [foraminifera]”, Gooday et al., 1996, p. 134), molecular detection may be more useful, especially in light of newer molecular methodologies, such as single-cell transcriptomics.
Conversely, species present in relatively low abundance or with patchy distributions can easily be missed in molecular surveys, particularly given the small volumes of sediment analyzed for foraminiferal DNA. The species- and genus-specific strategy we employ here should have reduced “swamping” by DNA from more common foraminiferal species. However, it is still possible that, below a certain threshold, DNA from a given species simply cannot be detected by this method. The notodendrodids may be among the “long tail” of protistan taxa according to numeric criteria (for a discussion, see Pedrós-Alió, 2006) but still be morphologically conspicuous, much like whales or lions might be in their environments. In such circumstances, morphological detection of adults may be much more efficient than molecular detection, much like the visual sighting of whales would be more effective than molecular analysis of seawater. In this context, underwater cameras equipped with long-focal-length macro-optics, deployed either on a fixed station (e.g., Hancock et al., 2015) or aboard remotely operated vehicles (e.g., Daly et al., 2013; Burns et al., 2024), would be useful tools for broader surveys of notodendrodid waters beyond non-decompression diving depths, particularly in the 53 to < 175 m range absent from our study.
Many Antarctic foraminiferal morphospecies have a circumpolar distribution. Our evidence suggests that notodendrodid monothalamids appear to be exceptions to this general pattern in being restricted to the McMurdo Sound region of the Ross Sea. Notodendrodes antarctikos, in particular, is currently known only in Explorers Cove and nearby Cape Bernacchi in western McMurdo Sound. The habitat it occupies must be of recent origin since a grounded ice sheet extended nearly to the edge of the continental shelf during the Last Glacial Maximum (Conway et al., 1999; Prothro et al., 2020), leaving little or no shallow-water habitat in the Ross Sea during this period. This raises interesting questions regarding the origin of this population. It seems unlikely that N. antarktikos could have evolved in situ during the last 12 000 years. The only plausible scenarios would seem to be that it migrated from somewhere else, in which case it may not be as narrowly endemic as it currently appears, or an ice-free refugia existed under the ice sheet in McMurdo Sound. In this context, surveys of foraminifera inhabiting specific deeper sites, such as the JOIDES and Pennell troughs, will be instructive (Prothro et al., 2020).
Assuming that N. antarctikos really is restricted to Explorers Cove, then it seems to meet all the criteria for threatened or endangered status. The policy implications of this would be of considerable interest, especially since other examples of protists with severely restricted ranges have also been described (e.g., Foissner, 2006). Buzas and Culver (1998) identify a total of 537 foraminiferal species that occur at only one locality around North and Central America (231 in the Caribbean alone), suggesting that N. antarctikos is not the only endangered foraminifera. We predict that there may be hundreds or thousands of protist species that are under current threat of extinction (see Staley, 1997).
The data are presented in Table 1 and Figs. 3 and 4.
AH, SDH, and SSB conceived the study. SPA, SDH, JP, and SSB collected samples and performed morphological identifications. AH performed the molecular analyses. AH, AJG, SDH, and SSB wrote the manuscript. AH, SSB, and SPA created the figures.
The contact author has declared that none of the authors has any competing interests.
Publisher's note: Copernicus Publications remains neutral with regard to jurisdictional claims made in the text, published maps, institutional affiliations, or any other geographical representation in this paper. While Copernicus Publications makes every effort to include appropriate place names, the final responsibility lies with the authors.
This article is part of the special issue “Advances and challenges in modern and benthic foraminifera research: a special issue dedicated to Professor John Murray”. It is a result of the Forams 2023, International Symposium on Foraminifera, Perugia, Italy, 26 to 30 June 2023.
We are indebted to Wojtek Majewski, Bruce W. Hayward, and an anonymous reviewer for providing helpful comments on the manuscript. We thank Callie English and Sarah Broderick for laboratory assistance; Joan M. Bernhard, Claire Beynon, Jack Harris, Tina King, Sergei Korsun, Molly F. Miller, Laura W. Parfrey, Laura Von Rosk, and Sally E. Walker for fieldwork; and Doug Coons, Steve Clabuesch, Hilary Hudson, Phil Forte, Gogi Gwardschaladse, Shawn Harper, David Huang, Henry Kaiser, Neal W. Pollock, Cecil Shin, Karen Sterling, and Danielle Woodward for research diving in extreme conditions. We thank the captain and crew of the US Coast Guard icebreaker Polar Star, the National Science Foundation Office of Polar Programs, Raytheon Polar Services Corporation, and Petroleum Helicopters Incorporated for financial and logistic support. ANDRILL samples were generously provided by David Harwood and collected during the 2005 sampling season of NSF OPP 0342484. This paper is dedicated to the memory of John Murray, our beloved colleague and friend.
This research has been supported by the US National Science Foundation's Directorate for Geosciences (grant nos. OPP 972583, OPP 0003639, ANT 0440769, and ANT 0944646).
This paper was edited by Malcolm Hart and reviewed by Bruce Hayward and one anonymous referee.
Altenbach, A. V., Pflaumann, U., Schiebel, R., Thies, A., Timm, S., and Trauth, M.: Scaling percentages and distributional patterns of benthic foraminifera with flux rates of organic carbon, J. Foramin. Res., 29, 173–185, 1999.
Alve, E. and Goldstein, S. T.: Propagule transport as a key method of dispersal in benthic foraminifera (Protista), Limnol. Oceanogr., 48, 2163–2170, https://doi.org/10.4319/lo.2003.48.6.2163, 2003.
Alve, E. and Goldstein, S. T.: Dispersal, survival and delayed growth of benthic foraminiferal propagules, J. Sea Res., 63, 36–51, https://doi.org/10.1016/j.seares.2009.09.003, 2010.
Bowser, S. S.: Invasive activity of Allogromia pseudopodial networks: Skyllocytosis of a gelatin/agar gel, J. Protozool., 32, 9–12, https://doi.org/10.1111/j.1550-7408.1985.tb03005.x ,1985.
Bowser, S. S., Gooday, A. J., Alexander, S. P., and Bernhard, J. M.: Larger agglutinated foraminifera of McMurdo Sound, Antarctica: Are Astrammina rara and Notodendrodes antarctikos allogromiids incognito?, Mar. Micropaleontol., 26, 75–88, https://doi.org/10.1016/0377-8398(95)00024-0, 1995a.
Bowser, S. S., Kinoshita, R. K., Bernhard, J. M., and Hayden, J. H.: Agglutinated test morphogenesis in Astrammina rara, a giant foraminiferan protist from Explorers Cove, McMurdo Sound, Ant. J. US., 30, 161–162, https://doi.org/10.2113/0320364, 1995b.
Bowser, S. S., Bernhard, J. M., Habura, A., and Gooday, A. J.: Structure, taxonomy and ecology of Astrammina triangularis (Earland), an allogromiid-like agglutinated foraminifer from Explorers Cove, Antarctica, J. Foramin. Res., 32, 364–374, https://doi.org/10.2113/0320364, 2002.
Bowser, S. S., Habura, A., and Pawlowski, J.: Molecular evolution of Foraminifera, in: Genome Evolution in Eukaryotic Microbes, edited by: Katz, L. A. and Bhattacharya, D., Oxford University Press, 78–95, https://doi.org/10.1093/oso/9780198569749.003.0006, 2006.
Burns, J. A., Becker, K. P., Casagrande, D., Daniels, J., Roberts, P., Orenstein, E., Vogt, D. M., Teoh, Z. E., Wood, R., Yin, A. H., Genot, B., Gruber, D. F., Katija, K., Wood, R. J., and Phillips, B. T.: An in situ digital synthesis strategy for the discovery and description of ocean life, Sci. Adv., 10, eadj4960, https://doi.org/10.1126/sciadv.adj4960, 2024.
Buzas, M. A. and Culver, S. J.: Assembly, disassembly, and balance in marine paleocommunities, Palaios, 13, 263–275, https://doi.org/10.2307/3515449, 1998.
Broach, K., Miller, M. F., and Bowser, S. S.: Bioturbation by the common Antarctic scallop (Adamussium colbecki) and ophiuroid (Ophionotus victoriae) under multi-year sea ice: Ecologic and stratigraphic implications, Palaios, 31, 1–11, https://doi.org/10.2110/palo.2015.069, 2016.
Cedhagen, T.: Taxonomy and biology of Pelosina arborescens with comparative notes on Astrorhiza limicola (Foraminiferida), Ophelia, 37, 143–162, https://doi.org/10.1080/00785326.1993.10429914, 1993.
Conway, H., Hall, B. L., Denton, G. H., Gades, A. M. and Waddington, A. D.: Past and future grounding-line retreat of the West Antarctic Ice Sheet, Science, 286, 580–583, https://doi.org/10.1126/science.286.5438.280, 1999.
Cornelius, N. and Gooday, A. J.: “Live” (stained) deep-sea benthic foraminifera in the western Weddell Sea: trends in abundance, diversity and taxonomic composition in relation to water depth, Deep-sea Res. Pt. II, 51, 1571–1602, https://doi.org/10.1016/j.dsr2.2004.06.024, 2004.
Culver, S. J. and Buzas, M. A.: Patterns of occurrence of benthic foraminifera in time and space, in: The Adequacy of the Fossil Record, edited by: Donovan, S. K. and Paul, C. R. C., New York: Cambridge Univ. Press, New York, 207–226, ISBN-13: 978-0471969884, 1998.
Daly M., Rack F., and Zook, R.: Edwardsiella andrillae, a new species of Sea Anemone from Antarctic ice, PLOS ONE, 8, e83476, https://doi.org/10.1371/journal.pone.0083476, 2013.
Dayton, P. K. and Oliver, J. S.: Antarctic soft-bottom benthos in oligotrophic and eutrophic environments, Science, 197, 55–58, https://doi.org/10.1126/science.197.4298.55, 1977.
DeLaca, T. E.: Use of dissolved amino acids by the foraminifer, Notodendrodes antarctikos, Amer. Zool., 22, 683–690, 1982.
DeLaca, T. E., Lipps, J. H., and Hessler, R.: The morphology and ecology of a new large agglutinated Antarctic foraminifer (Textulariina: Notodendrodidae nov.), Zool. J. Linn. Soc.-Lond., 69, 205–224, https://doi.org/10.1111/j.1096-3642.1980.tb01123.x, 1980.
DeLaca, T. E., Karl, D. M., and Lipps, J. H.: The use of dissolved organic carbon by agglutinated benthic foraminifera, Nature, 289, 287–289, https://doi.org/10.1038/289287a0, 1981.
DeLaca, T. E., Bernhard, J. M., Reilly, A. A., and Bowser, S. S.: Notodendrodes hyalinosphaira (sp. nov.): Structure and autecology of an allogromiid-like agglutinated foraminifer, J. Foramin. Res., 32, 177–187, https://doi.org/10.2113/0320177, 2002.
Finlay, B. J. and Fenchel, T.: Cosmopolitan metapopulations of free-living eukaryotes, Protist, 155, 237–244, https://doi.org/10.1078/143446104774199619, 2004.
Foissner, W.: Biogeography and dispersal of micro-organisms: a review emphasizing protists, Acta Protozool., 45, 111–136, 2006.
Gooday, A. J. and Jorissen, F. J.: Benthic foraminiferal biogeography: Controls on global distribution patterns in deep-water settings, Annu. Rev. Mar. Sci., 4, 237–262, https://doi.org/10.1146/annurev-marine-120709-142737, 2012.
Gooday, A. J., Bowser, S. S., and Bernhard, J. M.: Benthic foraminiferal assemblages in Explorers Cove, Antarctica: A shallow-water site with deep-sea characteristics, Prog. Oceanogr., 37, 117–166, https://doi.org/10.1016/S0079-6611(96)00007-9, 1996.
Gooday, A. J., Cedhagen, T., Kamenskaya, O. E., and Cornelius, N.: The biodiversity and biogeography of komokiaceans and other enigmatic foraminiferan-like protists in the deep Southern Ocean, Deep-Sea Res. Pt. II, 54, 1691–1719, https://doi.org/10.1016/j.dsr2.2007.07.003, 2007.
Gooday, A. J., Rothe, N., Bowser, S. S., and Pawlowski, J.: Benthic Foraminifera, in: Biogeographic Atlas of the Southern Ocean, edited by: De Broyer C., Koubbi P., Griffiths H. J., Raymond B., Udekem d'Acoz, C. Van de Putte, A. P., Danis, B., David, B., Grant, S., Gutt, J., Held, C., Hosie, G., Huettmann F., Post, A., and Ropert-Coudert, Y., Scientific Committee on Antarctic Research, Cambridge, 74-82, ISBN-13: 978-0948277283, 2014.
Gupta, B. K. S. and Smith, L. E.: Modern benthic foraminifera of the Gulf of Mexico: a census report, J. Foramin. Res., 40, 247–265, https://doi.org/10.2113/gsjfr.40.3.247, 2010.
Habura, A., Pawlowski, J., Hanes, S. D., and Bowser, S. S.: Unexpected foraminiferal diversity revealed by small-subunit rDNA analysis of Antarctic sediment, J. Eukaryot. Microbiol., 51, 173–179, https://doi.org/10.1111/j.1550-7408.2004.tb00542.x, 2004a.
Habura, A., Rosen, D. R., and Bowser, S. S.: Predicted secondary structure of the foraminiferal SSU 3′ major domain reveals a molecular synapomorphy for granuloreticulosean protists, J. Eukaryot. Microbiol., 51, 464–471, https://doi.org/10.1111/j.1550-7408.2004.tb00397.x, 2004b.
Habura, A., Goldstein, S. T., Broderick, S., and Bowser, S. S.: A bush, not a tree: The extraordinary diversity of cold-water basal foraminiferans extends to warm-water environments, Limnol. Oceanogr., 53, 1339–1351, https://doi.org/10.2307/40058256, 2008.
Hancock, L. G., Walker, S. E., Perez-Huerta, A., and Bowser, S. S.: Population dynamics and parasite load of a foraminifer on its scallop host with their carbonate biomass contributions, PLOS ONE, 10, e0132534, https://doi.org/10.1371/journal.pone.0132534, 2015.
Hayward, B. W. and Gordon, D. P.: A new species of the agglutinated foraminifer Jullienella (Schizamminidae) from New Zealand, J. Foramin. Res., 14, 111–114, https://doi.org/10.2113/gsjfr.14.2.111, 1984.
Hayward, B. W. and Holzmann, M.: Biogeography and species durations of selected Cenozoic shallow and deep-water smaller calcareous benthic foraminifera – A review, J. Foramin. Res., 53, 192–213, https://doi.org/10.2113/gsjfr.53.3.192, 2023.
Hayward, B. W., Holzmann, M., Pawlowski, J., Parker, J. Kaushik, T., Toyofuku, M., and Tsuchiya, M.: Molecular and morphological taxonomy of living Ammonia and related taxa (Foraminifera) and their biogeography, Micropaleontology, 67, 109–313, https://doi.org/10.47894/mpal.67.2-3.01, 2021.
Kamenskaya, O. E., Bagirov, N. E., and Simdianov, T. G.: Luffammina atlantica gen. et sp. nov. (Foraminiferida, Arboramminidae) from the hydrothermal area rainbow (Mid-Atlantic Ridge), in: Adaptational aspects of the evolution of marine Fauna, edited by: Kuznetsov, A. P. and Zezina, O. N., Collected Proceedings, VNIRO Publishers, Moscow, 144–152, 2002.
Langer, M., Weinmann, A. E., Makled, W. A., Könen, J., and Gooday, A. J.: New observations on test architecture and construction of Jullienella foetida Schlumberger, 1890, the largest shallow-water agglutinated foraminifer in modern oceans, PeerJ, 10, e12884, https://doi.org/10.7717/peerj.12884, 2022.
Majewski, W., Bowser, S. S., and Pawlowski, J.: Widespread intra-specific genetic homogeneity of coastal Antarctic benthic Foraminifera, Polar Biol., 38, 2047–2058, https://doi.org/10.1007/s00300-015-1765-1, 2015.
McClain, C. R. and Hardy, S. M.: The dynamics of biogeographic ranges in the deep sea, P. R. Soc. B, 277, 3533–3546, https://doi.org/10.1098/rspb.2010.1057, 2010.
McClintock, J. B., Tackett, L. B., and Bowser, S. S.: Video observations on non-swimming valve-claps in the Antarctic scallop Adamussium colbecki, Ant. Sci., 22, 173–174, https://doi.org/10.1017/S0954102009990757, 2010.
McIlroy, D., Green, O. R., and Brasier, M. D.: Palaeobiology and evolution of the earliest agglutinated Foraminifera: Platysolenites, Spirosolenites and related forms, Lethaia, 34, 13–29, https://doi.org/10.1080/002411601300068170, 2001.
Miller, M. F., Fan, Z., and Bowser, S. S.: Sediments beneath multi-year sea ice: Delivery by deltaic and aeolian processes, J. Sediment. Res., 85, 301–314, https://doi.org/10.2110/jsr.2015.20, 2015.
Murray, J.: Ecology and Applications of Benthic Foraminifera, Cambridge University Press, Cambridge, UK, ISBN-13: 978-0-521-8283-0, 2006.
Murray, J. W.: Living benthic foraminifera: biogeographical distributions and the significance of rare morphospecies, J. Micropalaeontol., 32, 1–58, https://doi.org/10.1144/jmpaleo2012-010, 2013.
Nørvang, A.: Schizamminidae, a new family of foraminifera, Atlantide Reports, 6, 169–201, 1961.
Pawlowski, J. and Holzmann, M.: Diversity and geographic distribution of benthic foraminifera: a molecular perspective, Biodivers. Conserv., 17, 317–328, https://doi.org/10.1007/s10531-007-9253-8, 2008.
Pawlowski, J., Fahrni, J., and Bowser, S. S.: Phylogenetic analysis and genetic diversity of Notodendrodes hyalinosphaira, J. Foramin. Res., 32, 173–176, https://doi.org/10.2113/0320173, 2002a.
Pawlowski, J., Fahrni, J. F., Brykczynska, U., Habura, A., and Bowser, S. S.: Molecular data reveal high taxonomic diversity of allogromiid foraminifera in Explorers Cove (McMurdo Sound, Antarctica), Polar Biol., 25, 96–105, https://doi.org/10.1007/s003000100317, 2002b.
Pawlowski, J., Fahrni, J. F., Guiard, J., Conlan, K., Hardecker, J., Habura, A., and Bowser, S. S.: Allogromiid foraminifera and gromiids from under the Ross Ice Shelf: morphological and molecular diversity, Polar Biol., 28, 514–522, https://doi.org/10.1007/s00300-005-0717-6, 2005.
Pawlowski, J., Fontaine, D., Aranda da Silva, A., and Guiard, J.: Novel lineages of Southern Ocean deep-sea foraminifera revealed by environmental DNA sequencing, Deep-Sea Res. Pt. II, 58, 1996–2003, https://doi.org/10.1016/j.dsr2.2011.01.009, 2011.
Pawlowski, J., Adl, S., Audic, S., Bass, D., Belbahri, L., Berney, C., Bowser, S. S., Cepicka, I., Decelle, J., Dunthorn, M., Fiore, A. M., Gile, G. H., Holzmann, M., Jahn, R., Keeling, P., Kostka, M., Kudryavtsev, A., Lara, E., Le Gall. L,, Lukes, J., Mann, D., Mitchell, E., Nitsche, F., Romeralo, M., Saunders, G. W., Simpson, A. G. B., Smirnov, A.V., Spouge, J., Stern, R. F., Stoeck, T., Zimmermann, J., Schindel, D., and de Vargas, C.: CBOL Protist Working Group: Barcoding eukaryotic richness beyond the animal, plant and fungal kingdoms, PLOS Biol., 10, e1001419, https://doi.org/10.1371/journal.pbio.1001419, 2012.
Pedrós-Alió, C.: Marine microbial diversity: can it be determined?, Trends Microbiol., 14, 257–263, https://doi.org/10.1016/j.tim.2006.04.007, 2006.
Pollock, N. W. and Bowser, S. S.: Scuba collection of benthic foraminifera in Explorers Cove, Antarctica: An accessible model of the deep-ocean benthos?, in: Diving for Science...1995: Proceedings of the American Academy of Underwater Sciences Fifteenth Annual Scientific Diving Symposium, edited by: Harper, D. E., American Academy of Underwater Sciences, Scripps Institution of Oceanography, La Jolla, California, USA, 4–8 October, 63–74, 1995.
Prothro, L. O., Majewski, W., Yokoyama, Y., Simkins, L. M., Anderson, J. B., Yamane, M., Miyairi, Y., and Ohkouchi, N.: Timing and pathways of East Antarctic Ice Sheet retreat, Quaternary Sci. Rev., 230, 106166, https://doi.org/10.1016/j.quascirev.2020.106166, 2020.
Sen Gupta, B. K. and Smith, L. E.: Modern benthic foraminifera of the Gulf of Mexico: A census report, J. Foramin. Res., 40, 247–265, https://doi.org/10.2113/gsjfr.40.3.247, 2010.
Shires, R., Gooday, A. J., and Jones, A. R.: A new large agglutinated foraminifer (Arboramminidae n. fam.) from an oligotrophic site in the abyssal Northeast Atlantic, J. Foramin. Res., 24, 149–157, https://doi.org/10.2113/gsjfr.24.3.149, 1994.
Staley, J. T.: Biodiversity: are microbial species threatened?, Curr. Opin. Biotech., 8, 340–345, https://doi.org/10.1016/S0958-1669(97)80014-6, 1997.
Suhr, S. B., Alexander, S. P., Gooday, A. J., Pond, D. W., and Bowser, S. S.: Trophic modes of large Antarctic Foraminifera: Roles of carnivory, omnivory, and detritivory, Mar. Ecol. Prog. Ser., 371, 155–164, https://doi.org/10.3354/meps07693, 2008.
Tendal, O. S. and Cedhagen, T.: Schizammina andamana n. sp., a large foraminiferan (Protozoa, Granuloreticulosa) from the shelf west of Thailand, Phuket Marine Biological Center Research Bulletin, 68, 11–19, https://api.semanticscholar.org/CorpusID:73637744 (last access: 16 August 2024), 2007.
Travis, J. L., Welnhofer, E. A., and Orokos, D. D.; Autonomous reorganization of foraminiferan reticulopodia, J. Foramin. Res., 32, 425–433, https://doi.org/10.2113/0320425, 2002.
Violanti, D.: Taxonomy and distribution of recent benthic foraminifers from Terra Nova Bay (Ross Sea, Antarctica), Oceanographic Campaign 1987/1988, Paleontographia Italica, 83, 25–71, 1996.
Vogel, S.: Life in Moving Fluids, 2nd Edn., Princeton University Press, Princeton, New Jersey, USA, 467 pp., ISBN: 0-691-03485-0, 1994.