the Creative Commons Attribution 4.0 License.
the Creative Commons Attribution 4.0 License.
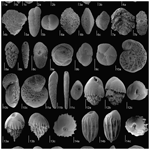
Late Miocene to Early Pliocene benthic foraminifera from the Tasman Sea (International Ocean Discovery Program Site U1506)
Maria Elena Gastaldello
Claudia Agnini
Laia Alegret
Modern and fossil benthic foraminifera have been widely documented from New Zealand, but detailed studies of material collected from drilling expeditions in the Tasman Sea are scarcer. This study aims to provide an updated taxonomic study for the Late Miocene–Early Pliocene benthic foraminifera in the Tasman Sea, with a specific focus on the paleoceanographic phenomenon known as the Biogenic Bloom. To achieve these goals, we analysed 66 samples from International Ocean Discovery Program (IODP) Site U1506 located in the Tasman Sea and identified a total of 98 taxa. Benthic foraminifera exhibit good preservation, allowing for accurate taxonomic identification. The resulting dataset serves as a reliable and precise framework for the identification and classification of the common deep-water benthic foraminifera in the region. The paleobathymetric analysis based on depth-dependent species indicates deposition at lower bathyal depths. Additionally, the quantitative analysis of the benthic foraminiferal assemblages allowed us to explore their response to the Biogenic Bloom at Site U1506. The paleoenvironmental analysis, focused on the Early Pliocene part of the Biogenic Bloom, points to high-productivity conditions driven by phytoplankton blooms and intensified vertical mixing of the ocean waters. These results provide valuable insights into the paleoceanographic events in the Tasman Sea, particularly the Biogenic Bloom, highlighting the significance of benthic foraminifera as reliable proxies for deciphering paleoenvironmental conditions. The taxonomic identifications and paleoenvironmental interpretations presented herein will aid in future paleoceanographic studies and facilitate comparisons with other deep-sea regions.
- Article
(14538 KB) - Full-text XML
-
Supplement
(393 KB) - BibTeX
- EndNote
Multiple studies have focused on recent (e.g. Vella, 1957; Eade, 1967; Hayward et al., 1999; Kawagata, 2001; Hayward et al., 2010, and references therein; Martin et al., 2010; Hayward et al., 2013) and fossil benthic foraminifera in New Zealand (e.g. Boersma, 1984a; Hornibrook et al., 1989; Nees, 1994, 1997; Kawagata, 1999; Nees et al., 1999; Hayward, 2002, and references therein; Hayward, 2004; Hayward et al., 2004; Mancin et al., 2013); however, fewer studies have dealt with material collected during ocean drilling expeditions in the Tasman Sea (Boersma, 1986; Kurihara and Kennett, 1986, 1988, 1992; Hayward, 2002; Kender et al., 2016; Kawagata and Kamahashi, 2016; Alegret et al., 2021).
The Tasman Sea has been the focus of three drilling expeditions since the 1970s, namely Deep Sea Drilling Project (DSDP) Leg 21, DSDP Leg 29, and International Ocean Discovery Program (IODP) Expedition 371. Hayward and Buzas (1979) documented the taxonomy and paleoecology of Early Miocene benthic foraminifera from DSDP Site 206 in the New Caledonia Basin. Kurihara and Kennett (1986, 1988, 1992) reported Miocene to recent benthic foraminifera from DSDP Site 206, along with DSDP Sites 588, 590, and 591 in the Lord Howe Rise, to evaluate the paleoceanography of the Tasman Sea (Fig. 1). Boersma (1986) studied Eocene to Pliocene benthic foraminifera from seven DSDP sites from Leg 90 (586B, 587, 588, 589, 590, 591, and 594). Kender et al. (2016) documented the benthic foraminiferal extinction and paleoenvironmental changes during the Middle Pleistocene at DSDP Site 593 in the Challenger Plateau. More recently, benthic foraminifera recovered during IODP Expedition 371 in the Tasman Sea were documented in the expedition reports (Sutherland et al., 2019b). Their paleodepth interpretation was summarised by Sutherland et al. (2020), and their assemblages are being currently studied in detail, with two quantitative studies published so far on Eocene (Alegret et al., 2021) and Miocene–Pliocene benthic foraminifera (Gastaldello et al., 2023a).
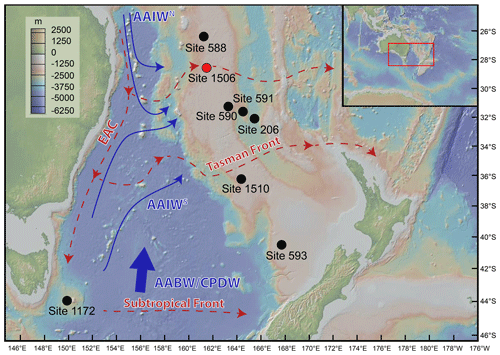
Figure 1Map of ODP sites where benthic foraminifera assemblages have been studied in the Tasman Sea: DSDP Site 206 (Hayward and Buzas, 1979; Kurihara and Kennett, 1986, 1988, 1992); DSDP Sites 588, 590, and 591 (Kurihara and Kennett, 1986, 1988, 1992); DSDP Site 593 (Kender et al., 2016); IODP Site U1506 (Gastaldello et al., 2023a); and IODP Site U1510 (Alegret et al., 2021). Arrows represent present-day circulation patterns in the Tasman Sea (modified after Gastaldello et al., 2023a). Surface water circulation (dotted red arrows) includes the East Australian Current, the Tasman Front, and the Subtropical Front; intermediate waters (thin blue arrows) include the north (AAIWN) and the south (AAIWS) Antarctic Intermediate Water. Deep waters (thick blue arrow) include the Antarctic Bottom Water and the Circumpolar Deep Water. Figure made with GeoMapApp (http://www.geomapapp.org, last access: 3 January 2024).
Gastaldello et al. (2023a) focused on the record of the Late Miocene–Early Pliocene Biogenic Bloom at Site U1506 in the Tasman Sea. This paleoceanographic phenomenon occurred between 9 and 3.5 Ma (Farrell et al., 1995; Dickens and Owen, 1999; Diester-Haass et al., 2005) and is characterised by a substantial increase in marine primary productivity, primarily observed in upwelling regions of the Indian and Pacific oceans (Peterson et al., 1992; Berger et al., 1993; Dickens and Owen, 1999; Grant and Dickens, 2002; Lyle et al., 2019). It is important to acknowledge that most quantitative studies on benthic foraminifera in the Tasman Sea (e.g. Hayward and Buzas, 1979; Boersma, 1986; Kurihara and Kennett, 1986, 1988, 1992) pre-date the concept of the Biogenic Bloom, which was first described by Farrell et al. (1995); thus the changes observed in benthic foraminiferal assemblages in these works were not linked to the Biogenic Bloom. This phenomenon has been identified and studied in the Tasman Sea at DSDP Site 590 (Grant and Dickens, 2002), at Ocean Drilling Project (ODP) Site 1172 (Diester-Haass et al., 2006), and at IODP Site U1506 (Sutherland et al., 2022; Gastaldello et al., 2023a). However, there is still much to discover about the regional imprint of the Biogenic Bloom (Farrell et al., 1995; Dickens and Owen, 1999; Grant and Dickens, 2002; Diester-Haass et al., 2005; Drury et al., 2021), and despite the extensive documentation of this event globally (Pillot et al., 2023), our understanding of its driving mechanisms, consequences, and biotic response remains limited.
The study of benthic foraminifera provides a valuable tool for deciphering the evolution of paleoenvironmental conditions at the seafloor (Murray, 2006). Many environmental factors, including bottom water oxygenation, the flux of organic matter to the seafloor, the type and quality of organic matter, and bottom current velocity, influence the abundance of benthic foraminiferal taxa and the composition of their assemblages (Jorissen et al., 2007). These factors, in turn, are shaped by complex local and regional physical, chemical, and biological parameters (Jorissen et al., 2007). In-depth studies of benthic foraminifera have the potential to unveil crucial information about paleoceanographic events such as the Biogenic Bloom, and a strong taxonomic framework and correct species identification are critical for paleoenvironmental interpretations and for comparison with other locations.
The main objectives of this study are twofold. First, we aim to provide an updated taxonomic assessment for the most common benthic foraminifera found in the Tasman Sea during the Late Miocene–Early Pliocene. This includes examining their distribution in terms of biogeography and temporal patterns. Secondly, we aim to refine the quantitative studies on benthic foraminifera at Site U1506 and to improve the previously made paleoenvironmental and paleoceanographic interpretations from Gastaldello et al. (2023a) to provide new insight into the Biogenic Bloom phenomenon in this region.
Site U1506 (28.66∘ S, 161.74∘ E; 1505 m water depth) was drilled during IODP Expedition 371 in the Tasman Sea (Fig. 1). The drilling operations took place on the northern part of the Lord Howe Rise in the south-western Pacific Ocean (Sutherland et al., 2019a). The paleodepth at Site U1506 from the late Oligocene to the Pleistocene is inferred to have been consistently lower bathyal, 1000–2000 m (Sutherland et al., 2019b). Our study spans a ∼ 140 m thick succession of foraminiferal-bearing nannofossil ooze/chalk from Hole 1506A, covering the Late Miocene to Early Pliocene time interval. Data are plotted on the CSF-A depth scale (core depth below sea floor A; m CSF-A from here on referred to as m). The age model for Site U1506 was developed using orbital tuning of the natural gamma radiation, benthic foraminiferal oxygen isotopes, and calcareous nannofossil biostratigraphy (Gastaldello et al., 2023a).
In this study, benthic foraminifera were analysed in a total of 66 samples from Core U1506A-24R-5W, 64-66 cm (221.75 m) to Core U1506A-10R-1W, 100–102 cm (82.01 m) (Supplement Table S1). The average sampling resolution is 2.2 m, which equals about 40 kyr. The limited recovery of Core 15 results in the lowest sampling resolution between 130 and 140 m (core recovery ∼ 7 %; Sutherland et al., 2019b). We revised the taxonomic identifications of the benthic foraminifera analysed in Gastaldello et al. (2023a), and we re-analysed the already-examined residues and the benthic assemblages of five samples between 144.17 and 82.01 m (U1506A-10R-1W, 100–102 cm; U1506A-11R-1W, 105–107 cm; U1506A-11R-3W, 130–132 cm; U1506A-12R-1W, 110–112 cm; U1506A-16R-4W, 126–128 cm). Two additional samples from Site U1506 were prepared and analysed for this study (U1506A-11R-4W, 80–82 cm at 96.05 m and U1506A-11R-3W, 30–32 cm at 94.05 m).
Samples were dried in an oven at 40 ∘C and weighed to obtain bulk dry sample weight. Subsequently, the samples were disaggregated in water with diluted (NaPO3)6, sieved to separate the fraction larger than 63 µm, and dried at 40 ∘C. For each sample, approximately 300 specimens of benthic foraminifera larger than 63 µm were picked and mounted on microslides for permanent record stored at the University of Zaragoza. A total of 98 taxa (84 calcareous and 14 agglutinated) were recognised at the species or higher taxonomic level. Species identification follows Van Morkhoven et al. (1986), Hermelin (1989), Hayward and Buzas (1979), Hayward et al. (2010, 2013), Kaminski and Gradstein (2005), and Holbourn et al. (2013).
All taxa were allocated to epifaunal or infaunal morphogroups based on their morphology following Corliss (1991), Jones and Charnock (1985), and Corliss and Chen (1988) (Fig. 2). The epifaunal–infaunal ratio was used as a proxy for oxygen levels and trophic conditions on the sea bed, with high relative abundances of infaunal morphogroups associated with eutrophic and/or low oxygen paleoenvironments and oligotrophic conditions and/or high oxygen availability commonly linked to assemblages dominated by epifaunal morphogroups (Jorissen et al., 1995, 2007). The percentage of agglutinated and calcareous taxa was calculated (Fig. 2). To interpret shifts in paleoenvironmental conditions, species with specific paleoecological preferences were categorised as suboxic–dysoxic species (Bernhard, 1986; Kaiho, 1991), opportunistic species (Smart et al., 1994; Erbacher et al., 1999; Fontanier et al., 2002; Hayward et al., 2005; Boscolo Galazzo et al., 2015; Dias et al., 2018), and phytodetritus-exploiting taxa (PETs; Gooday, 1988, 1993; Suhr et al., 2003; Jorissen et al., 2007).
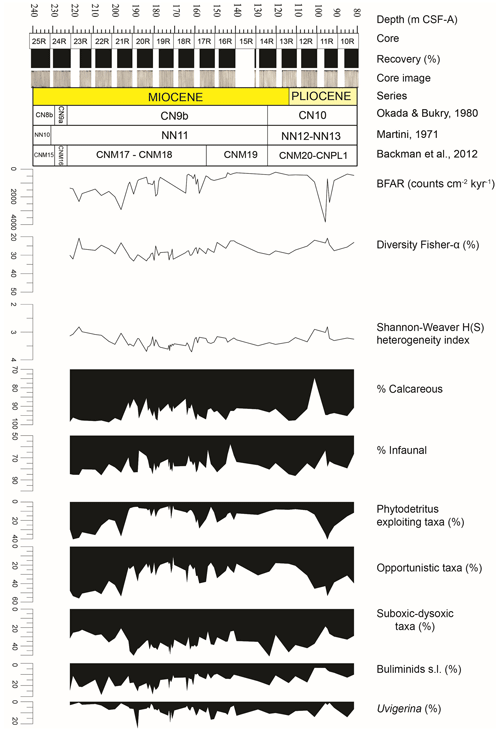
Figure 2Results of quantitative analyses of benthic foraminiferal assemblages across the Late Miocene–Early Pliocene at IODP Site U1506 plotted against depth (m CSF-A). BFARs: benthic foraminiferal accumulation rates. At the top: depth (m CSF-A), core, core recovery (%), and core images. Calcareous nannofossil biozonations (Martini, 1971; Okada and Bukry, 1980; Backman et al., 2012) and chronostratigraphy are also reported.
To assess changes in diversity and heterogeneity within the benthic foraminiferal assemblages, we calculated the Fisher α index (Fisher et al., 1943) and the Shannon–Weaver heterogeneity index H(S) (Shannon, 1948). The benthic foraminiferal accumulation rates (BFARs) were calculated using the equation proposed by Herguera and Berger (1991):
The BFAR is expressed as counts cm−2 kyr−1 (Supplement Table S2), “nr g−1 > 63 µm” is the number of benthic foraminifera per gram of sediment > 63 µm, “wt % > 63” is the weight percent of the sample > 63 µm, LSR is the linear sedimentation rate, and DBD is dry bulk density data (Sutherland et al., 2019b). BFAR is considered to be an indicator of export productivity from the surface, and it is used as a proxy for the delivery of food to the seafloor, which is a limiting factor for benthic foraminiferal productivity in the deep ocean (Gooday, 2003; Jorissen et al., 2007).
Species having a relative abundance exceeding > 2 % in at least one sample (Fig. 3) were described, and the most representative specimens of each species were imaged with a scanning electron microscope at the University of Zaragoza (Zeiss Merlin) and the University of Padova (TESCAN SOLARIS) (Figs. 4 to 7). The specimens illustrated are deposited at the Natural Science Museum of the University of Zaragoza (Spain, repository numbers MPZ-2023/333 to MPZ-2023/371).
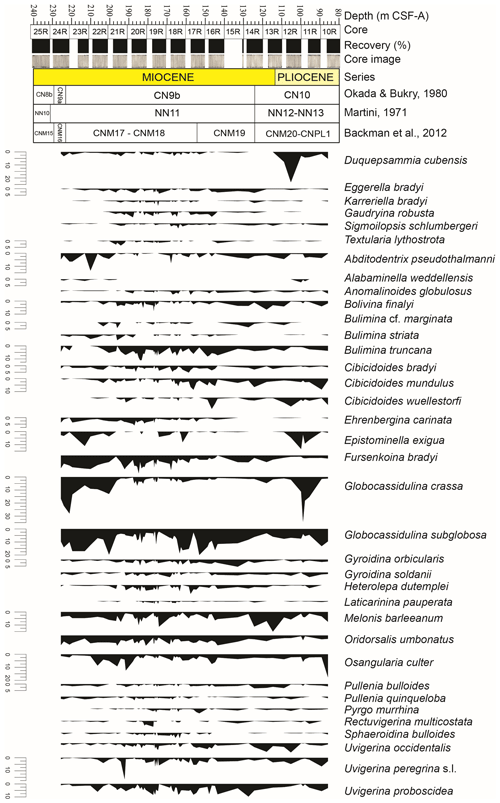
Figure 3Relative abundance (%) of selected benthic foraminiferal taxa (> 2 %) at IODP Site U1506 against depth. At the top: depth (m CSF-A), core, core recovery (%), and core images. Calcareous nannofossil biozonations (Martini, 1971; Okada and Bukry, 1980; Backman et al., 2012) and chronostratigraphy are also reported.
For taxonomic comparison with the type material, one of us (Laia Alegret) visited the National Paleontological Collection at GNS Science (Geological and Nuclear Sciences) in Lower Hutt, Wellington (New Zealand); the Cushman Collection at the Smithsonian Institution, National Museum of Natural History (USNM) in Washington DC (USA); and the foraminifera collection at the Natural History Museum (NHM) in London. We compared the specimens examined at these institutions with those found in our samples, enhancing the reliability of our taxonomic investigation.
The bathymetric terminology follows Van Morkhoven et al. (1986) and Hayward et al. (2010): inner shelf (0–50 m), middle shelf (50–100 m), outer shelf (100–200 m), upper bathyal (200–600 m), middle bathyal (600–1000 m), lower bathyal (1000–2000 m), abyssal (2000–6000 m).
3.1 Benthic foraminifera assemblages
Benthic foraminifera are abundant, the tests are well preserved, and most specimens show fine surface details. All species constituting more than 2 % of the assemblages in at least one sample (36 species in total) are described and commented on in this study (Fig. 3). The diversity of the assemblages (Fisher α index) ranges between 20 and 33. It generally reaches higher values in the lowermost part of the studied interval between 191.36 and 170.68 m, and it gradually decreases upwards, with a second minor increase between 134.46 and 107.91 m. The Shannon–Weaver heterogeneity index H(S) ranges between 2.8 and 3.7. It reaches higher values between 185.94 and 161.49 m and a minimum value in the upper part at around 95.05 m (Fig. 2).
At Site U1506, calcareous benthic foraminifera make up 75 %–98 % of the assemblages, and the abundance of agglutinated taxa (on average 5 %–10 % within the assemblage) reaches up to 25 % in only one sample (U1506A-12R-1, 110–112 cm, 101.41 m). Infaunal morphogroups dominate over the epifaunal ones, and their abundance typically ranges between 65 % and 85 % (Fig. 2). The infaunal group includes taxa such as Bulimina truncana, Fursenkoina bradyi, Globocassidulina crassa, Globocassidulina subglobosa, Oridorsalis umbonatus, and Uvigerina proboscidea, as well as the calcareous elongate uniserial foraminifera (e.g. Nodosaria and Stilostomella groups). All the agglutinated foraminifera recovered at Site U1506 belong to infaunal morphogroups (e.g. Eggerella, Karreriella, Textularia). Only one sample (U1506A-16R-3, 140–142 cm, 142.81 m) contains more than 40 % of epifaunal taxa, mainly Cibicidoides wuellestorfi, Cibicidoides mundulus, and Osangularia culter.
Overall BFARs show high values (ca. 800–1000) in the lower and top parts of the study interval, with the highest values (ca. 2000–3000) recorded between 98.73 and 92.91 m. The lowest values (ca. 200–400) are recorded between 144.61 and 103.21 m.
3.2 Taxonomy
This section presents systematic descriptions of the species whose relative abundance is > 2 % in at least one sample across the study interval at Site U1506. For each species, we included a synonymy list, taxonomic details, descriptions, remarks on taxonomy, and paleoecological and paleobathymetric notes.
-
Family Duquepsammiidae Seiglie and Baker, 1987
-
Genus Duquepsammia Seiglie and Baker, 1987
-
Duquepsammia cubensis (Cushman and Bermúdez, 1937)
Fig. 4: 1a–c
-
1937 Spiroplectoides cubensis Cushman and Bermúdez, pl. 1, figs. 44–45
-
1985 Bolivinopsis cubensis (Cushman and Bermúdez); Thomas, pl. 1, fig. 1
-
1986 Bolivinopsis cubensis (Cushman and Bermúdez); Boersma et al., pl. 3, figs. 1–2
-
1989 Bolivinopsis cubensis (Cushman and Bermúdez); Hornibrook et al., pl. 15, fig. 5
-
2005 Duquepsammina cubensis (Cushman and Bermúdez); Kaminski and Gradstein, pl. 102, figs. 1–8
Description. Test free, elongate, initially planispiral, later biserial, rhomboidal in cross-section. The planispiral part of the test is wider than the biserial part. Sutures are slightly depressed, inclined at ca. 60∘ in the biserial part. The peripheral margin is acute. Wall finely agglutinated. The aperture is an interiomarginal slit.
Remarks. Despite the known stratigraphic range of D. cubensis spanning from the Late Eocene to the Late Miocene (Seiglie and Baker, 1987), the specimens found in the Early Pliocene at Site U1506 perfectly match the description by Kaminski and Gradstein (2005). Preservation ranges from moderately good (fully complete tests) to broken tests that typically miss the planispiral portion. Our specimens closely resemble Spiroplectammina spectabilis (Grzybowski, 1898) emend. Kaminski; however, S. spectabilis goes extinct at the end of the Paleocene (Kaiho et al., 1996). Moreover, the spiral part of S. spectabilis is less developed and with less chambers per whorl compared to the genus Duquepsammia (Seiglie and Baker, 1987).
Paleoecology and paleobathymetric distribution. This deep-water agglutinated taxon has been commonly documented from middle bathyal to abyssal water depths (Kaminski and Gradstein, 2005). Duquepsammina cubensis has been documented in sediments of lower–middle bathyal depths from the Chatham Rise, east of New Zealand (Kennett and Casey, 1969).
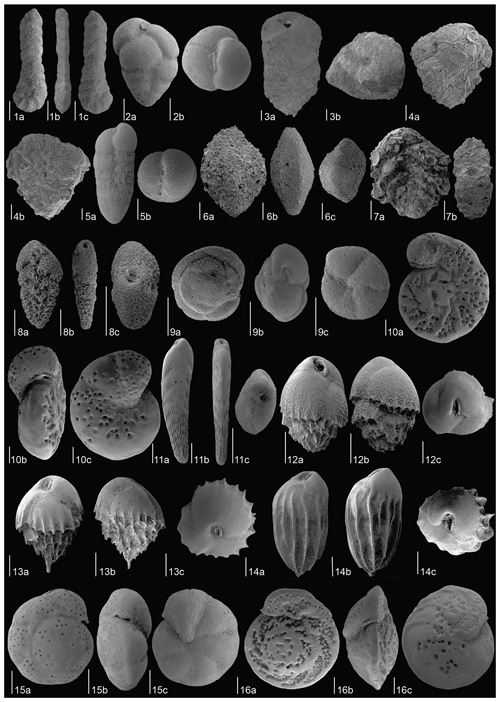
Figure 4SEM images of the most common benthic foraminiferal species at Site U1506 identified in the Late Miocene–Early Pliocene. (1) Duquepsammia cubensis (sample U1506A-11R-1W, 105–107 cm), MCNUZ-MPZ-2023/338: (a) frontal side, (b) side view, (c) dorsal side. (2) Eggerella bradyi (sample U1506A-21R-2W, 50–52 cm), MCNUZ-MPZ-2023/333: (a) frontal side, (b) outline view. (3) Karreriella bradyi (sample U1506A-21R-2W, 50–52 cm), MCNUZ-MPZ-2023/334: (a) frontal side, (b) outline view. (4) Gaudryina robusta adult (sample U1506A-18R-4W, 125–127 cm), MCNUZ-MPZ-2023/335: (a) frontal side, (b) outline view. (5) Gaudryina robusta juvenile (sample U1506A-21R-1W, 140–142 cm), MCNUZ-MPZ-2023/336: (a) frontal side, (b) lateral side. (6) Sigmoilopsis schlumbergeri (sample U1506A-13R-1W, 110–112 cm), MCNUZ-MPZ-2023/337: (a) dorsal side, (b), side view, (c) outline view. (7) Textularia lythostrota (sample U1506A-21R-2W, 50–52 cm), MCNUZ-MPZ-2023/339: (a) frontal view, (b), outline view. (8) Abditrodentrix pseudothalmannii (sample U1506A-11R-1W, 105–107 cm), MCNUZ-MPZ-2023/340: (a) frontal view, (b), side view, (c) outline view. (9) Alabaminella weddellensis (sample U1506A-23R-3W, 78–80 cm), MCNUZ-MPZ-2023/341: (a) dorsal side, (b), side view, (c) ventral side. (10) Anomalinoides globulosus (sample U1506A-21R-1W, 140–142 cm), MCNUZ-MPZ-2023/342: (a) dorsal side, (b), side view, (c) ventral side. (11) Bolivina finlayi (sample U1506A-21R-1W, 140–142 cm), MCNUZ-MPZ-2023/343: (a) frontal view, (b) side view, (c) outline view. (12) Bulimina cf. marginata (sample U1506A-21R-4W, 140–142 cm), MCNUZ-MPZ-2023/345: (a) frontal side, (b) dorsal side, (c) outline view. (13) Bulimina striata (sample U1506A-19R-4W, 97–99 cm), MCNUZ-MPZ-2023/346: (a) frontal side, (b) dorsal side, (c) outline view. (14) Bulimina truncana (sample U1506A-21R-4W, 140–142 cm), MCNUZ-MPZ-2023/347: (a) frontal side, (b) dorsal side, (c) outline view. (15) Cibicidoides bradyi (sample U1506A-11R-4W, 80–82 cm), MCNUZ-MPZ-2023/348: (a) dorsal side, (b), side view, (c) ventral side. (16) Cibicidoides mundulus (sample U1506A-10R-3W, 130–132 cm), MCNUZ-MPZ-2023/349: (a) dorsal side, (b), side view, (c) ventral side. All scale bars = 100 µm, except for (9a–c), which have a scale bar = 50 µm.
-
Family Eggerellidae Cushman 1937
-
Genus Eggerella Cushman 1933
-
Eggerella bradyi (Cushman 1911)
Fig. 4: 2a–b
-
1911 Verneuilina bradyi Cushman; p. 54, fig. 87
-
1937 Eggerella bradyi (Cushman); Cushman, p. 52, pl. 5, fig. 19
-
1951 Eggerella bradyi (Cushman); Phleger and Parker, p. 6, pl. 3, figs. 1–2
-
1986 Eggerella sp. (Cushman); Kurihara and Kennett; pl. 1, figs. 4–5
-
1987 Eggerella bradyi (Cushman); Loeblich and Tappan, p. 170, pl. 189, figs. 1–2
-
1998 Eggerella bradyi (Cushman); Robertson, p. 24, pl. 3, fig. 4
-
2010 Eggerella bradyi (Cushman); Hayward et al., p. 144, pl. 5, figs. 23–24
-
2013 Eggerella bradyi (Cushman); Holbourn et al., p. 232, figs. 1–4
-
2019 Eggerella bradyi (Cushman); Ridha et al., p. 222, fig. 8: 6a–b
Description. Test nearly conical, triserial, highly inflated chambers larger in the adult portion. Chambers are separated by narrow, depressed sutures. Wall smooth and finely agglutinated. The aperture is an elongated slit close to the base of the apertural face, surrounded by a small lip.
Remarks. The morphology of specimens retrieved at Site U1506 is consistent with the description provided for the holotype (USNM PP 26281) in the original description by Cushman (1911). Hermelin (1989) reported morphologic variants of E. bradyi in the western equatorial Pacific Ocean, with tests ranging from conical to cylindrical. Specimens at Site U1506 show conical forms and good preservation.
Paleoecology and paleobathymetric distribution. Eggerella bradyi is considered to be a cosmopolitan species, mostly found at bathyal and abyssal depths (Ingle and Keller, 1980). Hayward et al. (2010) reported it to be typical at lower bathyal depths (1000–2000 m) around New Zealand.
-
Family Verneuilinidae Cushman, 1911
-
Genus Gaudryina d'Orbigny, 1839
-
Gaudryina robusta Cushman, 1913
Fig. 4: 3a–b; 4a–b
-
1913 Gaudryina robusta Cushman; p. 78, figs. 2a–c
-
1921 Gaudryina robusta Cushman; Cushman, p. 152, pl. 30, figs. 1a–c
-
1968 Gaudryina robusta Cushman; Chiji et al., pl. 7, fig. 4
Description. Large test, initially triserial and with triangular outline. In the last portion, it becomes biserial with slightly inflated chambers. Wall coarsely agglutinated. Sutures are slightly depressed. The aperture is a small opening located in the inner margin of the last chamber.
Remarks. Specimens found at Site U1506 are consistent with the original description of G. robusta by Cushman (1913). Juvenile specimens show more triangular tests with flat last chambers (Fig. 4: 4). Adult specimens present more inflated and biserial chambers in the last portion of the test (Fig. 4: 3). The preservation is fairly good, and the tests observed are typically complete.
-
Family Eggerellidae Cushman 1937
-
Genus Karreriella Cushman 1933
-
Karreriella bradyi (Cushman 1911)
Fig. 4: 5a–b
-
1911 Gaudryina bradyi Cushman; p. 67, text fig. 107
-
1921 Gaudryina bradyi Cushman; Cushman, p. 149, pl. 29, fig. 3
-
1960 Karreriella bradyi (Cushman); Barker, pl. 46, figs. 1–4
-
1994 Karreriella bradyi (Cushman); Loeblich and Tappan, p. 25, pl. 30, figs. 8–16
-
1994 Karreriella bradyi (Cushman); Jones, pl. 46, figs. 1–4
-
2002 Karreriella bradyi (Cushman); Hayward, p. 288, pl. 2, fig. 1
-
2002 Karreriella bradyi (Cushman); Kuhnt et al., p. 130, pl. 5, figs. 1–2, 14
-
2010 Karreriella bradyi (Cushman); Hayward et al., pl. 5, figs. 25–27
-
2013 Karreriella bradyi (Cushman); Holbourn et al., p. 318, figs. 1–4
Description. Test elongate, triserial in the early stage, biserial in the adult part. Chambers are inflated and divided by depressed sutures. Wall finely agglutinated. The aperture is an elongated slit at the base of the apertural face, and it is surrounded by a lip.
Remarks. The original description by Cushman (1911) and the examination of two specimens belonging to K. bradyi (Challenger Expedition, ZF1452 and ZF1453) at the NHM allow us to ascribe our specimens to this taxon. Specimens from Site U1506 are well preserved, tests are moderately large, and the last chamber tends to be more inflated as compared to the early chambers.
Paleoecology and paleobathymetric distribution. Around New Zealand, forms of K. bradyi have been found at middle bathyal to abyssal depths (500–4000 m; Hayward et al., 2010). It has been reported to typically occur in oligotrophic deep-water environments in the South China Sea (Kuhnt et al., 1999).
-
Family Hauerinidae Schwager 1876
-
Genus Sigmoilopsis Finlay, 1947
-
Sigmoilopsis schlumbergeri (Silvestri 1904)
Fig. 4: 6a–c
-
1884 Planispira celata (Costa); Brady, p. 197, pl. 8, figs. 1–4
-
1904 Sigmoilina schlumbergeri Silvestri, p. 267, 269, illustrated in Schlumberger, 1887, pl. 7, figs. 12–14, text figs. 6–7
-
1986 Sigmoilopsis schlumbergeri (Silvestri); Van Morkhoven et al., pl. 18, figs. 1a–e
-
1986 Sigmoilopsis schlumbergeri (Silvestri); Kurihara and Kennett; pl. 1, figs. 11–12
-
1987 Sigmoilopsis schlumbergeri (Silvestri); Loeblich and Tappan, pl. 356, figs. 8–13
-
1994 Sigmoilopsis schlumbergeri (Silvestri); Jones, pl. 8, figs. 1–4
-
2010 Sigmoilopsis schlumbergeri (Silvestri); Hayward et al., pl. 9, figs. 12–16
-
2013 Sigmoilopsis schlumbergeri (Silvestri); Holbourn et al., p. 506, figs. 1–2
Description. Moderately large, elongate and ovate test, relatively compressed in the side view. Initially, the chambers have a quinqueloculine arrangement, but then the angle between planes of coiling between successive chambers quickly increases, leading first to a sigmoid appearance in section. Subsequently, the arrangement shifts to a biserial pattern, where the chambers are positioned nearly opposite each other. The aperture is circular with a lip and a simple tooth, located at the end of a short neck. Wall agglutinated with calcareous cement, coated with small- to medium-sized grains.
Remarks. Our specimens are large, and the centre is typically coated by darker grains with occasionally some lighter stripes, while the periphery contains lighter grains. The wall includes sand grains and calcareous nannofossils. Most of the specimens of S. schlumbergeri observed at Site U1506 are typically broken along the periphery of the test and in the neck area. Sigmoilopsis schlumbergeri closely resembles Sigmoilopsis celata (Costa); the distinction is mainly based on the periphery and the sutures. Our specimens present defined sutures, and, in the intact specimens, an acute periphery was observed, characteristics reported for S. schlumbergeri (Van Morkhoven et al., 1986).
Paleoecology and paleobathymetric distribution. Documented commonly in middle bathyal to abyssal depths around New Zealand (Hayward et al., 2010). In the Gulf of Mexico, it has been reported to be most common in the upper part of the middle bathyal zone (Phleger and Parker, 1951).
-
Family Textulariidae Ehrenberg, 1838
-
Genus Textularia Defrance, 1824
-
Textularia lythostrota (Schwager 1866)
Fig. 4: 7a–b
-
1866 Placanium lythostrotum Schwager: p. 194, pl. 4, fig. 4a–c
-
1986 Textularia lythostrota (Schwager); Kurihara and Kennett, pl. 1, figs. 1–2
-
1989 Textularia lythostrota (Schwager); Hermelin, pl. 1, figs. 2–5
-
1994 Textularia lythostrota (Schwager); Gupta, pl.1, figs. 4, 15
-
2010 Textularia lythostrota (Schwager); Hayward et al., pl. 6, figs. 26–29
Description. Large, biserial test with maximum width and thickness close to the aperture, compressed in side view. Periphery rounded and lobulated. Chambers are at an angle of ca. 45∘, divided by deeply depressed sutures. Coarsely agglutinated wall. The aperture is a small arch situated at the base of the last chamber.
Remarks. Specimens documented at Site U1506 are characterised by large tests, and the wall is coarsely agglutinated and includes biogenic grains such as calcareous nannofossils and broken pieces of planktonic foraminifera.
-
Family Bolivinitidae Cushman 1927
-
Genus Abditodentrix Patterson 1985
-
Abditodentrix pseudothalmanni (Boltovskoy and de Kahn 1981)
Fig. 4: 8a–c
-
1981 Bolivinita pseudothalmanni Boltovskoy and Guissani De Kahn: p. 44
-
1985 Abditodentrix asketocomptella Patterson, p. 140, pl. 1, figs. 1–9
-
1987 Abditodentrix pseudothalmanni (Boltovskoy and Guissani De Kahn); Loeblich and Tappan, pl. 554, figs. 1–2
-
1989 Abditodentrix asketocomptella (Patterson); Hermelin, pl. 10, fig. 16
-
1999 Abditodentrix pseudothalmanni (Boltovskoy and Guissani De Kahn); Kawagata, figs. 4–6
-
1989 Abditodentrix asketocomptella (Patterson); Hermelin, pl. 10, fig. 16
-
2010 Abditodentrix pseudothalmanni (Boltovskoy and Guissani De Kahn); Hayward et al., pl. 17, figs. 11–12
-
2019 Abditodentrix pseudothalmanni (Boltovskoy and Guissani De Kahn); Ridha et al., fig. 3: 9a–b
Description. Test elongate, biserial, flattened in side view with truncate edges, and rectangular in cross-section. Elongated chambers and depressed sutures. Terminal aperture with a toothplate on one side. Surface densely ornamented with reticulated costae.
Remarks. The identification of A. pseudothalmanni was based on the original description of Abditodentrix asketocomptella Patterson (1985), which is considered a junior synonym of A. pseudothalmanni by Loeblich and Tappan (1988). According to Hayward et al. (2010), Abditodentrix is distinguished from species belonging to Bolivina by having a rectangular cross-section. Our specimens show good preservation and rectangular cross-section; the tests are small, and the last two chambers tend to be slightly bigger and more inflated compared to the chambers in the early portion.
Paleoecology and paleobathymetric distribution. Abditodentrix is a close relative of Bolivina and most likely shares a similar infaunal lifestyle. This taxon has been reported to occur in high-productivity environments with low-dissolved-oxygen concentrations (Hayward et al., 2002). At several sites located in the waters around New Zealand, it has been documented from middle shelf to abyssal, with peak abundance at bathyal depths (400–1500 m; Hayward et al., 2010).
-
Family Eponididae Hofker 1951
-
Genus Alabaminella Saidova 1975
-
Alabaminella weddellensis (Earland 1936)
Fig. 4: 9a–c
-
1936 Eponides weddellensis Earland: p. 57
-
1975 Alabaminella weddellensis weddellensis (Earland); Saidova, pl. 108, fig. 1
-
1986 Epistominella rotunda (Husezima and Maruhasi); Kurihara and Kennett; pl. 4, figs. 4–8
-
1987 Alabaminella weddellensis (Earland); Loeblich and Tappan, pl. 593, figs. 18–22
-
2001 Alabaminella weddellensis (Earland); Hayward et al., figs. 15U–W
-
2003 Alabaminella weddellensis (Earland); Hayward et al., figs. 4O–Q
-
2010 Alabaminella weddellensis (Earland); Hayward et al., pl. 25, figs. 20–22
-
2015 Alabaminella weddellensis (Earland); Boscolo Galazzo et al., pl. 1, figs. 6–10
Description. Test small, biconvex trochospiral, the spiral side more convex than the umbilical side. Chambers broad and low, five to six in the last whorl. Rounded periphery. The sutures are depressed and radial on the umbilical side and oblique on the spiral side. The test wall is calcareous, smooth, and finely perforated. The aperture is a small oblique interiomarginal and extraumbilical slit.
Remarks. Specimens found in the study material agree with the original description by Earland (1936). The preservation is moderately good, with the fine perforation of the wall and the depressed sutures reported in the literature visible in most of the specimens.
Paleoecology and paleobathymetric distribution. Alabaminella weddellensis has been suggested to have an opportunistic behaviour, and it is included in phytodetritus-exploiting taxa. It is used as a proxy for a high seasonal supply of organic carbon flux linked to seasonal phytoplankton blooms (Gooday, 1993; Smart et al., 1994). Hayward et al. (2010) reported A. weddellensis to be abundant at all depths from middle shelf to abyssal around New Zealand (85–5000 m).
-
Family Anomalinidae Cushman 1927
-
Genus Anomalinoides Brotzen 1942
-
Anomalinoides globulosus (Chapman and Parr 1937)
Fig. 4: 10a–c
-
1884 Anomalina grosserugosa (von Gümbel); Brady, p. 673, pl. 94, figs. 4–5
-
1937 Anomalina globulosa Chapman and Parr, p. 117, pl. 9, fig. 27
-
1953 Anomalina sp.; Phleger, Parker and Pierson, p. 48, pl. 10, figs. 26–28
-
1986 Anomalinoides globulosus (Chapman and Parr); Van Morkhoven et al., p. 36, pl. 9
-
1994 Anomalinoides globulosus (Chapman and Parr); Jones, p. 98, pl. 94, figs. 4–5
-
2013 Anomalinoides globulosus (Chapman and Parr); Holbourn et al., p. 52, figs. 1–3
Description. Test large, low trochospiral, inflated, with rounded periphery. The spiral side is moderately evolute and slightly convex, and the umbilical side is more convex and involute. The sutures are depressed and curved. The wall is calcareous and perforated, with larger pores on the spiral side. Aperture peripheral with a low equatorial arch bordered by a thick lip.
Remarks. At Site U1506, specimens are typically broken, missing the last chambers; the perforations are commonly well-preserved, with pores visible on both sides. In this study, we identified two morphotypes of A. globulosus: morphotype A perfectly fits the original description of A. globulosus; morphotype B is a flatter morphotype of A. globulosus. Most specimens of A. globlosus identified at Site U1506 belong to morphotype B, they are flatter than the type material, they have more chambers in the final whorl, and the last two chambers are moderately inflated. The pores are large on both sides; the sutures on the dorsal side are limbate, apart from the ones in the last chambers, which are flush to slightly depressed. Boersma (1984b) noted a flatter form of Anomalinoides globulosus in the south-western Pacific at intermediate depth (< 1000 m) that closely resembles the morphology depicted as Anomalina grosserugosa in Brady (1884) and agrees with our A. globlosus morphotype B.
Paleoecology and paleobathymetric distribution. This cosmopolitan species has been documented at middle bathyal to abyssal depths (Pflum and Frerichs, 1976; Van Morkhoven et al., 1986). Boersma (1984b) observed a flatter morphotype of A. globulosus at intermediate depths (ca. 1000 m) in the south-western Pacific.
-
Family Bolivinidae Glaessner 1937
-
Genus Bolivina d'Orbigny 1839
-
Bolivina finlayi Hornibrook 1961
Fig. 4: 11a–c
-
1961 Bolivina finlayi Hornibrook, pl. 9, figs. 169–171
-
1979 Bolivina finlayi Hornibrook; Hayward and Buzas, pl. 6, fig. 67
-
1989 Bolivinita finlayi Kennett; Hornibrook et al., p. 159, fig. 38
-
2004 Bolivina finlayi Hornibrook; Hayward, fig. 2P
Description. Test elongate, ovoid to triangular in outline, compressed. Chambers numerous (18–20), elongated, biserial, slightly inflated; suture slightly depressed. The early portion of the test presents numerous longitudinal striae up to and beyond the half length of the test, finely perforated; the final chamber is smooth and sparsely perforated. Slit-like aperture.
Remarks. The specimens found at Site U1506 are well preserved. The fine longitudinal striae, which extend across approximately half the length of the test, are difficult to observe under a microscope but are visible in the SEM images (Fig. 7: 1). This species is similar to Bolivina punctatostriata (Kreuzberg, 1930), but our specimens lack the typical strongly curved sutures of B. punctatostriata. Moreover, B. punctatostriata is restricted to shelf depths around New Zealand (Hayward and Buzas, 1979). This species is also similar to Bolivina striatula (Cushman, 1922), but B. striatula present striae only in the lower half of the test and are highly perforated.
-
Family Buliminidae Jones 1875
-
Genus Bulimina d'Orbigny 1826
-
Bulimina cf. marginata d'Orbigny 1826
Fig. 4: 12a–c
-
1826 Bulimina marginata d'Orbigny: p. 269, n. 4, pl. 12, figs. 10–12
-
1999 Bulimina marginata f. marginata d'Orbigny; Hayward et al., pl. 9, fig. 5.
-
2008 Bulimina marginata d'Orbigny; Kender et al., p. 553, pl. 17, figs. 10–11
-
2010 Bulimina marginata f. marginata d'Orbigny; Hayward et al., pl. 17, figs. 23–24
Description. Test triserial and elongate, conical in outline and rounded in cross-section. Chambers rapidly increase in size and are larger and inflated close to the apertural end. The chamber margins are fringed with typical short spines. The sutures are distinct and depressed. The wall is calcareous and smooth. A short spine is often observed in the apical end. Loop-shaped aperture, with a lip.
Remarks. The holotype of B. marginata was not available at the USNM, but we examined the holotypes of B. marginata grandissima Martin (1952) (USNM 237467) and Bulimina marginata tessellata Cushman and Todd (1945) (USNM CC 44419), and both varieties show clear spines at the base of the chambers. Bulimina marginata is known for its high variability, with three forms documented in its type area, the Adriatic Sea, by Jorissen (1988) and two additional forms recognised in New Zealand by Hayward et al. (2010). Our specimens closely resemble the description of B. marginata marginata (Jorissen, 1988), characterised by an elongated test and undercut margins with short spines. The preservation of B. marginata is generally good at Site U1506.
Paleoecology and paleobathymetric distribution. Bulimina marginata is considered to be a cosmopolitan species (Murray, 1991). Around New Zealand this taxon occurs with the highest abundance at shelf and bathyal depths (0–2000 m; Hayward et al., 2010). Along with other Bulimina species, B. marginata is associated with low-oxygen conditions at the seafloor (Fontanier et al., 2002).
-
Family Buliminidae Jones 1875
-
Genus Bulimina d'Orbigny 1826
-
Bulimina striata d'Orbigny 1843
Fig. 4: 13a–c
-
1826 Bulimina costata d'Orbigny; pl. 18, fig. 1 (nomen nudum)
-
1843 Bulimina striata d'Orbigny in Guérinméneville, p. 9, pl. 2, fig. 16
-
1852 Bulimina costata d'Orbigny; d'Orbigny, p. 194
-
1986 Bulimina mexicana Cushman; Van Morkhoven et al., p. 61, pl. 19, figs. 1–4
-
1986 Bulimina striata d'Orbigny; Kurihara and Kennett, pl. 2, fig. 13
-
1994 Bulimina striata d'Orbigny; Loeblich and Tappan, pl. 242, figs. 8–14
-
2005 Bulimina striata d'Orbigny; Vénec-Peyre, p. 157, pl. 18, fig. 1
-
2010 Bulimina striata d'Orbigny; Hayward et al., pl. 17, figs. 25–27
-
2019 Bulimina striata d'Orbigny; Ridha et al., fig. 3: 13
Description. Test elongate, triserial, conical in side view. Rounded apertural end, acute apical end, subcircular in cross-section. Chambers are inflated and increase rapidly in size, separated by depressed sutures. Wall calcareous, ornamented with longitudinal costae that extend from the external margin and create short spines. The aperture is loop-shaped and extends up from the base of the final chamber; a lip merged with an internal toothplate is present and borders the aperture (Fig. 7: 2).
Remarks. In our material, the costae are not long and end as short spines at the margin of the chamber. The best-preserved specimens present a short spine in the apical end.
Paleoecology and paleobathymetric distribution. Bulimina striata is a shallow infaunal species interpreted as an indicator of suboxic conditions (Kaiho, 1994), and it has been linked to high-productivity conditions (Russo et al., 2007). In the Gulf of Mexico, it is dominant in the upper part of the middle bathyal zone (Bandy, 1961). Around New Zealand, B. striata occurs mostly outer shelf to the upper abyssal, with its greatest abundance between 200–2000 m (Hayward et al., 2010).
-
Family Buliminidae Jones 1875
-
Genus Bulimina d'Orbigny 1826
-
Bulimina truncana von Gümbel 1868
Fig. 4: 14a–c
-
1868 Bulimina truncana von Gümbel; p. 644, pl. 2, figs. 77a–b
-
1884 Bulimina rostrata Brady; p. 408, pl. 51, figs. 14–15
-
1979 Bulimina truncana von Gümbel; Hayward and Buzas p. 45, pl. 7, figs. 87–88
-
1986 Bulimina rostrata Brady; Kurihara and Kennett, pl. 2, figs. 12–13
-
1991 Bulimina alazanensis Cushman: Schroder-Adams, p. 624, pl. 1, fig. 5
-
1999 Bulimina truncana von Gümbel; Kawagata, fig. 5–7
-
2001 Bulimina truncana von Gümbel; Hayward et al., figs. 14DD–EE
-
2010 Bulimina truncana von Gümbel; Hayward et al., pl. 17, figs. 28–29
-
2019 Bulimina truncana von Gümbel; Ridha et al., fig. 3: 10a–b
Description. Test triserial, elongate, sub-triangular in cross-section. The apertural end is rounded and pointed in the apical end. The test has the greatest width near the apertural end. The chambers are concealed by surface ornamentations. Wall calcareous, finely perforated, with marked longitudinal costae occurring almost throughout the test. The final chambers are smooth. The costae form a spinose protuberance in the initial portion. Loop-shaped aperture with an internal tooth plate.
Remarks. Our specimens agree with the original description of von Gümbel (1868) and with the description of Hayward et al. (2010). At Site U1506, B. truncana presents typically elongate tests with distinct continuous costae; the aperture is loop-shaped; and, in the specimens with good preservation, the internal tooth plate can be observed (Fig. 7: 3a–b).
Paleoecology and paleobathymetric distribution. This cosmopolitan species (von Gümbel, 1868) has been documented around New Zealand from upper bathyal to abyssal depths, with the highest abundance at middle to lower bathyal depths (700–2000 m; Hayward et al., 2010).
-
Family Cibicididae Cushman 1927
-
Genus Cibicidoides Thalmann 1939
-
Cibicidoides bradyi (Trauth 1918)
Fig. 4: 15a–c
-
1918 Truncatulina bradyi Trauth, p. 235
-
1976 Cibicidoides bradyi (Trauth); Pflum and Frerichs, pl. 3, figs. 6–7
-
1989 Cibicidoides bradyi (Trauth); Hermelin, pl. 17 figs. 2–4
-
1994 Gyroidina bradyi (Trauth); Jones, p. 99, pl. 95, fig. 5
-
1999 Cibicidoides bradyi (Trauth); Kawagata, fig. 7.1
-
2010 Cibicides bradyi (Trauth); Hayward et al., pl. 22, figs. 1–3
-
2013 Cibicidoides bradyi (Trauth); Holbourn et al., p. 162, figs. 1–6
-
2019 Cibicidoides bradyi (Trauth); Ridha et al., fig. 8: 1a–b
Description. Test trochospiral, unequally biconvex, periphery rounded without a keel. Umbilical side involute, spiral side evolute. Chambers inflated, six to eight per whorl. Wall calcareous, coarsely perforated on the spiral side, finely perforated on the umbilical side. Sutures are radial on the umbilical side and oblique to slightly curved on the spiral side. The aperture is a small slit that extends from the periphery to the spiral side.
Remarks. At Site U1506, specimens of C. bradyi show good preservation, and it is possible to observe both the oblique sutures and the pores on the spiral side. Compared to other Cibicidoides species (e.g. Cibicidoides mundulus or Cibicidoides mexicanus), it is characterised by a smaller test and fewer chambers per whorl. Commonly, the umbilicus is slightly open, and on rare occasions a small umbilical plug is present.
Paleoecology and paleobathymetric distribution. Cibicidoides bradyi has been related to well-oxygenated bottom waters (Denne and Sen Gupta, 1991; Barmawidjaja et al., 1992). Around New Zealand, C. bradyi occurs mostly from middle bathyal to abyssal depths (800–4000 m; Hayward et al., 2010). Egger (1893) documented C. bradyi from outer shelf to abyssal water depths (100–4000 m).
-
Family Cibicididae Cushman 1927
-
Genus Cibicidoides Thalmann 1939
-
Cibicidoides mundulus (Brady et al., 1888)
Fig. 4: 16a–c
-
1888 Truncatulina mundula Brady, Parker and Jones; p. 228, pl. 45, figs. 25a–c
-
1953 Cibicides kullenbergi Parker; Phleger, Parker and Pierson, p. 49, pl. 11, figs. 7–8
-
1955 Cibicidoides mundulus (Brady, Parker and Jones); Loeblich and Tappan, p. 25, figs. 4a–c
-
1986 Cibicidoides mundulus (Brady, Parker and Jones); Van Morkhoven et al., pl. 21
-
1989 Cibicidoides mundulus (Brady, Parker and Jones); Hermelin, pl. 17, figs. 9–11
-
2013 Cibicidoides mundulus (Brady, Parker and Jones); Holbourn et al., p. 196, figs. 1–8
-
2019 Cibicidoides mundulus (Brady, Parker and Jones); Ridha et al., fig. 8: 2a–c
Description. Test trochospiral, keeled, biumbonate, unequally biconvex with the umbilical side more convex; 10 to 12 chambers in the last whorl, low and inflated, separated by flush curved sutures. Wall calcareous with fine pores on the umbilical side and coarser pores on the spiral side. The aperture is an equatorial slit that extends on the spiral side, rounded by a lip.
Remarks. The specimens of C. mundulus found at Site U1506 match the original description by Brady, Parker, and Jones in 1888, as well as the descriptions successively given by Van Morkhoven et al. (1986) and Holbourn et al. (2013). Our specimens display a medium to large test size and are moderately well preserved.
Paleoecology and paleobathymetric distribution. The bathymetric distribution of C. mundulus ranges from bathyal to abyssal depths (Van Morkhoven et al., 1986). This species has been associated with oligotrophic and oxic environments at the seafloor (Loubere 1994; Fariduddin and Loubere 1997; Schmiedl et al., 1997; De Rijk et al., 2000).
-
Family Cibicididae Cushman 1927
-
Genus Cibicidoides Thalmann 1939
-
Cibicidoides wuellerstorfi (Schwager 1866)
Fig. 5: 1a–c
-
1866 Anomalina wuellerstorfi Schwager, p. 258, pl. 7, figs. 105, 107
-
1884 Truncatulina wuellerstorfi (Schwager); Brady, pl. 93, figs. 8–9
-
1979 Cibicides wuellerstorfi (Schwager); Hayward and Buzas, pl. 11, figs. 140–142
-
1986 Planulina wuellestorfi (Schwager); Van Morkhoven et al., pl. 14
-
1989 Planulina wuellestorfi (Schwager); Hornibrook et al., fig. 22, n. 7
-
2010 Cibicides wuellerstorfi (Schwager); Hayward et al.,pl. 23 figs. 17–22
-
2013 Planulina wuellestorfi (Schwager); Holbourn et al., p. 416, figs. 1–3
-
2019 Cibicidoides wuellerstorfi (Schwager): Ridha et al., fig. 8: 3a–c
Description. Test compressed, discoidal, and low trochospiral. In cross-section, the test is compressed planoconvex, with a flat spiral side and an evolute, more convex umbilical side. Carinate periphery is acute; 8 to 10 chambers in the final whorl, narrow and curved, separated by thick and curved sutures. Sutures limbate, curved on the spiral side and more sinusoid on the umbilical side; slightly depressed between the last chambers. Medium-sized umbo on the umbilical side. Wall calcareous with coarse pores on the spiral side and finer pores on the umbilical side. The aperture is an equational interiomarginal slit with a lip extending on both sides.
Remarks. Specimens at Site U1506 show fairly good preservation and have overall large tests, with thick and elevated sutures and coarse pores with a rounded keel on the spiral side. The tests show variability in the level of compression; some specimens present a distinctly convex umbilical side.
Paleoecology and paleobathymetric distribution. This epifaunal species has been associated with high-energy environments, well-oxygenated bottom waters, and intermediate to high temperatures (Lutze and Thiel, 1989; Linke and Lutze, 1993; Mackensen et al., 1995). Hayward et al. (2010) reported C. wuellerstorfi to be abundant from upper bathyal to abyssal depths (400–3000 m) around New Zealand.
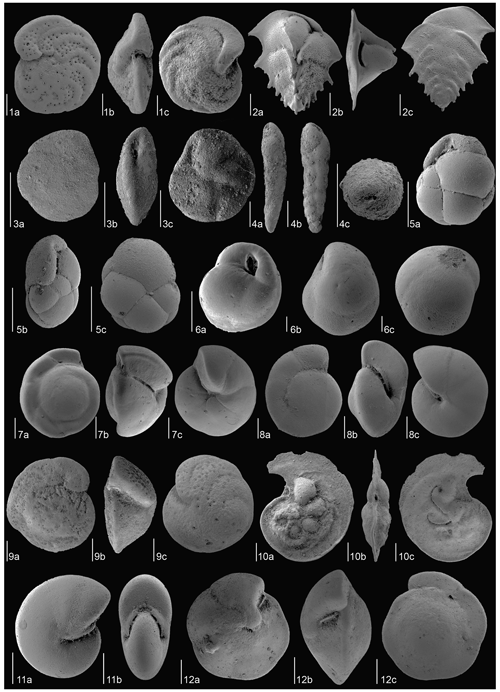
Figure 5SEM images of the most common benthic foraminiferal species at Site U1506 identified in the Late Miocene–Early Pliocene. (1) Cibicidoides wuellestorfi (sample U1506A-11R-1W, 105–107 cm), MCNUZ-MPZ-2023/350: (a) dorsal side, (b), side view, (c) ventral side. (2) Ehrenbergina carinata (sample U1506A-19R-4W, 97–99 cm), MCNUZ-MPZ-2023/351: (a) frontal side, (b) apertural view, (c) dorsal side. (3) Epistominella exigua (sample U1506A-11R-4W, 80–82 cm), MCNUZ-MPZ-2023/352: (a) dorsal side, (b) apertural view, (c) ventral side. (4) Fursenkoina bradyi (sample U1506A-21R-1W, 140–142 cm), MCNUZ-MPZ-2023/344: (a) frontal view, (b) side view, (c) outline view. (5) Globocassidulina crassa (sample U1506A-11R-1W, 105–107 cm), MCNUZ-MPZ-2023/353: (a) apertural view, (b) side view, (c) dorsal side. (6) Globocassidulina subglobosa (sample U1506A-12R-1W, 110–112 cm), MCNUZ-MPZ-2023/354: (a) apertural view. (6) Globocassidulina subglobosa (sample U1506A-11R-1W, 105–107 cm), MCNUZ-MPZ-2023/355: (b) side view, (c) dorsal side. (7) Gyroidina orbicularis (sample U1506A-20R-1W, 50–52 cm), MCNUZ-MPZ-2023/356: (a) dorsal side, (b) side view, (c) ventral side. (8) Gyroidina soldanii (sample U1506A-21R-2W, 50–52 cm), MCNUZ-MPZ-2023/357: (a) dorsal side, (b) side view, (c) ventral side. (9) Heterolepa dutemplei (sample U1506A-21R-1W, 140–142 cm), MCNUZ-MPZ-2023/358: (a) dorsal side, (b) side view, (c) ventral side. (10) Laticarinina pauperata (sample U1506A-11R-1W, 105–107 cm), MCNUZ-MPZ-2023/359: (a) dorsal side, (b), side view, (c) ventral side. (11) Melonis barleeanum (sample U1506A-20R-1W, 50–52 cm), MCNUZ-MPZ-2023/360: (a) side view, (b) apertural view. (12) Oridorsalis umbonatus (sample U1506A-18R-4W, 50–52 cm), MCNUZ-MPZ-2023/361: (a) dorsal side, (b), side view, (c) ventral side. All scale bars = 100 µm.
-
Family Ehrenberginidae Cushman 1927
-
Genus Ehrenbergina Reuss 1850
-
Ehrenbergina carinata Eade 1967
Fig. 5: 2a–c
-
1967 Ehrenbergina carinata Eade, p. 448, pl. 9, figs. 1–4
-
1983 Ehrenbergina carinata Eade; Nomura, pl. 5, figs. 5–6
-
2010 Ehrenbergina carinata Eade; Hayward et al., pl. 18, figs. 9–11
-
2019 Ehrenbergina carinata Eade; Ridha et al., fig. 4: 4a–b
Description. Test triangular in both cross-section and dorsal view. The ventral side is raised toward the central keel; the dorsal side is flat and slightly concave. Chambers biserially arranged, slightly inflated in the adult portion. The sutures are depressed on the ventral side and slightly raised on the dorsal side. Wall calcareous and finely perforated on both sides. Well-developed peripheral keel, with large spines extending from each chamber. The apertural face is flat without pores. The aperture is an elongated slit that extends from the margin with the previous chamber into the apertural face, bordered by a narrow lip that partially covers the aperture.
Remarks. Two paratypes of Ehrenbergina carinata were examined at the GNS collection in Wellington (TF 1561), and they are consistent with our material. The larger specimens of E. carinata at Site U1506 are generally well preserved, with a developed keel and evident spines protruding from it. We identified this species as E. carinata based on the keel, the spines, and the smooth dorsal side, which differentiate it from Ehrenbergina aspinosa, characterised by smaller spines and more raised sutures on the dorsal side.
Paleoecology and paleobathymetric distribution. This shallow infaunal species has been associated with low-oxygen conditions and high nutrient levels (Nomura et al., 1991; Gupta et al., 2006, 2008). Around New Zealand, E. carinata has been documented at bathyal depths (400–2000 m; Hayward et al., 2010).
-
Family Pseudoparrellidae Voloshinova 1952
-
Genus Epistominella Husezima and Maruhasi 1944
-
Epistominella exigua (Brady 1884)
Fig. 5: 3a–c
-
1884 Pulvinulina exigua Brady, p. 696, pl. 103, figs. 13–14
-
1986 Epistominella exigua (Brady); Kurihara and Kennett, pl. 3, figs. 10–12
-
1994 Pseudoparrella exigua (Brady); Loeblich and Tappan, pl. 307, figs. 1–7
-
2010 Epistominella exigua (Brady); Hayward et al., pl. 31, figs. 6–10
-
2013 Epistominella exigua (Brady); Holbourn et al., p. 340, figs. 1–6
-
2015 Epistominella exigua (Brady); Boscolo Galazzo, pl. 1, figs. 11–13
Description. Test small to medium in size, low trochospiral and biconvex, compressed, with an acute periphery. Around five slightly inflated chambers for each of the three whorls. The sutures are oblique and straight on the dorsal side and radial, depressed, and slightly curved on the umbilical side. Wall calcareous, typically glassy and translucent. The aperture is an elongated interiomarginal slit, parallel to the peripheral margin, extending from the face of the last chamber onto the umbilical side.
Remarks. Our specimens agree with the original description by Brady (1884). Due to recrystallisation, our specimens do not present the typical glassy and translucent wall. Only well-preserved specimens show the fine perforations described by Brady (1884) in the original description and illustrated by Holbourn et al. (2013). The radial and curved sutures on the umbilical side and the aperture have been used as the main morphological features to recognise this species, being observable in most of the specimens.
Paleoecology and paleobathymetric distribution. In the Gulf of California, E. exigua has been found in the upper–middle bathyal zone (Bandy, 1961), while around New Zealand, the highest abundance has been documented at lower bathyal to abyssal depths (1000–5000 m; Hayward et al., 2010). Epistominella exigua is considered a phytodetritus-exploiting taxon, having opportunistic behaviour and showing high abundance related to phytoplankton blooms. This species, along with other phytodetritus-exploiting taxa, is used as a proxy for highly seasonal nutrient supply (Gooday, 1988, 1993; Jorissen et al., 2007; Corliss et al., 2009).
-
Family Bolivinitidae Cushman, 1927
-
Genus Fursenkoina Loeblich and Tappan, 1961
-
Fursenkoina bradyi (Cushman, 1922)
Fig. 5: 4a–c
-
1922 Virgulina bradyi Cushman, p. 155, pl. 24, figs. 1
-
1960 Virgulina bradyi Cushman; Barker, pl. 52, fig. 9
-
1985 Fursenkoina bradyi (Cushman); Thomas, pl. 3, fig. 7
-
1994 Fursenkoina bradyi (Cushman); Jones, pl. 52, fig. 9
Description. Test elongate, twisted, fusiform, with the maximum width in the final whorl, circular in cross-section. Chambers biserially arranged; moderately rounded; not inflated; and separated by distinct, curved sutures. The wall is calcareous and smooth. Elongate and oval aperture, extending from the base of the last chamber.
Remarks. The specimens ascribed to F. bradyi at Site U1506 mostly agree with the original description by Cushman (1922) and with the holotype (USNM PP 16287 A). However, the chambers of the specimens observed at Site U1506 are not inflated. Due to partial recrystallisation and the presence of particularly delicate elements, certain distinctive morphological characteristics, such as the fine perforation of the wall and the toothplate in the aperture, are not always visible or present in our specimens.
Paleoecology and paleobathymetric distribution. This cosmopolitan species typically occurs at upper bathyal depths (Jones, 1994). Kaiho (1994) assigned the genus Fursenkoina to the dysoxic indicators.
-
Family Cassidulinidae d'Orbigny 1839
-
Genus Globocassidulina Voloshinova 1960
-
Globocassidulina crassa (d'Orbigny 1839)
Fig. 5: 5a–c
-
1839 Cassidulina crassa d'Orbigny, p. 56, pl. 7, figs. 18–20
-
1967 Globocassidulina crassa (d'Orbigny); Eade, fig. 4
-
1983 Globocassidulina crassa (d'Orbigny); Nomura, p. 37–40, text figs 31–32, pl. 3, figs. 9a–c, 10a–b
-
2001 Globocassidulina crassa (d'Orbigny); Hayward et al., figs. 14MM–NN
-
2010 Globocassidulina crassa (d'Orbigny); Hayward et al., pl. 19, figs. 19–21
Description. Test subcircular, oval to circular in outline, compressed in profile, and with a rounded periphery. Chambers are biserially arranged with a planispiral coil. The sutures are distinct and slightly depressed. Wall calcareous, smooth, and finely perforated. The primary aperture is a narrow slit extending up the apertural face at an angle, without apertural toothplates.
Remarks. Our specimens are characterised by generally small tests; no large tests have been found at Site U1506. Previously denominated as Cassidulina crassa (i.e. Sejrup and Guilbault, 1980; Murray, 1991; Suhr and Pond, 2006), we attributed this species to the genus Globocassidulina following the description of Loeblich and Tappan (1988). According to these authors, the main difference between the two genera is the aperture, slit-like and extending up the apertural face in Globocassidulina, and a narrow slit at the base of the apertural face and parallel to the peripheral margin in Cassidulina. Our specimens present an aperture typical of the genus Globocassidulina (Fig. 7: 4).
Paleoecology and paleobathymetric distribution. Globocassidulina crassa is a cold-water species, related to strong bottom currents (Schmiedl et al., 1997). This species has been associated with phytoplankton blooms and is considered to be a phytodetritus-exploiting taxon (Suhr and Pond, 2006). Hayward et al. (2010) documented this species around New Zealand from middle shelf to abyssal depths.
-
Family Cassidulinidae d'Orbigny 1839
-
Genus Globocassidulina Voloshinova 1960
-
Globocassidulina subglobosa (Brady 1881)
Fig. 5: 6a–c
-
1881 Cassidulina subglobosa Brady, p. 60
-
1884 Cassidulina subglobosa Brady, pl. 54, figs. 17a–c
-
1951 Cassidulina subglobosa Brady; Phleger and Parker, p. 27, pl. 14, figs. 11–12
-
1983 Globocassidulina subglobosa (Brady); Nomura, fig. 16, pl. 2, figs. 8a–c, pl. 13, figs. 5–6
-
1986 Globocassidulina subglobosa (Brady); Kurihara and Kennett; pl. 5, figs. 4–8
-
2001 Globocassidulina subglobosa (Brady); Hayward et al., fig. 14QQ
-
2003 Globocassidulina subglobosa (Brady); Hayward et al., fig. 4J
-
2010 Globocassidulina subglobosa (Brady); Hayward et al., pl. 20, figs. 4–6
-
2013 Globocassidulina subglobosa (Brady); Holbourn et al., p. 264, figs. 1–2
-
2019 Globocassidulina subglobosa (Brady); Ridha et al., fig. 4: 2
Description. Test subglobular, chambers biserially arranged with a rounded periphery, and subcircular in cross-section. The size is highly variable. Chambers inflated, separated by slightly depressed sutures. The wall is calcareous, and the surface is smooth and finely perforated. The primary aperture is a straight loop-like slit extending up the apertural face, with an apertural toothplate on the posterior side.
Remarks. Specimens of G. subglobosa at Site U1506 agree with the original description by Brady (1881). Most of our specimens have a small test, and larger specimens have been found occasionally, particularly between 194.75 and 103.21 m CSF-A. The good preservation allows for the recognition of the diagnostic morphological features, and SEM images show the apertural toothplate in the primary aperture (Fig. 7: 5).
Paleoecology and paleobathymetric distribution. This species is interpreted as an indicator of oxic conditions, strong bottom currents, and oligotrophic environments with low pulsed food supply (Schmiedl et al., 1997; Kaiho, 1999). This opportunistic species has been associated with high phytodetritus pulses to the seafloor and therefore is considered a phytodetritus-exploiting taxon (Gooday, 1993; Suhr et al., 2003; Jorrisen et al., 2007). Globocassidulina subglobosa shows high abundance at lower bathyal to abyssal depths around New Zealand (Hayward et al., 2010). In the Gulf of California and the Gulf of Mexico, it has been documented mainly at upper to middle bathyal depths (Bandy, 1961; Pflum and Frerichs, 1976).
-
Family Cancrisidae Chapman, Parr and Collins 1934
-
Genus Gyroidinoides Brotzen 1942
-
Gyroidina orbicularis d'Orbigny 1826
Fig. 5: 7a–c
-
1826 Gyroidina orbicularis d'Orbigny, p. 278
-
1979 Gyroidina sp.; Hayward and Buzas, pl. 18, figs. 226–228
-
1989 Gyroidina orbicularis d'Orbigny; Hermelin, pl. 16, figs. 7–9
-
1987 Gyroidina orbicularis d'Orbigny; Loeblich and Tappan, pl. 716, figs. 8–13
-
1989 Gyroidina zelandica Finlay; Hornibrook et al., fig. 20, n. 11a–b
-
1994 Gyroidina orbicularis d'Orbigny; Jones, pl. 115, fig. 6;
-
2010 Gyroidina orbicularis d'Orbigny; Hayward et al., pl. 27, figs. 1–6
Description. Test moderately large to large in size, planoconvex, flat on the spiral side, and highly convex on the umbilical side. The periphery is angular with a rounded keel. Around seven to nine chambers in the last whorl. The sutures are oblique on the spiral side and depressed, radial to slightly curved on the umbilical side. Wall calcareous and smooth. The aperture is an interiomarginal slit not connected to the umbilicus.
Remarks. At Site U1506, specimens of G. orbicularis have large tests and are fairly well preserved. The species Gyroidina zelandica Finlay (1939) illustrated by Hornibrook et al. (1989) is characterised by a flat spiral side and conical dorsal side, eight chambers per coil (maximum nine chambers), a small umbilicus, and an angular periphery. Based on the comparison of the original description of both species, we consider G. zelandica to be a synonym of G. orbicularis.
Paleoecology and paleobathymetric distribution. Around New Zealand, this species has been documented from bathyal to abyssal depths (200–5000 m; Hayward et al., 2010).
-
Family Cancrisidae Chapman, Parr and Collins 1934
-
Genus Gyroidinoides Brotzen 1942
-
Gyroidina soldanii d'Orbigny 1826
Fig. 5: 8a–c
-
1826 Gyroidina soldanii d'Orbigny, p. 278
-
1846 Gyroidina soldanii d'Orbigny; d'Orbigny, pl. 8, figs. 10–12
-
1978 Gyroidina soldanii d'Orbigny; Wright, p. 715, pl. 5, figs. 7–9
-
1994 Gyroidina neosoldanii Brotzen; Loeblich and Tappan, pl. 361, figs. 13–15; pl. 362, figs. 1–7
-
2001 Gyroidinoides soldanii (d'Orbigny); Hayward et al., figs. 15HH–II
-
2010 Gyroidina soldanii d'Orbigny; Hayward et al., pl. 27, figs. 7–12
-
2013 Gyroidinoides soldanii (d'Orbigny); Holbourn et al., p. 278, figs. 1–3
-
2019 Gyroidinoides soldanii (d'Orbigny); Ridha et al., fig. 6: 3a–c
Description. Test planoconvex, trochospiral, with a rounded to angular periphery. The spiral side is evolute and flat, and the umbilical side is involute and more rounded; 8 to 10 chambers in the last whorl, wall calcareous and smooth, with fine perforations. The sutures are radial and depressed, straight on the umbilical side, and more curved on the spiral side. The aperture is an extraumbilical interiomarginal slit.
Remarks. This species has been identified following the original description by d'Orbigny (1826) and the more recent one by Hayward et al. (2010). Specimens at Site U1506 show good preservation, the test size ranges from medium to large, and some juvenile specimens have been observed occasionally. In the larger and better-preserved specimens, it is possible to observe the fine perforations of the test.
Paleoecology and paleobathymetric distribution. This cosmopolitan species is commonly found at bathyal depths (Holbourn et al., 2013). In New Zealand, it occurs from outer shelf to lower abyssal depths (100–5000 m; Hayward et al., 2010).
-
Family Cibicididae Cushman 1927
-
Genus Heterolepa Franzenau, 1884
-
Heterolepa dutemplei (d'Orbigny 1846)
Fig. 5: 9a–c
-
1846 Rotalina dutemplei d'Orbigny, p. 157, pl. 8, figs. 19–21
-
1986 Cibicidoides dutemplei (d'Orbigny); Van Morkhoven et al., p. 112, pl. 35.
-
1987 Heterolepa dutemplei (d'Orbigny); Loeblich and Tappan, p. 632, pl. 709, figs. 1–8
-
1996 Heterolepa dutemplei (d'Orbigny); Revets, p. 67, pl. 2, figs. 9–12.
-
2013 Heterolepa dutemplei (d'Orbigny); Holbourn et al., p. 294, figs. 1–3
Description. Test trochospiral, planoconvex in cross-section with a flat spiral side and a convex umbilical side; 9 to 10 chambers in the last whorl, inflated and separated by curved and slightly depressed sutures. The sutures on the umbilical side form a pinwheel pattern. Wall calcareous with pores on both sides of the test. The aperture is an equatorial slit, extending onto the spiral side, surrounded by a thin lip.
Remarks. Specimens found at Site U1506 were ascribed to H. dutemplei following the description of Van Morkhoven et al. (1986) and Holbourn et al. (2013). Our specimens are large and well preserved. In some specimens, the early whorl on the spiral side is covered by a thick and crystalline area, which is a typical feature of Cibicidoides mexicanus (Nuttall, 1932). Heterolepa dutemplei bears a striking resemblance to Cibicidoides mexicanus (Nuttall), suggesting a possible evolutionary connection between these two species (Van Morkhoven et al., 1986). Our specimens show the pinwheel pattern on the umbilical side, which is a distinctive feature of H. dutemplei. Furthermore, C. mexicanus is known to have a stratigraphic range that extends from the late Eocene to the Early Miocene, whereas H. dutemplei has been identified from the Early Miocene through the Pliocene.
Paleoecology and paleobathymetric distribution. Heterolepa dutemplei spans from shelf to upper bathyal water depths (Van Morkhoven et al., 1986). The presence of this epifaunal species has been linked to environments characterised by high oxygen levels and low salinity (Hageman, 1979; Van der Zwaan, 1982; Jonkers, 1984).
-
Family Discorbinellidae Sigal 1952
-
Genus Laticarinina Galloway and Wissler 1927
-
Laticarinina pauperata (Parker and Jones 1865)
Fig. 5: 10a–c
-
1865 Pulvinulina repanda Fichtell and Moll var. menardii d'Orbigny, subvar. pauperata Parker and Jones, p. 395, pl. 16, figs. 50–51
-
1884 Pulvinulina pauperata (Parker and Jones); Brady, p. 696, pl. 104, figs. 3–11
-
1986 Laticarinina pauperata (Parker and Jones); Van Morkhoven et al., p. 89, pl. 26
-
1942 Laticarinina bulbrooki Cushman and Todd; Cushman and Todd, p. 19, pl. 4, figs. 8-9
-
1942 Laticarinina crassicarinata Cushman and Todd; Cushman and Todd, p. 18, pl. 4, figs. 11–12
-
1987 Laticarinina pauperata (Parker and Jones); Loeblich and Tappan, pl. 631, figs. 1–4
-
1992 Laticarinina pauperata (Parker and Jones); Mackensen, p. 668, pl. 2, figs. 1–2
-
2010 Laticarinina pauperata (Parker and Jones); Hayward et al., pl. 24, figs. 19–21
-
2013 Laticarinina pauperata (Parker and Jones); Holbourn et al., p. 328, figs. 1–2
-
2019 Laticarinina pauperata (Parker and Jones); Ridha et al., fig. 7: 3a–b
Description. Test large, elliptical in outline, strongly compressed in profile, with a wide and transparent keel. Planoconvex to unequally biconvex. Both sides are evolute, the spiral side is flat to slightly convex, and the umbilical side is raised. Six to eight inflated chambers in the last whorl. Sutures are radial and depressed on the umbilical side and flush, barely distinguishable on the spiral side. Wall calcareous and smooth. The aperture consists of a subequatorial slit.
Remarks. Our specimens show large and compressed tests with a wide and transparent keel. We distinguished this species from Laticarinina altocamerata, based on the more compressed test and the less raised umbilical side. Most of the specimens observed at Site U1506 have a broken keel.
Paleoecology and paleobathymetric distribution. Kaiho (1994) related this species to oxic conditions on the seafloor. Hayward et al. (2010) documented L. pauperata from lower bathyal to abyssal depths (1500–3000 m) around New Zealand.
-
Family Nonionidae Schultze 1854
-
Genus Melonis Montfort 1808
-
Melonis barleeanum (Williamson 1858)
Fig. 5: 11a–b
-
1858 Noniona barleeana Williamson, p. 32, pl. 3, figs. 68–69
-
1985 Melonis barleeanus (Williamson); Thomas, p. 677, pl. 12, fig. 3
-
1986 Melonis barleeanum (Williamson); Kurihara and Kennett, pl. 9, figs. 10–11
-
1987 Melonis barleeanum (Williamson); Loeblich and Tappan, pl. 696, figs. 5–6
-
1989 Melonis barleeanum (Williamson); Hermelin, p. 88, pl. 17, fig. 12
-
1994 Melonis barleeanus (Williamson); Loeblich and Tappan, p. 159, pl. 347, figs. 1–5
-
2013 Melonis barleeanum (Williamson); Holbourn et al., p. 354, figs. 1–2
-
2019 Melonis barleeanus (Williamson); Ridha et al., fig. 7: 6a–b
Description. Test planispiral, involute, laterally compressed, and symmetrical. Open umbilicus on both sides, 10 to 12 chambers, gradually increasing in size in the final whorl. The chambers are separated by slightly depressed sutures, slightly curved. Wall calcareous, coarsely perforated. The aperture is a low interiomarginal, equatorial slit that extends to both umbilici.
Remarks. We were able to distinguish M. barleeanus from Melonis pompilioides based on the flatter test shape of M. barleeanus, which generally has more chambers in the last whorl. Our specimens bear a close morphological resemblance to Melonis affinis (Reuss), apart from the prominent apertural lip, which allows us to identify them as M. barleeanum (Milker and Schmiedl, 2012). Moreover, the type levels of the two species are very different: Oligocene for M. affinis and recent for M. barleeanum (Holbourn et al., 2013). Our specimens are all well preserved and characterised by small tests with visible pores.
Paleoecology and paleobathymetric distribution. Around New Zealand, this species has been documented from the outer shelf to abyssal depths (Hayward et al., 2010), with no depth preference. Melonis has been related to suboxic and eutrophic conditions on the seafloor (Kaiho, 1994; Hayward et al., 2010).
-
Family Orispiralidae Loeblich and Tappan 1984
-
Genus Oridorsalis Andersen 1961
-
Oridorsalis umbonatus (Reuss 1851)
Fig. 5: 12a–c
-
1851 Rotalina umbonata Reuss, p. 75, pl. 5, fig. 35
-
1983 Oridorsalis umbonatus (Reuss); Dailey, p. 767, pl. 6, figs. 1–3
-
1983 Oridorsalis umbonatus (Reuss); Tjalsma and Lohmann, p. 18, pl. 6, figs. 8a–b
-
1986 Oridorsalis umbonatus (Reuss); Kurihara and Kennett, pl. 6, figs. 11–13
-
1989 Oridorsalis umbonatus (Reuss); Hermelin, pl. 16, figs. 1–5
-
1994 Oridorsalis umbonatus (Reuss); Bolli et al., p. 372, pl. 58, figs. 10-13
-
1999 Oridorsalis umbonatus (Reuss); Kawagata, fig. 10.4
-
2001 Oridorsalis umbonatus (Reuss); Alegret and Thomas, pl. 9, fig. 8–9
-
2001 Oridorsalis umbonatus (Reuss); Hayward et al., figs. 16R–S
-
2010 Oridorsalis umbonatus (Reuss); Hayward et al., pl. 30, figs. 3–6
-
2013 Oridorsalis umbonatus (Reuss); Alegret and Thomas, pl. I, fig. 16
-
2019 Oridorsalis umbonatus (Reuss); Ridha et al., fig. 6: 6a–d
Description. Test trochospiral, unequally biconvex, and lenticular. The outline is lobulated with an acute periphery. The spiral side is more evolute and convex, and the umbilical side is involute and less convex. Five to six chambers in the last whorl. On the spiral side, the chamber sutures are at a right angle to the spiral suture, and on the umbilical side the sutures are radial and slightly curved towards the umbo. Wall calcareous and smooth. The aperture is an interiomarginal slit extending from the periphery to the umbilicus.
Remarks. The lobulated outline and curved sutures towards the umbo and the chamber sutures at right angles to the spiral suture serve as the primary diagnostic features of O. umbonatus. At Site U1506, the size of O. umbonatus varies from small to moderately large. In some specimens the last chamber is broken or completely missing.
Paleoecology and paleobathymetric distribution. Oridorsalis umbonatus has a carbon isotope signature typical for shallow infaunal taxa (e.g. Thomas and Shackleton, 1996; Katz et al., 2003). It has been reported from lower bathyal to abyssal depths in the Indian, Atlantic, and Pacific oceans and the Southern Ocean (Corliss, 1979; Streeter and Shackleton, 1979; Gupta, 1994; Hayward et al., 2010; Alegret and Thomas, 2013). Previous studies have associated this species with high-oxygen environments (Hayward et al., 2010).
-
Family Osangulariidae Loeblich and Tappan 1964
-
Genus Osangularia Brotzen 1940
-
Osangularia culter (Parker and Jones 1865)
Fig. 6: 1a–c
-
1865 Planorbulina farcta var. ungeriana subvar. culter Parker and Jones, pp. 382, 421, pl. 19, fig. 1
-
1866 Anomalina bengalensis Schwager; Schwager, p. 259, pl. 7, fig. 111
-
1884 Truncatulina culter (Parker and Jones); Brady, p. 668, pl. 96, fig. 3
-
1953 Osangularia culter (Parker and Jones); Phleger, Parker and Pierson, p. 42, pl. 9, figs. 11–16
-
1979 Osangularia culter (Parker and Jones); Hayward and Buzas, pl. 15, figs. 193–194; pl. 22, figs. 279–281
-
1989 Osangularia culter (Parker and Jones); Hermelin, p. 84, pl. 16, figs. 11–13
-
1994 Osangularia bengalensis (Schwager); Jones, p. 100, pl. 96, fig. 3
-
1995 Osangularia culter (Parker and Jones); Nomura, p. 278, pl. 4, fig. 8
-
2013 Osangularia culter (Parker and Jones); Holbourn et al., p. 386, figs. 1-2
-
2019 Osangularia culter (Parker and Jones); Ridha et al., fig. 6: 7a–c
Description. Test trochospiral and lenticular. The umbilical side is more convex and involute, and the spiral side is less convex and evolute. The periphery is acute with a thin, serrate keel; 8 to 10 chambers in the last whorl; sutures are raised and curved on the spiral side and depressed and radial on the umbilical side. Wall calcareous, smooth, finely perforated. The aperture is an interiomarginal slit extending from the umbilicus to the periphery.
Remarks. Specimens at Site U1506 were ascribed to O. culter based on the original description by Parker and Jones (1865). Our specimens exhibit a relatively good state of preservation, with the peripheral keel remaining intact.
Paleoecology and paleobathymetric distribution. This species, known for its wide distribution, has been documented globally. Previous studies (Brady, 1884; Frerichs, 1970; Pflum and Frerichs, 1976; Holbourn et al., 2013) suggest that this cosmopolitan species is typically found at depths not exceeding 700 m.
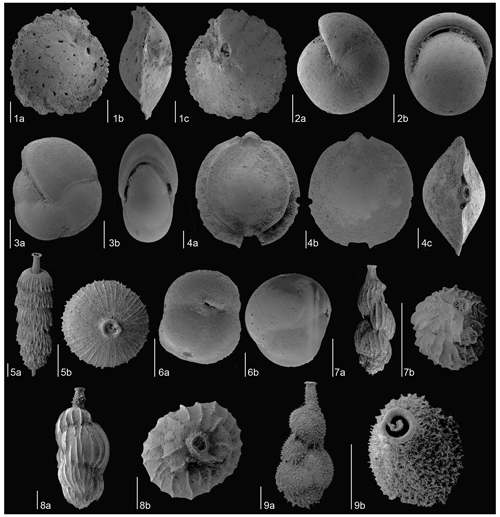
Figure 6SEM images of the most common benthic foraminiferal species at Site U1506 identified in the Late Miocene–Early Pliocene. (1) Osangularia culter (sample U1506A-18R-4W, 50–52 cm), MCNUZ-MPZ-2023/362: (a) dorsal side, (b), side view, (c) ventral side. (2) Pullenia bulloides (sample U1506A-21R-4W, 140–142 cm), MCNUZ-MPZ-2023/363: (a) side view, (b), apertural view. (3) Pullenia quinqueloba (sample U1506A-19R-4W, 97–99 cm), MCNUZ-MPZ-2023/364: (a) side view, (b), apertural view. (4) Pyrgo murrhina (sample U1506A-16R-1W, 136–138 cm), MCNUZ-MPZ-2023/365: (a) frontal side, (b) dorsal side, (c) apertural view. (5) Rectuvigerina multicostata (sample U1506A-20R-6W, 50–52 cm), MCNUZ-MPZ-2023/366: (a) frontal side, (b) outline view. (6) Sphaeroidina bulloides (sample U1506A-18R-5W, 116–118 cm), MCNUZ-MPZ-2023/367: (a) frontal side. (6) Sphaeroidina bulloides (sample U1506A-18R-5W, 116–118 cm), MCNUZ-MPZ-2023/368: (b) side view. (7) Uvigerina occidentalis (sample U1506A-12R-1W, 110–112 cm), MCNUZ-MPZ-2023/369: (a) frontal side, (b) outline view. (8) Uvigerina peregrina (sample U1506A-17R-2W, 133–135 cm), MCNUZ-MPZ-2023/370: (a) frontal side, (b) outline view. (9) Uvigerina proboscidea (sample U1506A-11R-3W, 30–32 cm), MCNUZ-MPZ-2023/371: (a) frontal side, (b) outline view. All scale bars = 100 µm.
-
Family Nonionidae Schultze 1854
-
Genus Pullenia Parker and Jones 1862
-
Pullenia bulloides (d'Orbigny 1846)
Fig. 6: 2a–b
-
1846 Nonionina bulloides d'Orbigny; d'Orbigny, p. 107, pl. 5, figs. 9–10
-
1884 Pullenia sphaeroides (d'Orbigny); Brady, p. 615, pl. 84, figs. 12–13
-
1960 Pullenia bulloides (d'Orbigny); Barker pl. 84, figs. 12–13
-
1975 Pullenia sphaeroides (d'Orbigny); Saidova, pl. 70, fig. 7
-
1986 Pullenia bulloides (d'Orbigny); Kurihara and Kennett, pl. 6, figs. 5–6
-
1989 Pullenia bulloides (d'Orbigny); Hermelin, pl. 15, figs. 4–5
-
1990 Pullenia bulloides (d'Orbigny); Thomas et al., pl. 10, fig. 15
-
1994 Pullenia bikiniensis McCulloch; Loeblich and Tappan, p. 159, pl. 348, figs. 9–14
-
1999 Pullenia bulloides (d'Orbigny); Kawagata, fig. 9.5
-
2001 Pullenia bulloides (d'Orbigny); Hayward et al., figs. 16T–U
-
2010 Pullenia bulloides (d'Orbigny); Hayward et al., pl. 29, figs. 15–17
-
2013 Pullenia bulloides (d'Orbigny); Holbourn et al., p. 442, figs. 1–3
-
2019 Pullenia bulloides (d'Orbigny); Ridha et al., fig. 7: 7a–b
Description. Test globular, planispiral, and involute. The outline is circular, and the periphery is broadly rounded. Four to five inflated chambers in the last whorl, separated by radial and slightly depressed sutures. Wall calcareous and smooth. The aperture is a narrow interiomarginal slit, stretching from the periphery to the umbilici.
Remarks. At Site U1506, the specimens belonging to P. bulloides exhibit excellent preservation and are characterised by moderately large tests consisting of three to six chambers with close umbilici. This species closely resembles P. quinqueloba, but it can be distinguished by its more subglobular shape and circular outline.
Paleoecology and paleobathymetric distribution. Pullenia bulloides is a cosmopolitan species found at middle to lower bathyal depths in the Pacific, as documented by Smith (1964). In the vicinity of New Zealand, Hayward et al. (2010) reported its highest abundance at middle bathyal to abyssal depths.
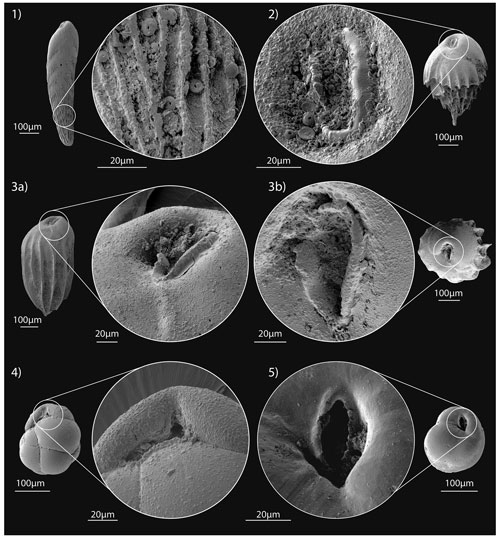
Figure 7Close-up SEM images of selected Late Miocene–Early Pliocene benthic foraminiferal species at Site U1506. (1) Bolivina finlayi (sample U1506A-21R-1W, 140–142 cm), MCNUZ-MPZ-2023/343, close-up view of the striae; (2) Bulimina striata (sample U1506A-19R-4W, 97–99 cm), MCNUZ-MPZ-2023/346, detail of the aperture; (3a–b) Bulimina truncana (sample U1506A-21R-4W, 140–142 cm), MCNUZ-MPZ-2023/347, aperture; (4) Globocassidulina crassa (sample U1506A-11R-1W, 105–107 cm), MCNUZ-MPZ-2023/353, aperture; (5) Globocassidulina subglobosa (sample U1506A-11R-1W, 105–107 cm), MCNUZ-MPZ-2023/354, aperture.
-
Family Nonionidae Schultze, 1854
-
Genus Pullenia Parker and Jones, 1862
-
Pullenia quinqueloba (Reuss, 1851)
Fig. 6: 3a–b
-
1851 Nonionina quinqueloba Reuss; Reuss, p.71, pl.5, figs. 31a–b
-
1884 Pullenia quinqueloba (Reuss); Brady, p. 617, pl. 84, figs. 14–15
-
1975 Pullenia borealis Saidova; Saidova, pl. 69, fig. 9
-
1983 Pullenia quinqueloba (Reuss); Tjalsma and Lohmann, p. 36, pl. 16, fig. 2
-
1986 Pullenia osloensis Feyling-Hanssen; Kurihara and Kennett; pl. 6, figs. 7–8
-
1990 Pullenia quinqueloba (Reuss); Thomas et al., pl. 10, fig. 19
-
1994 Pullenia borealis Saidova; Loeblich and Tappan, pl. 348, figs. 1–6
-
1994 Pullenia quinqueloba (Reuss); Jones, pl. 84, figs. 14–15
-
2010 Pullenia quinqueloba (Reuss); Hayward et al., pl. 29 figs. 18–20
-
2013 Pullenia quinqueloba (Reuss); Holbourn et al., p. 448, figs. 1–2
-
2019 Pullenia quinqueloba (Reuss); Ridha et al., fig. 7: 8a–b
Description. Test globular, planispiral, and involute. Compressed in apertural view and with a rounded periphery in umbilical view. Five chambers with a triangular shape, inflated and gradually increasing in size in the last whorl. The sutures are depressed, radial, and slightly curved. Wall calcareous, smooth, and finely perforated. The aperture is a narrow interiomarginal slit, stretching from the periphery to the umbilici.
Remarks. Specimens ascribed to P. quinqueloba generally display a small size and are well preserved. Pullenia quinqueloba is distinguished from P. bulloides by its smaller and more compressed test, characterised by five triangular chambers and a more lobulated outline.
Paleoecology and paleobathymetric distribution. Pullenia quinqueloba has a wide paleobathymetric range, from middle shelf to abyssal (50–5000 m), with slightly higher relative abundances at abyssal depths in New Zealand (Hayward et al., 2010).
-
Family Hauerinidae Schwager 1876
-
Genus Pyrgo Defrance 1824
-
Pyrgo murrhina (Schwager 1866)
Fig. 6: 4a–c
-
1866 Biloculina murrhina Schwager; Schwager, p. 203, pl. 4, figs. 15a–c
-
1945 Pyrgo murrhina (Schwager); Cushman and Todd, p. 12, pl. 2, fig. 5
-
1986 Pyrgo murrhina (Schwager); Van Morkhoven et al., p. 50, pl. 15
-
1986 Pyrgo murrhina (Schwager); Kurihara and Kennett; pl. 1, fig. 13
-
1989 Pyrgo murrhina (Schwager); Hermelin, pl. 2, figs. 12, 15–16
-
1994 Pyrgo murrhina (Schwager); Loeblich and Tappan, pl. 91, figs. 11–15
-
1994 Pyrgo murrhina (Schwager); Jones, pl. 2, figs. 10–11, 15
-
1998 Pyrgo murrhina (Schwager); Robertson, p. 34, pl. 10, fig. 2
-
2010 Pyrgo murrhina (Schwager); Hayward et al., pl. 7, figs. 20–23
-
2013 Pyrgo murrhina (Schwager); Holbourn et al., p. 458, figs. 1–2
Description. Test large, slightly compressed, circular to oval in outline, and elliptical in cross-section. The periphery presents a wide peripheral flange with a keel and two small spines on the opposite side of the aperture. Wall porcellaneous and smooth. The aperture is circular to oval, and it ends with a short bifid tooth at the end of a short neck.
Remarks. Complete specimens of P. murrhina are uncommon at Site U1506, with the fragile neck and small spines often missing. Instead, we predominantly found fragmented pieces, typically comprising only one side of the test. We identified this species by the presence of a compressed test, an oval outline, and a keel that despite being occasionally damaged remains visible in many cases.
Paleoecology and paleobathymetric distribution. Pyrgo murrhina has a cosmopolitan distribution (Van Morkhoven et al., 1986). P. murrhina is a bathyal to abyssal species, and most records east of New Zealand range from 1000 m to 4000 m; its upper depth limit off the east coast of New Zealand shallows southwards, from middle–upper bathyal up to the upper shelf around the subantarctic islands (Bandy and Arnal, 1957; Hayward et al., 2010).
-
Family Siphogenerinoididae Saidova 1981
-
Genus Rectuvigerina Mathews 1945
-
Rectuvigerina multicostata (Cushman and Jarvis 1929)
Fig. 6: 5a–b
-
1929 Siphogenerina multicostata Cushman and Jarvis: p. 14, pl. 3, fig. 6
-
1949 Siphogenerina spinea Bermúdez; Bermúdez, p. 223, pl. 13, figs. 78–79
-
1986 Rectuvigerina multicostata (Cushman and Jarvis); Van Morkhoven et al., p. 115, pl. 36
-
1986 Rectuvigerina sp.; Kurihara and Kennett, pl. 3, fig. 9
-
1987 Rectuvigerina multicostata (Cushman and Jarvis); Loeblich and Tappan, p. 519, pl. 569, figs. 28–29
-
1993 Rectuvigerina multicostata (Cushman and Jarvis); Katz and Miller, pl. 1, fig. 2
-
1998 Rectuvigerina multicostata (Cushman and Jarvis); Robertson, p. 144, pl. 55, figs. 6–7
-
2013 Rectuvigerina multicostata (Cushman and Jarvis); Holbourn et al., p. 47, fig. 1
Description. Test elongated, initially triserial and later uniserial. The chambers are inflated and gradually increase in size. The chambers are separated by depressed sutures. Wall calcareous with fine pores and longitudinal costae, which can form short spines, mostly in the early chambers. Some specimens present a basal spine. The aperture is terminal and rounded with a lip at the end of a long neck.
Remarks. The holotype of R. multicostata (USNM CC 10056) was checked at the Smithsonian Institution. Our specimens fully agree with the holotype and the original description by Cushman and Jarvis (1929). At Site U1506, R. multicostata has commonly large tests. The best-preserved specimens show the basal spine and a wide, thin lip around the aperture.
Paleoecology and paleobathymetric distribution. This species has been documented at bathyal depths (Van Morkhoven et al., 1986), and it thrives in regions characterised by a significant influx of organic matter resulting from elevated surface productivity (Holbourn et al., 2013).
-
Family Sphaeroidinidae Cushman 1927
-
Genus Sphaeroidina d'Orbigny 1826
-
Sphaeroidina bulloides d'Orbigny 1826
Fig. 6: 6a–b
-
1826 Sphaeroidina bulloides d'Orbigny: p. 267, Model No. 65, illustrated in Parker et al., 1865, pl. 2, fig. 58
-
1846 Sphaeroidina austriaca d'Orbigny: p. 284, pl. 20, figs. 19–21
-
1884 Sphaeroidina bulloides d'Orbigny; Brady, p. 620, pl. 84, figs. 1–2
-
1949 Sphaeroidina ciperana Cushman and Todd; p. 67, pl. 12, fig. 14
-
1949 Sphaeroidina compacta Cushman and Todd; p. 19, pl. 4, fig. 14
-
1949 Sphaeroidina compressa Cushman and Todd; p. 20, pl. 4, figs. 15–16
-
1949 Sphaeroidina nitida Cushman and Todd; p. 20, pl. 4, fig. 13
-
1953 Sphaeroidina japonica Asano; p. 19, pl. 2, figs. 43–44
-
1986 Sphaeroidina bulloides d'Orbigny; Van Morkhoven et al., p. 80, pl. 24
-
1989 Sphaeroidina bulloides d'Orbigny; Kurihara and Kennett, pl. 2, fig. 1
-
1994 Sphaeroidina bulloides d'Orbigny; Jones, p. 91, pl. 84, figs. 1–2
-
1999 Sphaeroidina bulloides d'Orbigny; Hayward et al., pl. 11, figs. 15–16
-
2010 Sphaeroidina bulloides d'Orbigny; Hayward et al., pl. 32, figs. 12–13
-
2013 Sphaeroidina bulloides d'Orbigny; Holbourn et al., p. 520, figs. 1–3
-
2019 Sphaeroidina bulloides d'Orbigny; Ridhaa et al., fig. 7: 2a–c
Description. Test subglobular, typically with three chambers visible and the last chamber covering half of the test. The sutures are slightly depressed. Wall calcareous and smooth. The aperture is round with a lip and a simple tooth.
Remarks. Our specimens are well preserved. Notably, the larger specimens display a thicker, opaque wall, whereas the smaller specimens are characterised by more translucent and thinner walls.
Paleoecology and paleobathymetric distribution. Sphaeroidina bulloides is a cosmopolitan species; its bathymetric distribution spans from shelf to abyssal depths (Van Morkhoven et al., 1989; Holbourn et al., 2013). Around New Zealand, it is most common between outer shelf and lower bathyal depths (100–1000 m; Hayward et al., 2010). Its upper depth limit is in the middle shelf (Van Morkhoven et al., 1986). Kaiho (1994) included S. bulloides in the suboxic group.
-
Family Uvigerinidae Haeckel 1894
-
Genus Uvigerina d'Orbigny 1826
-
Uvigerina occidentalis Cushman 1923
Fig. 6: 7a–b
-
1923 Uvigerina occidentalis Cushman, p. 34, pl. 5, figs. 3–4
-
1995 Uvigerina sp.; Yassini and Jones, fig. 613
-
2001 Trifarina occidentalis (Cushman); Hayward et al., figs. 14UU–VV
-
2010 Trifarina occidentalis (Cushman); Hayward et al., pl. 21, figs. 18–20
Description. Test small, minute, and elongated and widest in the middle. Triangular to subcircular in cross-section. Chambers distinct and progressively increasing in size, sutures incised. Wall calcareous and ornamented with longitudinal fine costae, continuous over chambers but not across sutures. The aperture is terminal with a lip, at the end of the neck.
Remarks. Our specimens fully agree with the holotype (USNM CC 2357) and with the original description by Cushman (1923). Hayward et al. (2010) assigned this species to the genus Trifarina; which is characterised by having sharp edges, “angles sharp to longitudinally carinate” according to Loeblich and Tappan (1988). However, our specimens display more rounded edges, and therefore we assigned them to the genus Uvigerina. Uvigerina occidentalis was differentiated from other Uvigerina species based on its small, elongated, and fusiform test ornamented with costae. In contrast, the other Uvigerina species with costae (e.g. Uvigerina peregrina) exhibit larger tests and inflated chambers.
Paleoecology and paleobathymetric distribution. In the vicinity of New Zealand, U. occidentalis ranges from outer shelf to abyssal, and it is most abundant at bathyal depths (Hayward et al., 2010).
-
Family Uvigerinidae Haeckel 1894
-
Genus Uvigerina d'Orbigny 1826
-
Uvigerina peregrina s.l. Cushman 1923
Fig. 6: 8a–b
-
1923 Uvigerina peregrina Cushman: p. 166, pl. 42, figs. 7–10
-
1986 Uvigerina peregrina Cushman; Kurihara and Kennett, pl. 3, figs. 1–3
-
1987 Uvigerina peregrina Cushman; Loeblich and Tappan, pl. 573, figs. 24–28
-
1989 Uvigerina peregrina Cushman; Hermelin, pl. 12, figs. 6–8
-
1999 Uvigerina peregrina Cushman; Kawagata, figs. 5–8
-
2001 Uvigerina peregrina Cushman; Hayward et al., figs. 14WW–XX
-
2003 Uvigerina peregrina Cushman; Hayward et al., fig. 4M
-
2005 Uvigerina peregrina Cushman; Schönfeld and Altenbach, fig. 2c
-
2008 Uvigerina peregrina Cushman; Lobegeier and Sen Gupta, pl. 3, fig. 11
-
2010 Uvigerina peregrina Cushman; Hayward et al., pl. 21, figs. 27–31
-
2019 Uvigerina peregrina Cushman; Ridha et al., fig. 5: 6a
Description. Test small to medium in size, triserial, and fusiform. Generally, 2.5 times as long as wide. Chambers inflated and gradually increasing in size. Wall calcareous and ornamented with raised longitudinal costae, not continuous but separated by depressed sutures. The basal end is often ornamented by short spines. The aperture is rounded and terminal, at the end of a short cylindrical neck, typically covered in small spines.
Remarks. The holotype of U. peregrina checked at the Smithsonian (USNM PP 17574) shows deep costae that reach the last chambers. Following Hayward et al. (2010), we used a broad concept of this species (Uvigerina peregrina s.l.). The specimens observed at Site U1506 exhibit distinctive features such as large tests, inflated chambers, and longitudinal costae; however, the high variability in these characteristics made differentiating distinct taxa impossible. The overall preservation is reasonably good; however, the neck region is frequently found to be broken. In the best-preserved specimens, small spines were observed around the neck area.
Paleoecology and paleobathymetric distribution. This cosmopolitan species has been documented from shelf to abyssal depths. Around New Zealand, it occurs with the highest abundance from middle bathyal to abyssal depths; in the South Atlantic, it is common at lower bathyal depths (Mead, 1985: Schönfeld and Altenbach, 2005; Schweizer, 2006; Hayward et al., 2010). Uvigerina peregrina has been linked to oxygen-depleted water masses and eutrophic conditions at the seafloor (Lohmann, 1978; Streeter and Shackleton, 1979; Schnitker, 1979; Miller and Lohmann, 1982).
-
Family Uvigerinidae Haeckel 1894
-
Genus Uvigerina d'Orbigny 1826
-
Uvigerina proboscidea Schwager 1866
Fig. 6: 9a–b
-
1866 Uvigerina proboscidea Schwager: p. 250, pl. 7, fig. 96
-
1945 Uvigerina proboscidea Schwager; Cushman and Todd, p. 50, pl. 7, figs. 28–29
-
1986 Uvigerina proboscidea Schwager; Kurihara and Kennett, pl. 3, fig. 6
-
1986 Uvigerina proboscidea Schwager; Van Morkhoven et al., p. 28, pl. 6.
-
1993 Uvigerina proboscidea Schwager; Katz and Miller, pl. 4, fig. 8
-
1995 Uvigerina proboscidea Schwager; Nomura, p. 274, pl. 2, fig. 5
-
2001 Neouvigerina proboscidea Schwager; Hayward et al., fig. 14RR
-
2010 Neouvigerina proboscidea Schwager; Hayward et al., pl. 21, figs. 9–11
-
2013 Uvigerina proboscidea Schwager; Holbourn et al., p. 602, figs. 1–3
-
2019 Uvigerina proboscidea Schwager; Ridha et al., fig. 5: 5c
Description. The test is triserial and elongate, about 2.5 times as long as broad. Circular in cross-section with a basal spine. The inflated chambers have a subspherical shape and are separated by depressed sutures. Wall calcareous, finely perforated, and hispid. The aperture is terminal with a lip, located at the end of a short neck.
Remarks. Specimens observed at Site U1506 exhibit good preservation. The neck area is frequently preserved without damage. Among the Uvigerina species observed at Site U1506, U. proboscidea is the only species without costae and being solely ornamented by short spines.
Paleoecology and paleobathymetric distribution. The greatest abundance around New Zealand ranges from the outer shelf to upper bathyal depths (100–600 m; Hayward et al., 2010). This species has been associated with high-productivity conditions and low seasonality of the food supply (Loubere, 1998; Gupta and Thomas, 1999; Loubere and Fariduddin, 1999).
4.1 Paleobathymetry
Shipboard and post-cruise studies of benthic foraminifera and ostracods indicate lower bathyal depths from the Miocene to the Pliocene at Site U1506 (Sutherland et al., 2019b, 2020). Here we present a more detailed analysis of the paleodepth distribution of benthic foraminifera across the Late Miocene–Early Pliocene at this site. Our paleobathymetric estimates are based on the presence and abundance of depth-dependent species, with paleodepths and upper depth limits derived from Van Morkhoven et al. (1986) and Hayward et al. (1999, 2001, 2004, 2010). The pattern of occurrence of benthic foraminifera at various depths is shown in Fig. 8.
The occurrence of species such as Heterolepa dutemplei (up to 7 %), Eggerella bradyi (up to 4 %), Karreriella bradyi (up to 4 %), Osangularia culter (1 %–18 %), and Textularia lythostrota (up to 4 %), which have their upper depth limit in the outer shelf zone and are not typically found at depths shallower than ca. 600 m, suggests deposition at paleodepths deeper than upper-shelf bathyal settings. The study interval contains a high percentage of species that are common at lower bathyal to abyssal depths, such as Bulimina truncana (up to 11 %), Cibicidoides mundulus (up to 9 %), Epistominella exigua (up to 13 %), Globocassidulina subglobosa (up to 20 %), Oridorsalis umbonatus (up to 11 %), and Uvigerina peregrina (up to 16 %). Stilostomellids (maximum abundance 13 %) and Nodosariids (maximum abundance 9 %) have been observed in all the samples and are typically abundant at bathyal and abyssal depths (Hayward et al., 1999, 2002; Kennett and Casey, 1969). Abyssal taxa (> 2000 m) such as Nuttallides umbonifera make up < 3 % of the assemblages.
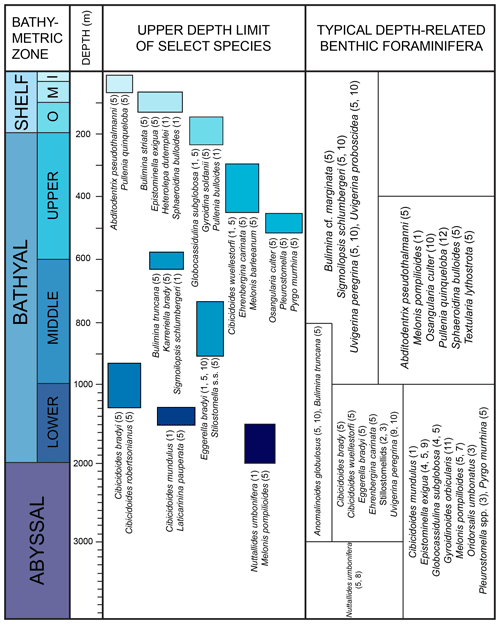
Figure 8Paleobathymetric distribution and upper depth limits of Neogene benthic foraminifera. (1) Van Morkhoven et al. (1986), (2) Hayward et al. (1999), (3) Hayward et al. (2001), (4) Hayward et al. (2004), (5) Hayward et al. (2010), (6) Smith (1964), (7) Igle and Keller (1980), (8) Douglas and Woodruff (1981), (9) Mead (1985), (10) Pflum and Frerichs (1976), (11) Corliss (1979), (12) Ingle (1980). Modified after Van Morkhoven et al. (1986).
Based on these new data and in agreement with Sutherland et al. (2019b, 2020), we interpret the study interval at Site U1506 deposited in the lower bathyal zone, between ca. 1000 and 2000 m paleodepth.
4.2 Paleoenvironmental interpretations
The comparison of recent and fossil benthic foraminifera is a valuable tool to reconstruct paleoenvironmental parameters such as nutrient supply to the seafloor, bottom water oxygenation, or seasonality (e.g. Bernhard, 1986; Jorissen et al., 1995; Fontanier et al., 2002). This approach is commonly employed in paleoceanographic studies, but we acknowledge that the ecology of present-day foraminifera is not fully resolved (Murray, 2006).
At Site U1506, the paleoenvironmental and paleoceanographic changes across the Late Miocene–Early Pliocene were documented by Gastaldello et al. (2023a), who carried out a multi-proxy analysis and identified two intervals with paleoenvironmental significance. The older interval (Interval 1, from 221.75 to 194.75 m, 7.4 to 6.7 Ma) is characterised by a high relative abundance of PETs (e.g. E. exigua, G. crassa, G. subglobosa), indicative of high seasonal surface primary productivity due to phytoplankton blooms. The low abundance of species that can thrive in oxygen-deficient conditions (e.g. Bulimina, Uvigerina; Kaiho, 1994) and the high coarse fraction accumulation rates point to intense bottom currents and well-oxygenated waters during this interval (Gastaldello et al., 2023a). The younger interval (Interval 2, from 194.75 to 82.01 m, 6.7 to 4.5 Ma) is characterised by abundant infaunal morphogroups and species adapted to low-oxygen conditions and high productivity (such as Bolivina, Bulimina, Fursenkoina, Melonis, and Uvigerina species; Kaiho, 1994; Smart et al., 2007), pointing to a continuous and high food supply and oxygen deficiency at the seafloor (Gastaldello et al., 2023a).
The change in productivity regime and current strength at 6.7 Ma correlates with a stratigraphic change in the seismic profiles, which show a switch from complex bedforms prior to 6.7 Ma to a layer-cake pattern afterwards (Gastaldello et al., 2023a). The diverse expression of the Biogenic Bloom has been related to a change in ocean and atmospheric circulation during the Late Miocene. During Interval 1, the northward migration of the Westerlies and the strengthening of the East Australian Current (EAC) led to an increased influence of this current at Site U1506, accounting for the strong bottom currents and the productivity conditions inferred from benthic foraminifera (Gastaldello et al., 2023a). After 6.7 Ma, the southward movement and the weakening of the Westerlies and the EAC led to weaker bottom currents. The intensification of the Asian monsoon, the drying of the Asian landmass, and the resulting weathering increase could have led to a higher food supply to the seafloor during Interval 2, as inferred from benthic foraminifera (Gastaldello et al., 2023a).
Based on new data from this study, we redefined the thickness and the position of Interval 2 (old Interval 2 from Gastaldello et al., 2023a), which now spans from 194.75 to 103.21 m (6.7 to 4.9 Ma), and defined a third interval between 103.21 and 82.01 m (4.9 to 4.5 Ma) (Fig. 9). The previous paleoenvironmental interpretations of Interval 2 from Gastaldello et al. (2023a) remain unchanged. Interval 3 spans from 4.9 to 4.5 Ma (103.21 and 82.01 m), the upper part of the study section, and is characterised by high BFARs (maximum value of ca. 3800 at 96.05 m), low diversity and heterogeneity, low relative abundance of buliminids s.l. and Uvigerina, and high relative abundance of PETs (e.g. A. weddellensis, E. exigua, and G. crassa) (Fig. 9). The high BFARs are probably linked to the rapid proliferation of PETs, as these small-sized benthic foraminifera tend to form large populations, which in turn increase the test counts per gram of sediment. These assemblage changes suggest a transition in the productivity regime from a phase of continuous food supply and low-oxygen conditions observed during Interval 2 to a phase of increased seasonality in food supply, along with well-oxygenated bottom waters, during Interval 3. These observations collectively suggest a scenario of elevated productivity, as indicated by high BFARs, small-sized specimens, and abundance peaks in PETs and opportunistic taxa. These findings could potentially be interpreted in terms of high seasonal surface primary productivity driven by phytoplankton blooms.
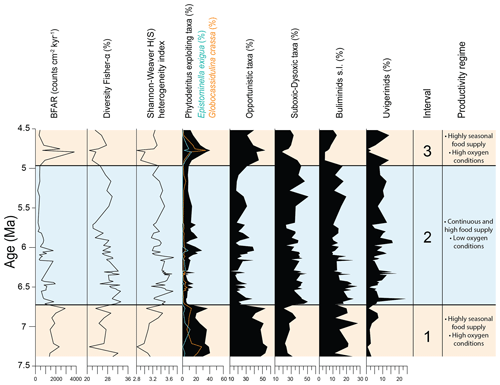
Figure 9Changes in benthic foraminiferal proxies and relative abundance of selected taxa across the Late Miocene–Early Pliocene at IODP Site U1506 plotted against age (Ma). Highlighted Interval 1 (orange), Interval 2 (blue), and Interval 3 (orange) indicate the productivity regimes.
The paleoenvironmental conditions inferred for Interval 3 are comparable to those in Interval 1, although both the benthic foraminifera assemblages and the paleoceanographic settings are not comparable. Considering the benthic foraminifera assemblages, the main difference between Intervals 1 and 3 could be observed in the composition of the PET group. Both intervals 1 and 3 are dominated by PETs, with a similar relative abundance of A. weddellensis, E. exigua, and G. crassa. The main difference between Interval 3 and Interval 1 can be observed in the relative abundance of G. subglobosa, higher in Interval 1 and lower in Interval 3. While G. subglobosa is known to have a preference for high-oxygen conditions (Kaiho, 1999), the decrease in relative abundance of this species in Interval 3 could not be attributed to a change in oxygenation levels since the suboxic–dysoxic taxa show low values in both Interval 1 and 3. The decrease in G. subglobosa could rather be attributed to changes in ocean currents during these two intervals. The productivity regime inferred during Interval 1 in Gastaldello et al. (2023a) has been linked to a northward migration of the Westerlies and a strengthening of the EAC. In turn, the EAC strengthening has been associated with high current activity and high abundance of G. subglobosa and G. crassa. However, in the warmer Early Pliocene (Interval 3), the Westerlies exhibited reduced strength and shifted poleward (to the south) (Brierley et al., 2009; Abell et al., 2021). Moreover, previous studies on the Lord Howe Rise (ODP Site 590) reported a weakening of the EAC during the Early Pliocene related to the closure of the Central American Seaway around 4.8–4.0 Ma (Haug and Tiedemann, 1998; Haug et al., 2001; Steph et al., 2006; Karas et al., 2011). Sea surface temperatures (SSTs) at Site 590 show stable values comparable to modern annual values, indicating that there has been no migration of the EAC over the site during the Early Pliocene (Karas et al., 2011). The low relative abundance of G. subglobosa during Interval 3 could be related to a weaker EAC and to less intense bottom water currents at Site U1506. Instead, compared to the previous interpretation by Gastaldello et al. (2023a), the high abundance of G. crassa documented in both Interval 1 and 3 indicates that this species is not influenced by the bottom water strength as previously thought. The Early Pliocene was characterised by substantially higher global temperatures compared to the present-day climate. The equatorial Pacific was characterised by warmer equatorial SSTs, persistent El Niño conditions, and a minimal east–west gradient (Fedorov et al., 2006; Groeneveld et al., 2006; Steph et al., 2006; Drury et al., 2018). At the same time, the ocean tropical warm pool expanded towards the poles, and the temperature gradient between the Equator and the mid-latitudes experienced a notable decrease, leading to a weakening of the Hadley and Walker cells (Fedorov et al., 2006; Brierley et al., 2009). A modelling study of the Early Pliocene showed that these conditions may have contributed to increased tropical cyclone activity in the Pacific, including the south-western Pacific region, resulting in intensified vertical mixing of the ocean waters (Fedorov et al., 2006). The development of a deeper mixed layer during the winter season is often linked to intensified phytoplankton blooms and the consequent high abundance of PETs. This deeper mixed layer plays a crucial role in transporting nutrients, thereby promoting the enhanced growth rates of phytoplankton during the following spring period (Campbell and Aarup, 1992; Thomas and Gooday, 1996). Therefore, this mechanism could account for the high-productivity conditions inferred from the benthic foraminiferal assemblages during Interval 3. Although the paleoenvironmental conditions in Intervals 1 and 3 are similar, they occurred in different climatic settings and were influenced by distinct climatic processes.
We conducted a comprehensive investigation of Late Miocene–Early Pliocene benthic foraminifera from Site U1506 in the Tasman Sea, with a specific focus on the paleoceanographic phenomenon known as the Biogenic Bloom. Benthic foraminifera exhibit overall well-preserved tests with fine surface details, allowing for accurate taxonomic identification. The taxonomic dataset in this study comprises a systematic description and illustration of the 36 most common species (those making up > 2 % of the assemblages in at least one sample), including taxonomic details and remarks and paleoecological and paleobathymetric annotation. The benthic foraminiferal assemblages in the Tasman Sea consist of cosmopolitan species that have been described from Upper Miocene and Lower Pliocene deposits from other regions and from the Holocene in the south-western Pacific Ocean.
A detailed paleodepth interpretation of Site U1506 across the Late Miocene–Early Pliocene was performed based on the abundance and distribution of depth-dependent benthic foraminifera. The simultaneous occurrence of species not commonly encountered at depths shallower than ca. 600 m (e.g. H. dutemplei, E. bradyi, O. culter) coupled with the present species that are most abundant at lower bathyal to abyssal depths (e.g. E. exigua, G. subglobosa, U. peregrina), alongside the low abundance of abyssal taxa (e.g. N. umbonifera and P. bulloides), indicates a lower bathyal environment at Site U1506.
Three intervals with paleoenvironmental significance were differentiated across the study record. We redefined the position of Interval 2 (6.7 to 4.9 Ma) defined in Gastaldello et al. (2023a) and defined Interval 3 (4.9 to 4.5 Ma). The elevated BFAR and relative abundance of PETs observed during Interval 3 indicate a scenario of high seasonal surface primary productivity driven by phytoplankton blooms. A similar situation was previously noted for Interval 1. However, in this study, we observed a notable difference in the relative abundance among PETs between the two intervals (1 and 3). When PETs are abundant, the phytodetritus species G. subglobosa shows high abundance in Interval 1 but low abundance in Interval 3. This pattern is attributed to varying strengths of bottom currents. Globocassidulina subglobosa exhibits high abundances during periods of intense current activity (Interval 1), while its relative abundance declines when the bottom water currents weaken (Interval 3). Our findings thus contribute to a better understanding of the regional imprint of the Biogenic Bloom in the Tasman Sea and provide insights into the paleoenvironmental and paleoceanographic changes that occurred during this time interval.
Data generated in this study are included in the Supplement and will be available at Pangaea https://doi.org/10.1594/PANGAEA.963742 (Gastaldello et al., 2023b).
The supplement related to this article is available online at: https://doi.org/10.5194/jm-43-1-2024-supplement.
Conceptualisation: MEG, CA, LA; data curation: MEG, LA; investigation: MEG; supervision: CA, LA; writing – original draft: MEG.
At least one of the (co-)authors is a member of the editorial board of Journal of Micropalaeontology. The peer-review process was guided by an independent editor, and the authors also have no other competing interests to declare.
Publisher’s note: Copernicus Publications remains neutral with regard to jurisdictional claims made in the text, published maps, institutional affiliations, or any other geographical representation in this paper. While Copernicus Publications makes every effort to include appropriate place names, the final responsibility lies with the authors.
The authors would like to thank Ann Holbourn and Ellen Thomas for their kind support in some taxonomic identifications and Bruce Hayward and an anonymous reviewer for their very constructive comments, which were material in improving this paper. This work was funded by the University of Padova DOR grant, CARIPARO PhD scholarship, Fondazione Ing. Aldo Gini scholarship, and grant PID2019-105537RB-I00 funded by MCIN/AEI/10.13039/501100011033 and by “ERDF A way of making Europe.” We acknowledge the use of Servicio General de Apoyo a la Investigación-SAI, Universidad de Zaragoza. This research used samples and data provided by the International Ocean Discovery Program (IODP).
This research has been supported by the Ministerio de Ciencia e Innovación (grant no. PID2019-105537RB-I00).
This paper was edited by Moriaki Yasuhara and reviewed by two anonymous referees.
Abell, J. T., Winckler, G., Anderson, R. F., and Herbert, T. D.: Poleward and weakened westerlies during Pliocene warmth, Nature, 589, 70–75, 2021.
Alegret, L. and Thomas, E.: Upper Cretaceous and lower Paleogene benthic foraminifera from northeastern Mexico, Micropaleontology, 47, 269–316, https://doi.org/10.2113/47.4.269, 2001.
Alegret, L. and Thomas, E.: Benthic foraminifera across the Cretaceous/Paleogene boundary in the Southern Ocean (ODP Site 690): Diversity, food and carbonate saturation, Mar. Micropaleontol., 105, 40–51, 2013.
Alegret, L., Harper, D. T., Agnini, C., Newsam, C., Westerhold, T., Cramwinckel, M. J., et al.: Biotic response to early Eocene warming events: Integrated record from offshore Zealandia, north Tasman Sea, Paleoceanogr. Paleocl., 36, e2020PA004179, https://doi.org/10.1029/2020PA004179, 2021.
Andersen, H. V.: Foraminifera of the mudlumps, lower Mississippi River Delta, in: Genesis and Paleontology of the Mississippi River Mudlumps: Louisiana Geol. Survey, Geol. Bull., Vol. 35, pt. 2, p. 107, 1961.
Asano, K. J.: Miocene foraminifera from the Noto Peninsula, Ishikawa Prefecture, Tohoku University, Institute of Geological Paleontology Short Papers, 5, 1–21, 1953.
Bandy, O. L.: Distribution of foraminifera, radiolaria and diatoms in sediments of the Gulf of California, Micropaleontology, 7, 1–26, 1961.
Bandy, O. L. and Arnal, R. E.: Distribution of recent foraminifera off west coast of Central America, AAPG Bull., 41, 2037–2053, 1957.
Barker, R. W.: Taxonomic notes on the species figured by H. B. Brady in his report of the foraminifera dredged by HMS Challenger during the years 1973–1876, American Association of Petroleum Geologists, Special Publication 9, 238 pp., 1960.
Barmawidjaja, D. M., Jorissen, F. J., Puskaric, S. V., and Van der Zwaan, G. J.: Microhabitat selection by benthic foraminifera in the northern Adriatic Sea, J. Foramin. Res., 22, 297–317, 1992.
Berger, W. H., Leckie, R. M., Janecek, T. R., Stax, R., and Takayama, T.: Neogene carbonate sedimentation on ontong java plateau: highlights and open questions, in: Proceedings of the Ocean Drilling Program Scientific Results, Vol. 130, 711–744, 1993.
Bermúdez, P. J.: Tertiary Smaller Foraminifera of the Dominican Republic, Special Publications of the Cushman Laboratory for Foraminiferal Research, Vol. 25, 1–322, 1949.
Bernhard, J. M.: Characteristic assemblages and morphologies of benthic foraminifera from anoxic, organic-rich deposits, Jurassic through Holocene, J. Foramin. Res., 16, 207–215, https://doi.org/10.2113/gsjfr.16.3.207, 1986.
Boersma, A.: Handbook of common Tertiary Uvigerina, Microclimates Press, 1984a.
Boersma, A.: Oligocene and other Tertiary benthic foraminifers from a depth traverse down Walvis Ridge, Deep Sea Drilling Project Leg 74, southeast Atlantic, Initial Rep. Deep Sea, 75, 1273–1300, 1984b.
Boersma, A.: Biostratigraphy and biogeography of Tertiary bathyal benthic foraminifers: Tasman Sea, Coral Sea, and on the Chatham Rise, Deep Sea Drilling Project, Leg 90, edited by: Kennett, J. P. and Von der Borch, C. C., Initial Rep. Deep Sea, 325, 111–171, https://doi.org/10.1127/pala/2022/0132, 1986.
Bolli, H. M., Beckmann, J. P., and Saunders, J. B.: Benthic foraminiferal biostratigraphy of the south Caribbean region, Cambridge University Press, 1994.
Boltovskoy, E. and Giussani de Kahn, G.: Cinco nuevos taxones en Orden Foraminiferida, Communicaciones des Museo Argentino de Ciencias Naturales “Bernardino Rivadavia” e Instituto Nacional de Investigacion de las Ciencias Naturales, Hydrobiologia, 2, 43–51, 1981.
Boscolo Galazzo, F., Thomas, E., and Giusberti, L., Benthic foraminiferal response to the Middle Eocene climatic optimum (MECO) in the south-eastern Atlantic (ODP Site 1263), Palaeogeogr. Palaeocl., 417, 432–444, https://doi.org/10.1016/j.palaeo.2014.10.004, 2015.
Brady, H. B.: Report on the Foraminifera dredged by HMS Challenger, during the years 1873–1876, Reports of the scientific results of the voyage of HMS challenger, Zoology, 9, 1–184, 1884.
Brady, H. B., Parker, W. K., and Jones, T. R.: On some foraminifera from the Abrolhos Bank, Trans. Zool. Soc. Lond., 12, 211–239, 1888.
Brierley, C. M., Fedorov, A. V., Liu, Z., Herbert, T. D., Lawrence, K. T., and LaRiviere, J. P.: Greatly expanded tropical warm pool and weakened Hadley circulation in the early Pliocene, Science, 323, 1714–1718, 2009.
Brotzen, F.: Die Foraminiferengattung Gavelinella nov. gen. und die Systematik der Rotaliiformes, Sveriges Geologiska Undersökning, 36, p. 19, p. 23, 1942.
Campbell, J. W. and Aarup, T.: New production in the North Atlantic derived from seasonal patterns of surface chlorophyll, Deep-Sea Res. Pt. A, 39, 1669–1694, 1992.
Chapman, F. and Parr, W. J.: Foraminifera, in: Australasian Antarctic Expedition 1911–1914, edited by: Johnston, T. H., Scientific Reports, Series C (Zoology and Botany), Sydney, David Harold Paisley, 1, 1–190, 1937.
Chapman, F., Parr, W. J., and Collins, A. C.: Tertiary Foraminifera of Victoria, Australia-The Balcombian Deposits of Port Phillip, Part III, Journal of the Linnean Society of London, Zoology, 38, 553–577, 1934.
Chiji, M. and Lopez, S. M.: Regional foraminiferal assemblages in Tanabe Bay, Kii Peninsula, central Japan, Publ. Seto Mar. Biol. Labor., 16, 85–125, 1968.
Corliss, B. H.: Taxonomy of Recent deep-sea benthonic foraminifera from the southeast Indian Ocean, Micropaleontology, 25, 1–19, https://doi.org/10.2307/1485207, 1979.
Corliss, B. H.: Morphology and microhabitat preferences of benthic foraminifera from the northwest Atlantic Ocean, Mar. Micropaleontol., 17, 195–236, 1991.
Corliss, B. H. and Chen, C., Morphotype patterns of Norwegian Sea deep-sea benthic foraminifera and ecological implications, Geology, 16, 716–719, 1988.
Corliss, B. H., Brown, C. W., Sun, X., and Showers, W. J.: Deep-sea benthic diversity linked to seasonality of pelagic productivity, Deep-Sea Res. Pt. I, 56, 835–841, 2009.
Cushman, J. A.: A monograph of the Foraminifera of the North Pacific Ocean, Part II. Textulariidae, Bulletin of the United States National Museum, 71, 1–108, 1911.
Cushman, J. A.: A monograph of the foraminifera of the North Pacific Ocean, US Government Printing Office, 1913.
Cushman, J. A.: Foraminifera of the Philippine and adjacent seas, Bulletin of the United States National Museum, 100, 1–608, 1921.
Cushman, J. A.: Shallow- water foraminifera of the Tortugas region. Publications of the Carnegie Institution of Washington, no. 311, Depart. Mar. Biol., 17, p. 85, 1922.
Cushman, J. A.: The Foraminifera of the Atlantic Ocean, Part 4: Lagenidae, United States National Museum Bulletin, 104, 228 pp., 1923.
Cushman, J. A.: American Upper Cretaceous species of Bolivina and related species, Contributions from the Cushman Laboratory for Foraminiferal Research, 2, 85–91, 1927.
Cushman, J. A.: Some new foraminiferal genera, Contributions from the Cushman Laboratory for Foraminiferal Research, 9, 32–38, 1933.
Cushman, J. A.: A monograph of the foraminiferal family Valvulinidae, Special Publications, Cushman Laboratory for Foraminiferal Research, 8, 1–210, 1937.
Cushman, J. A. and Bermúdez, P. J.: Further new species of foraminifera from the Eocene of Cuba, Contributions from the Cushman Laboratory for Foraminiferal Research, 13, 1–29, 1937.
Cushman, J. A. and Jarvis, P. W.: New foraminifera from Trinidad, Contributions from the Cushman Laboratory for Foraminiferal Research, 5, 6–17, 1929.
Cushman, J. A. and Todd, R.: The Recent and fossil species of Laticarinina, Contributions from the Cushman Laboratory for Foraminiferal Research, 18, 14–20, 1942.
Cushman, J. A. and Todd, R.: Miocene foraminifera from Buff Bay, Jamaica, Special Publications of the Cushman Laboratory for Foraminiferal Research, 15, 1–73, 1945.
Cushman, J. A. and Todd, R.: The genus Sphaeroidina and its species, Contributions from the Cushman Laboratory for Foraminiferal Research, 25, 11—21, 1949.
Dailey, D. H.: Late cretaceous and Paleocene benthic foraminifers from deep-sea drilling project site-516, rio-grande rise, western South-Atlantic Ocean, Initial Rep. Deep Sea, 72, 757–782, 1983.
Defrance J. L. M.: Dictionnaire des Sciences Naturelles dans lequel on traite méthodiquement des diffrents êtres de la nature, Paris, Vol. 32, p. 273, 1824.
De Rijk, S., Jorissen, F. J., Rohling, E. J., and Troelstra, S. R.: Organic flux control on bathymetric zonation of Mediterranean benthic foraminifera, Mar. Micropaleontol., 40, 151–166, 2000.
Denne, R. A. and Gupta, B. K. S.: Association of bathyal foraminifera with water masses in the northwestern Gulf of Mexico, Mar. Micropaleontol., 17, 173–193, 1991.
Dias, B. B., Barbosa, C. F., Faria, G. R., Seoane, J. C. S., and Albuquerque, A. L. S.: The effects of multidecadal-scale phytodetritus disturbances on the benthic foraminiferal community of a Western Boundary Upwelling System, Brazil, Mar. Micropaleontol., 139, 102–112, 2018.
Dickens, G. R. and Owen, R. M.: The latest Miocene–early Pliocene biogenic bloom: a revised Indian Ocean perspective, Mar. Geol., 161, 75–91, 1999.
Diester-Haass, L., Billups, K., and Emeis, K. C.: In search of the late Miocene–early Pliocene “BB” in the Atlantic Ocean (Ocean ng Program Sites 982, 925, and 1088), Paleoceanography, 20, PA4001, https://doi.org/10.1029/2005PA001139, 2005
Diester-Haass, L., Billups, K., and Emeis, K. C.: Late Miocene carbon isotope records and marine biological productivity: Was there a (dusty) link?, Paleoceanography, 21, PA4216, https://doi.org/10.1029/2006PA001267, 2006.
d'Orbigny, A. D.: Tableau méthodique de la classe des Céphalopodes, Ann. Sci. Naturell., 7, 245–314, 1826.
d'Orbigny, A.: Foraminifères, in: Voyage dans l'Amérique Méridionale (le Brésil, la République orientale de l'Uruquay, la République Argentine, la Patagonie, la République du Chili, la République de Bolivia, la République du Pérou) 238 éxécuté pendant les années 1826, 1827, 1832 et 1833, vol. 5(5), Bertrand, Paris, Levrault, Strasbourg, 1–86, 1839.
d'Orbigny, A. D.: Foraminiferes fossiles du Bassin Tertiaire de Vienne (Autriche) 2, Paris, Gide et Camp, 312 pp., 1846.
d'Orbigny, A. D.: Prodrome de Paleontologie stratigraphique universelle des animaux mollusques et rayonnes. Table alphabetique et synonomique des genres et des especes, Vol. 3, V. Masson, Paris, p. 196, 1852.
Douglas, R. and Woodruff, F.: Deep-sea benthic foraminifera, in: The oceanic lithosphere, edited by: Emilani, C., Vol. 7, The Sea, Wiley, New York, 1233–1327, 1981.
Drury, A. J., Lee, G. P., Gray, W. R., Lyle, M., Westerhold, T., Shevenell, A. E., and John, C. M.: Deciphering the state of the late Miocene to early Pliocene equatorial Pacific, Paleoceanogr. Paleocl., 33, 246–263, 2018.
Drury, A. J., Liebrand, D., Westerhold, T., Beddow, H. M., Hodell, D. A., Rohlfs, N., Wilkens, R. H., Lyle, M., Bell, D. B., Kroon, D., Pälike, H., and Lourens, L. J.: Climate, cryosphere and carbon cycle controls on Southeast Atlantic orbital-scale carbonate deposition since the Oligocene (30–0 Ma), Clim. Past, 17, 2091–2117, https://doi.org/10.5194/cp-17-2091-2021, 2021.
Eade, J. V.: New Zealand Recent foraminifera of the families Islandiellidae and Cassidulinidae, New Zeal. J. Mar. Fresh., 1, 421–454, 1967.
Earland, A.: Foraminifera, Part IV, Additional records from the Weddell Sea sector from material obtained by the S. Y. “Scotia”, Discovery Reports, 13, 1–76, 1936.
Egger, J. G.: Foraminiferen aus Meeresgrundproben: gelothet von 1874 bis 1876, Verlag der K. Akademie, in: Commission, edited by: Franz, G., Vol. 12, 1893.
Ehrenberg, C. G.: Ueber dem blossen Auge unsichtbare Kalkthierchen und Kieselthierchen als Hauptbestandteile der Kreidegebirge, Berichte der Königlich-Preussischen Akademie der Wissenschaften zu Berlin, Vol. 3, 192–200, 1838.
Erbacher, J., Hemleben, C., Huber, B. T., and Markey, M.: Correlating environmental changes during early Albian oceanic anoxic event 1B using benthic foraminiferal paleoecology, Mar/ Micropaleontol/, 38, 7–28, https://doi.org/10.1016/S0377-8398(99)00036-5, 1999.
Fariduddin, M. and Loubere, P.: The surface ocean productivity response of deeper water benthic foraminifera in the Atlantic Ocean, Mar. Micropaleontol., 32, 289–310, 1997.
Farrell, J. W., Raffi, I., Janecek, T. R., Murray, D. W., Levitan, M., Dadey, K. A., et al.: 35. Late neogene sedimentation patterns in the eastern equatorial Pacific Ocean, in: Proceedings of the ocean drilling program, Scientific Results, Vol. 138, 1995.
Fedorov, A. V., Dekens, P. S., McCarthy, M., Ravelo, A. C., DeMenocal, P. B., Barreiro, M., et al.: The Pliocene paradox (mechanisms for a permanent El Niño), Science, 312, 1485–1489, 2006.
Finlay, H. J.: New Zealand Foraminifera: Key Species in Stratigraphy – No. 3, Trans. Roy. Soc. New Zeal., 69, 309–329, 1939.
Finlay, H. J.: New Zealand Foraminifera; Key species in stratigraphy – No. 5, New Zealand J. Sci. Technol., Vol. 28, 259–292, 1947.
Fisher, R. A., Corbet, A. S., and Williams, C. B.: The relation between the number of species and the number of individuals in a random sample of an animal population, J. Anim. Ecol., 12, 42–58, https://doi.org/10.2307/1411, 1943.
Fontanier, C., Jorissen, F. J., Licari, L., Alexandre, A., Anschutz, P., and Carbonel, P.: Live benthic foraminiferal faunas from the Bay of Biscay: faunal density, composition, and microhabitats, Deep-Sea Res. Pt. I, 49, 751–785, https://doi.org/10.1016/S0967-0637(01)00078-4, 2002.
Franzenau, A.: Heterolepa, egy uj genus a foraminiferak rendjében, Term észetrajzi Füzetek, Budapest, Vol. 8, 181–184, 1884.
Frerichs, W. E.: Distribution and ecology of benthonic foraminifera in the sediments of the Andaman Sea, Contrib. Cushman Found Foraminiferal Res., 21, 123–147, 1970.
Galloway, J. J. and Wissler, S. G.: Pleistocene foraminifera from the Lomita Quarry, Palos Verdes Hills, California, J. Paleontol., 1, 35–87, 1927.
Gastaldello, M. E., Agnini, C., Westerhold, T., Drury, A. J., Sutherland, R., Drake, M. K., et al.: The Late Miocene-Early Pliocene Biogenic Bloom: An integrated study in the Tasman Sea, Paleoceanogr. Paleocl., 38, e2022PA004565, https://doi.org/10.1029/2022PA004565, 2023a.
Gastaldello, M. E., Agnini, C., and Alegret, L.: Benthic foraminifera counts and benthic foraminifera accumulation rates from IODP Hole 371-U1506A, PANGAEA [data set], https://doi.org/10.1594/PANGAEA.963742, 2023b.
Glaessner, M. F.: Studien über Foraminiferen aus der Kreide und dem Tertiär des Kaukasus; 1. Die Foraminiferen der ältesten Tertiärschichten des Nordwest – Kaukas, Problemy Paleontologii, Paleontologichesjaya Laboratoriya Moskovskogo Gosudarstvennogo Universiteta, Vol. 2–3, 349–408, 1937.
Gooday, A. J.: A response by benthic foraminifera to the deposition of phytodetritus in the deep sea, Nature, 332, 70–73, https://doi.org/10.1038/332070a0, 1988.
Gooday, A. J.: Deep-sea benthic foraminiferal species which exploit phytodetritus: characteristic features and controls on distribution, Mar. Micropaleontol., 22, 187–205, https://doi.org/10.1016/0377-8398(93)90043-W, 1993.
Gooday, A. J.: Benthic foraminifera (Protista) as tools in deep-water palaeoceanography: environmental influences on faunal characteristics, Adv. Mar. Biol., 46, 1–9, https://doi.org/10.1016/S0065-2881(03)46002-1, 2003.
Grant, K. M. and Dickens, G. R.: Coupled productivity and carbon isotope records in the southwest Pacific Ocean during the late Miocene–early Pliocene biogenic bloom, Palaeogeogr. Palaeocl., 187, 61–82, 2002.
Groeneveld, J., Steph, S., Tiedemann, R., Garbe-Schönberg, C., Nürnberg, D., and Sturm, A.: Pliocene mixed-layer oceanography for Site 1241, using combined and δ18O analyses of Globigerinoides sacculifer, in: Proceedings of the Ocean Drilling Program: Scientific Results, Vol. 202, 1–27, Texas A and M University, 2006.
Grzybowski, J.: Otwornice pokładow naftonośnych okolicy Krosna. Rozprawy Wydziału Matematyczno-Przyrodniczego, Akademia Umiejętności w Krakowie, serya 2, vol. 33, p. 293, pl. 12, fig. 12 (see also: Kaminski, M. A. 1984; shape variation in Spiroplectammina spectabilis (Grzybowski), Acta Paleontologica Polonica, 29, 29–49), 1898.
Guerin-Meneville, F. E.: Iconographie du Regne Animal de G. Cuvier, Mollusques, J. B. Bailliére, Paris, https://doi.org/10.5962/bhl.title.10331, 1843.
Gupta, A. K.: Taxonomy and bathymetric distribution of Holocene deep-sea benthic foraminifera in the Indian Ocean and the Red Sea, Micropaleontology, 40, 351–367, https://doi.org/10.2307/1485940, 1994.
Gupta, A. K. and Thomas, E.: Latest Miocene-Pleistocene Productivity and Deep-Sea Ventilation in the Northwestern Indian Ocean, Deep Sea Drilling Project Site 219, Paleoceanography, 14, 62–73, 1999.
Gupta, A. K., Das, M., and Bhaskar, K.: South Equatorial Current (SEC) driven changes at DSDP Site 237, Central Indian Ocean, during the Plio-Pleistocene: evidence from benthic foraminifera and stable isotopes, J. Asian Earth Sci., 28, 276–290, 2006.
Gupta, A. K., Raj, M. S., Mohan, K., and De, S.: A major change in monsoon-driven productivity in the tropical Indian Ocean during ca 1.2–0.9 Myr: Foraminiferal faunal and stable isotope data, Palaeogeogr. Palaeocl., 261, 234–245, 2008.
Haeckel, E.: Systematische Phylogenie, Entwurf eines Natürlichen Systems der Organismen auf Grund ihrer Stammesgeschichte, Systematische Phylogenie der Protisten und Pflanzen, 1. Georg Reimer, Berlin, https://doi.org/10.3931/e-rara-72554, 1984.
Hageman, J.: Benthic foraminiferal assemblages from Plio-Pleistocene open bay to lagoonal sediments of the western Peloponnesus (Greece), Doctoral dissertation, Utrecht University, 1979.
Haug, G. H. and Tiedemann, R.: Effect of the formation of the Isthmus of Panama on Atlantic Ocean thermohaline circulation, Nature, 393, 673–676, 1998.
Haug, G. H., Tiedemann, R., Zahn, R., and Ravelo, A. C.: Role of Panama uplift on oceanic freshwater balance, Geology, 29, 207–210, 2001.
Hayward, B. W.: Late Pliocene to middle Pleistocene extinctions of deep-sea benthic foraminifera (“Stilostomella extinction”) in the southwest Pacific, J. Foramin. Res., 32, 274–307, 2002.
Hayward, B. W.: Foraminifera-based estimates of paleobathymetry using Modern Analogue Technique, and the subsidence history of the early Miocene Waitemata Basin, New Zeal. J. Geol. Geophys., 47, 749–767, 2004.
Hayward, B. W. and Buzas, M. A.: Taxonomy and paleoecology of Early Miocene benthic foraminifera of Northern New Zealand and the North Tasman Sea, Paleobiology, 36, 154 pp., https://doi.org/10.5479/si.00810266.36.1, 1979.
Hayward, B. W., Grenfell, H. R., Reid, C. M., and Hayward, K. A.: Recent New Zealand shallow- waterbenthic foraminifera: Taxonomy, ecologic distribution, biogeography, and use in paleoenvironmental assessment, Institute of Geological and Nuclear Sciences Monograph, 21, 258 pp., 1999.
Hayward, B. W., Carter, R., Grenfell, H. R., and Hayward, J. J.: Depth distribution of Recent deep-sea benthic foraminifera east of New Zealand, and their potential for improving paleobathymetric assessments of Neogene microfaunas, New Zeal. J. Geol. Geophys., 44, 555–587, 2001.
Hayward, B. W., Neil, H., Carter, R., Grenfell, H. R., and Hayward, J. J.: Factors influencing the distribution patterns of Recent deep-sea benthic foraminifera, east of New Zealand, Southwest Pacific Ocean, Mar. Micropaleontol., 46, 139–176, 2002.
Hayward, B. W., Grenfell, H. R., Sabaa, A., and Hayward, J. J.: Recent benthic foraminifera from offshore Taranaki, New Zealand, New Zeal. J. Geol. Geophys., 46, 489–518, 2003.
Hayward, B. W., Grenfell, H. R., Carter, R., and Hayward, J. J.: Benthic foraminiferal proxy evidence for the Neogene palaeoceanographic history of the Southwest Pacific, east of New Zealand, Mar. Geol., 205, 147–184, 2004.
Hayward, B. W., Grenfell, H. R., Sabaa, A. T., and Sikes, E.: Deep-sea benthic foraminiferal record of the mid-Pleistocene transition in the SW Pacific, Geol. Soc. Lond. Spec. Publ., 247, 85–115, 2005.
Hayward, B. W., Grenfell, H. R., Sabaa, A. T., Neil, H. L., and Buzas, M.A.: Recent New Zealand deep-water benthic foraminifera: Taxonomy, ecologic distribution, biogeography and use in paleoenvironmental assessment, GNS Science Monograph, 26, 363 pp., Lower Hutt New Zealand, 2010.
Hayward, B. W., Sabaa, A. T., Grenfell, H. R., Neil, H., and Bostock, H.: Ecological distribution of recent deep-water foraminifera around New Zealand, J. Foramin. Res., 43, 415–442, https://doi.org/10.2113/gsjfr.43.4.415, 2013.
Herguera, J. C. and Berger, W.: Paleoproductivity from benthic foraminifera abundance: Glacial to postglacial change in the west-equatorial Pacific, Geology, 19, 1173–1176, 1991.
Hermelin, J. O. R.: Pliocene benthic foraminifera from the Ontong-Java plateau (western equatorial Pacific Ocean): faunal response to changing paleoenvironments, Cushman Foundation for Foraminiferal Research Special Publication, 26, 1–143, 1989.
Hofker, J.: The Foraminifera of the Siboga Expedition, Part 3, Siboga Expeditie, Monograph., 4, 1–513, 1951.
Holbourn, A., Henderson, A. S., and MacLeod, N.: Atlas of benthic foraminifera, John Wiley and Sons, https://doi.org/10.1002/9781118452493, 2013.
Hornibrook de, B. N.: Tertiary foraminifera from the Oamaru district (New Zealand) – Part 1: Systematics and distribution, New Zeal. Geol. Surv. Paleontol. Bull., 34, 1–194, 1961.
Hornibrook, N. D. B., Brazier, R. C., and Strong, C. P.: Manual of New Zealand Permian to Pleistocene foraminiferal biostratigraphy, Paleontol. Bull., 56, 1–175, 1989.
Husezima, R. and Maruhasi, M.: A new genus and thirteen new species of foraminifera from the core-sample of Kasiwazaki oil field, Niigata-ken, Journal Sigenkagaku Kenkyusyo, 1, 391–400, 1944.
Ingle Jr., J. C. and Keller, G.: Benthic foraminiferal biofacies of the eastern Pacific margin between 40∘ S and 32∘ N, in: Quaternary depositional environments of the Pacific coast: Pacific Coast Paleogeography Symposium 4, edited by: Field, M., Douglas, R. G., Bouma, A. R., et al., Pacific Section of the Society of Economic Paleontologists and Mineralogists, 341–355, 1980.
Jones, R. W. and Charnock, M. A.: “Morphogroups” of agglutinated foraminifera. Their life positions and feeding habits and potential applicability in (paleo)ecological studies, Rev. Palèobiol., 4, 311—320, 1985.
Jonkers, H. A.: Pliocene benthonic foraminifera from homogeneous and laminated marls on Crete, Doctoral dissertation, Utrecht University, 1984.
Jorissen, F. J.: Benthic foraminifera from the Adriatic Sea: principles of phenotypic variation, Doctoral dissertation, Utrecht University, 1988.
Jorissen, F. J., de Stigter, H. C., and Widmark, J. G.: A conceptual model explaining benthic foraminiferal microhabitats, Mar. Micropaleontol., 26, 3–15, https://doi.org/10.1016/0377-8398(95)00047-X, 1995.
Jorissen, F. J., Fontanier, C., and Thomas, E.: Paleoceanographical Proxies Based on Deep-Sea Benthic Foraminiferal Assemblage Characteristics, Dev. Mar. Geol., 1, 263–325, https://doi.org/10.1016/S1572-5480(07)01012-3, 2007.
Kaiho, K.: Global changes of Paleogene aerobic/anaerobic benthic foraminifera and deep-sea circulation, Palaeogeogr., Palaeocl., 83, 65–85, 1991.
Kaiho, K.: Benthic foraminiferal dissolved-oxygen index and dissolved-oxygen levels in the modern ocean, Geology, 22, 719–722, 1994.
Kaiho, K., et al.: Latest Paleocene benthic foraminiferal extinction and environmental changes at Tawanui, New Zealand, Paleoceanography, Vol. 11, 447–465, https://doi.org/10.1029/96PA01021, 1996.
Kaminski, M. A. and Gradstein, F. M.: Atlas of Paleogene Cosmopolitan Deepwater Agglutinated Foraminifera, The Grzybowski Foundation, ISBN: 83-912385-X, 2005.
Karas, C., Nürnberg, D., Tiedemann, R., and Garbe-Schönberg, D.: Pliocene climate change of the Southwest Pacific and the impact of ocean gateways, Earth Planet. Sc. Lett., 301, 117–124, 2011.
Katz, M. E. and Miller, K. G.: Neogene subsidence along the Northeast Australian margin: benthic foraminiferal evidence, in: Scientific Results of the Proceedings of the Ocean Drilling Program, edited by: Davies, P. J., McKenzie, J. A., Julson, A., Sarg, J. F., and the Shipboard Scientific Party, 133, 75–92, 1993.
Katz, M. E., Katz, D. R., Wright, J. D., Miller, K. G., Pak, D. K., Shackleton, N. J., and Thomas, E.: Early Cenozoic benthic foraminiferal isotopes: Species reliability and interspecies correction factors, Paleoceanography, 18, 1024, https://doi.org/10.1029/2002PA000798, 2003.
Kawagata, S.: Late Quaternary bathyal benthic foraminifera from three Tasman Sea cores, southwest Pacific Ocean, Science Reports, Institute of Geosciences, University of Tsukuba, Section B, 20, 1–46, 1999.
Kawagata, S.: Tasman Front shifts and associated paleoceanographic changes during the last 250,000 years: foraminiferal evidence from the Lord Howe Rise, Mar. Micropaleontol., 41, 167–191, 2001.
Kawagata, S. and Kamihashi, T.: Middle Pleistocene to Holocene Upper Bathyal Benthic Foraminifera from IODP Hole U1352B in Canterbury Basin, New Zealand, Paleontol. Res., 20, 1–85, 2016.
Kender, S., Kaminski, M. A., and Jones, R. W.: Early to middle Miocene foraminifera from the deep-sea Congo Fan, offshore Angola, Micropaleontology, 54, 477–568, 2008.
Kender, S., McClymont, E. L., Elmore, A. C., Emanuele, D., Leng, M. J., and Elderfield, H.: Mid Pleistocene foraminiferal mass extinction coupled with phytoplankton evolution, Nat. Commun., 7, 11970, https://doi.org/10.1038/ncomms11970, 2016.
Kennett, J. P. and Casey, R. E.: Foraminiferal evidence for a pre-middle eocene age of the chatham rise, New Zealand, New Zeal. J. Mar. Freshw. Res., 3, 20–28, 1969.
Kreuzberg, G.: Eine tertiare Foraminiferen fauna von Neuseeland, Neues Jahrbuch Mineralogie, Geologie und Palaeontologie, Stuttgart, Beil-Bd., 64, 271–292, 1930.
Kuhnt, W., Hess, S., and Jian, Z.: Quantitative composition of benthic foraminiferal assemblages as a proxy indicator for organic carbon flux rates in the South China Sea, in: Response of West Pacific Marginal Seas to Global Climate Change, edited by: Sarnthein, M. and Wang, P. X., Mar. Geol., 156, 123–157, 1999.
Kuhnt, W., Holbourn, A., and Zhao, Q.: The early history of the South China Sea: evolution of Oligocene-Miocene deep water environments, Rev. Micropaleontol., 45, 99–159, 2002.
Kurihara, K. and Kennett, J. P.: Neogene benthic foraminifers-distribution in depth traverse, Southwest Pacific, Initial Rep. Deep Sea, 90, 1037–1077, 1986.
Kurihara, K. and Kennett, J. P.: Bathymetric migration of deep-sea benthic foraminifera in the southwest Pacific during the Neogene, J. Foramin. Res., 18, 75–83, 1988.
Kurihara, K. and Kennett, J. P.: Paleoceanographic significance of Neogene benthic foraminiferal changes in a southwest Pacific bathyal depth transect, Mar. Micropaleontol., 19, 181–199, 1992.
Linke, P. and Lutze, G. F.: Microhabitat preferences of benthic foraminifera – a static concept or a dynamic adaptation to optimize food acquisition?, Mar. Micropaleontol., 20, 215–234, 1993.
Lobegeier, M. K. and Sen Gupta, B. K.: Foraminifera of hydrocarbon seeps, Gulf of Mexico, J. Foramin. Res., 38, 93–116, 2008.
Loeblich, A. R. and Tappan, H.: Revision of some Recent foraminiferal genera, Smithsonian Miscellaneous Collections, 128, 1–37, https://repository.si.edu/handle/10088/22914 (last access: 3 January 2024), 1955.
Loeblich, A. R. and Tappan, H.: Remarks on the systematics of the Sarcodina (Protozoa), renamed homonyms and new and validated genera, Proceedings of the Biological Society of Washington, Vol. 74, 213–234, 1961.
Loeblich, A. R. and Tappan, H.: Sarcodina, chiefl y “Thecamoebians” and Foraminiferida, in: Treatise on Invertebrate Paleontology, edited by: Moore, R. C., Part C, Protista 2 (2 vols), Lawrence, KS: Geological Society of America and University of Kansas Press, 1–900, ISBN: 978-0813730035, 1964.
Loeblich, A. R. and Tappan, H.: Suprageneric Classification of the Foraminiferida (Protozoa), Micropaleontology, 30, 1–70, https://doi.org/10.2307/1485456, 1984.
Loeblich, A. R. and Tappan, H.: Foraminiferal genera and their classification, 2 vols, Van Nostrand Reinhold, New York, 1182 pp., 1987.
Loeblich, A. R. and Tappan, H.: Foraminiferal evolution, diversification, and extinction, J. Paleontol., 62, 695–714, 1988.
Loeblich, A. R. and Tappan, H.: Foraminifera of the Sahul Shelf and Timor Sea, Cushman Foundation for Foraminiferal Research Special Publication, 31, 661 pp., 1994.
Lohmann, G. P.: Abyssal benthonic foraminifera as hydrographic indicators in the western South Atlantic Ocean, J. Foramin. Res., 8, 6–34, 1978.
Loubere, P.: Quantitative estimation of surface ocean productivity and bottom water oxygen concentration using benthic foraminifera, Paleoceanography, 9, 723–737, 1994.
Loubere, P.: The impact of seasonality on the benthos as reflected in the assemblages of deep-sea foraminifera, Deep-Sea Res. Pt. I, 45, 409–432, 1998.
Loubere, P. and Fariduddin, M.: Quantitative estimation of global patterns of surface ocean biological productivity and its seasonal variation on timescales from centuries to millennia, Global Biogeochem. Cy., 13, 115–133, 1999.
Lutze, G. F. and Thiel, H.: Epibenthic foraminifera from elevated microhabitats; Cibicidoides wuellerstorfi and Planulina ariminensis, J. Foramin. Res., 19, 153–158, 1989.
Lyle, M., Drury, A. J., Tian, J., Wilkens, R., and Westerhold, T.: Late Miocene to Holocene high-resolution eastern equatorial Pacific carbonate records: stratigraphy linked by dissolution and paleoproductivity, Clim. Past, 15, 1715–1739, https://doi.org/10.5194/cp-15-1715-2019, 2019.
Mackensen, A.: Neogene benthic foraminifers from the southern Indian Ocean (Kerguelen Plateau) biostratigraphy and paleoecology, Science Results, Proc. ODP, 120, 649–673, 1992.
Mackensen, A., Schmiedl, G., Harloff, J., and Giese, M.: Deep-sea foraminifera in the South Atlantic Ocean: ecology and assemblage generation, Micropaleontology, 41, 342–358, https://doi.org/10.2307/1485808, 1995.
Mancin, N., Hayward, B. W., Trattenero, I., Cobianchi, M., and Lupi, C.: Can the morphology of deep-sea benthic foraminifera reveal what caused their extinction during the mid-Pleistocene Climate Transition?, Mar. Micropaleontol., 104, 53–70, 2013.
Martin, R. A., Nesbitt, E. A., and Campbell, K. A.: The effects of anaerobic methane oxidation on benthic foraminiferal assemblages and stable isotopes on the Hikurangi Margin of eastern New Zealand, Mar. Geol., 272, 270–284, 2010.
Mathews, R. D.: Rectuvigerina, a new genus of foraminifera from a restudy of Siphogenerina, J. Paleontol., 19, 588–606, p. 590, 1945.
Mead, G. A.: Recent benthic foraminifera in the Polar Front region of the southwest Atlantic, Micropaleontology, 31, 221–248, 1985.
Miller, K. G. and Lohmann, G. P.: Environmental distribution of Recent benthic foraminifera on the northeast United States continental slope, Geol. Soc. Am. Bull., 93, 200–206, 1982.
Milker, Y. and Schmiedl, G.: A taxonomic guide to modern benthic shelf foraminifera of the western Mediterranean Sea, Palaeontol. Electron., 15, 1–134, 2012.
Montfort P.: Conchyliologie systématique et classification méthodique des coquilles, Paris: Schoell, Vol. 1, pp. lxxxvii + 409, p. 66, 1808.
Murray, J. W.: Ecology and palaeoecology of benthic foraminifera: Longman Scientific and Technical, Harlow, Essex, UK, 1991.
Murray, J. W.: Ecology and applications of benthic foraminifera, Cambridge University Press, 2006.
Nees, S.: The Benthic Foraminiferal Record Of Late Quaternary Glacial/Interglacial Cycles In Core E36-23, Se Tasman Plateau, in: The evolution of the Tasman Sea Basin, edited by: Van der Lingen, G. J., Swanson, K. M. and Muir, R. J., Proceedings of the Tasman Sea Conference, November 1992, Christchurch, New Zealand, Rotterdam, Balkema Publishers, ISBN 9789054103288, 1994.
Nees, S.: Late Quaternary palaeoceanography of the Tasman Sea: the benthic foraminiferal view, Palaeogeogr. Palaeocl., 131, 365–389, 1997.
Nees, S., Armand, L., De Deckker, P., Labracherie, M., and Passlow, V.: A diatom and benthic foraminiferal record from the South Tasman Rise (southeastern Indian Ocean): implications for palaeoceanographic changes for the last 200,000 years, Mar. Micropaleontol., 38, 69–89, 1999.
Nomura, R.: Cassidulinidae (Foraminiferida) from the Uppermost Cenozoic of Japan – Part 2, Tohoku University Science Reports, Series 2, Geology, 54, 1–93, 1983.
Nomura, R.: Paleogene to Neogene deep-sea paleoceanography in the eastern Indian Ocean: benthic foraminifera from ODP Sites 747, 757 and 758, Micropaleontology, 41, 251–290, https://doi.org/10.2307/1485862, 1995.
Nomura, R., Peirce, J. W., Weissel, J. K., Taylor, E., Dehn, J., Driscoll, N. W., et al.: Oligocene to Pleistocene benthic foraminifer assemblages at sites 754 and 756, eastern Indian Ocean, in: Proceedings of the Ocean Drilling Program, Scientific results, Vol. 121, 31–75, Texas A and M University, Ocean Drilling Program, 1991.
Nuttall, W. L. F.: Lower Oligocene foraminifera from Mexico, J. Paleontol., 6, 3–35, 1932.
Parker, W. K. and Jones, T. R.: VI. On some foraminifera from the North Atlantic and Arctic Oceans, including Davis Straits and Baffin's Bay, Philos. T. R. Soc. Lond., 155, 325–441, 1865.
Patterson, R. T.: Abditodendrix, a new foraminiferal genus in the family Bolivinitidae, J. Foramin. Res., 15, 138–140, 1985.
Peterson, L. C., Murray, D. W., Ehrmann, W. U., and Hempel, P.: Cenozoic carbonate accumulation and compensation depth changes in the Indian Ocean, Washington, D.C., American Geophysical Union Geophysical Monograph Series, 70, 311–333, 1992.
Pflum, C. E. and Frerichs, W. E.: Gulf of Mexico deep-water foraminifers, Cushman Foundation for Foraminiferal Research Special Publication, 14, 125 pp., 1976.
Phleger, F. B. and Parker, F. L.: Ecology of foraminifera, northwest Gulf of Mexico, Part II. Foraminifera species, Mem. Geol. Soc. Am., 46, 1–64, 1951.
Phleger, F. B., Parker, F. L., and Peirson, J. F.: Sediment cores from the North Atlantic Ocean, Swedish Deep-Sea Exped., 7, 3–122, 1953.
Pillot, Q., Suchéras-Marx, B., Sarr, A. C., Bolton, C. T., and Donnadieu, Y.: A global reassessment of the spatial and temporal expression of the Late Miocene Biogenic Bloom, Paleoceanogr. Paleocl., 38, e2022PA004564, https://doi.org/10.1029/2022PA004564, 2023.
Reuss, A. E.: Neue Foraminiferen aus den Schichten des österreichischen Tertiärbeckens, Denkschriften der Kaiserlichen Akademie der Wissenschaften, Mathematisch – Naturwissenschaftliche Classe, Vol. 1, 365–390, 1850.
Reuss, A. E.: Die Foraminiferen und Entomostraceen des Kreidemergels von Lemberg, Naturwissenschaftliche Abhandlungen, Wien, Österreich, Vol. 4, 17–52, 1851.
Revets, S. A.: The Generic Revision of Five Families of Rotaliine Foraminifera, Part 1 – The Bolivinitidae, Part 2 – The Anomalinidae, Alabaminidae, Cancrisidae and Gavelinellidae, Cushman Found. Foramin. Res., 34, 1–55 ISBN: 9781970168228, 1996.
Ridha, D., Boomer, I., and Edgar, K. M.: Latest Oligocene to earliest Pliocene deep-sea benthic foraminifera from Ocean Drilling Program (ODP) Sites 752, 1168 and 1139, southern Indian Ocean, J. Micropalaeontol., 38, 189–229, 2019.
Robertson, B. E.: Systematics and paleoecology of the benthic foraminiferida from the Buff Bay section, Miocene of Jamaica, Micropaleontology, 44, 1–266, 1998.
Russo, B., Curcio, E., and Iaccarino, S.: Paleoecology and paleoceanography of a Langhian succession (Tremiti Islands, southern Adriatic Sea, Italy) based on benthic foraminifera, Boll. Leg. It., 46, 107–124, 2007.
Saidova, K. M.: Bentosniye foraminifery Tikhogo Okeana, P.P. Shirshov Institute of Oceanology, Academy of Sciences of the USSR, Moscow, 3 parts, 1975.
Schlumberger, C.: Note sur le genre Planispirina, B. Soc. Zool. Fr., 11, 105–118, 1887.
Schmiedl, G., Mackensen, A., and Müller, P. J.: Recent benthic foraminifera from the eastern South Atlantic Ocean: dependence on food supply and water masses, Mar. Micropaleontol., 32, 249–287, 1997.
Schnitker, D.: The deep waters of the western North Atlantic during the past 24,000 years, and the re-initiation of the Western Boundary Undercurrent, Mar. Micropaleontol., 4, 265–280, 1979.
Schönfeld, J. and Altenbach, A. V.: Late Glacial to Recent distribution pattern of deep-water Uvigerina species in the north-eastern Atlantic, Mar. Micropaleontol., 57, 1–24, 2005.
Schwager, C.: Fossile Foraminiferen von Kar Nikobar, Reise der Österreichischen Fregatte Novara um die Erde in den Jahren 1857, 1858, 1859, Palaontol. Mitteil., 2, 187–268, 1866.
Schweizer, M.: Evolution and molecular phylogeny of Cibicides and Uvigerina (Rotaliida, Foraminifera), Doctoral dissertation, University of Utrecht, 2006.
Schroder-Adams, C. J.: Middle Eocene to Holocene benthic foraminifer assemblages from the Kerguelen Plateau (southern Indian Ocean), in: Proceedings of the Ocean Drilling Program, Scientific Results, College Station, Vol. 119, 611–630, 1991.
Schultze, M. J. S.: Über den Organismus der Polythalamien (Foraminiferen), nebst Bemerkungen über die Rhizopoden im allgemeinen, Ingelmann, Leipzig, 1–68, https://books.google.pt/books?id=o7rk00_xueQC (last access: 3 January 2024), 1854.
Schwager, C.: Saggio di una classificazione dei foraminiferi avuto riguardo alle loro famiglie naturali, Bolletino R, Comitato Geologico d'Italia, 7, 475–485, 1876.
Seiglie, G. A. and Baker, M. B.: Duquepsammiidae, a new family, and Duquepsammia, a new genus of agglutinated foraminifers, Micropaleontology, 33, 263–266, https://doi.org/10.2307/1485642, 1987.
Sejrup, H. P. and Guilbault, J. P.: Cassidulina reniforme and C. obtusa (Foraminifera), taxonomy, distribution, and ecology, Sarsia, 65, 79–85, 1980.
Silvestri, A.: Ricerche strutturali su alcune forme dei Trubi dei Bonfornello (Palermo), Memorie dell'Accademia Pontificia dei Nuovi Lincei, 22, 235–276, 1904.
Shannon, C. E.: A mathematical theory of communication, Bell Syst. Tech. J., 27, 379–423, 1948.
Smart, C. W., King, S. C., Gooday, A. J., Murray, J. W., and Thomas, E.: A benthic foraminiferal proxy of pulsed organic matter paleofluxes, Mar. Micropaleontol., 23, 89–99, 1994.
Smart, C. W., Thomas, E., and Ramsay, A. T.: Middle–late Miocene benthic foraminifera in a western equatorial Indian Ocean depth transect: Paleoceanographic implications, Palaeogeogr. Palaeocl., 247, 402–420, 2007.
Smith, P. B.: Ecology of benthonic species, US Government Printing Office, p. 55, 1964.
Steph, S., Tiedemann, R., Groeneveld, J., Sturm, A., and Nürnberg, D.: Pliocene changes in tropical east Pacific upper ocean stratification: Response to tropical gateways?, Proceedings of the Ocean Drilling Program: Scientific Results, Vol. 202, 1–51, 2006.
Streeter, S. S. and Shackleton, N. J.: Paleocirculation of the deep North Atlantic: 150,000-year record of benthic foraminifera and oxygen-18, Science, 203, 168–171, 1979.
Suhr, S. B. and Pond, D. W.: Antarctic benthic foraminifera facilitate rapid cycling of phytoplankton-derived organic carbon, Deep-Sea Res. Pt. II, 53, 895–902, 2006.
Suhr, S. B., Pond, D. W., Gooday, A. J., and Smith, C. R.: Selective feeding by benthic foraminifera on phytodetritus on the western Antarctic Peninsula shelf: evidence from fatty acid biomarker analysis, Mar. Ecol. Prog. Ser., 262, 153–162, 2003.
Sutherland, R., Dickens, G. R., Blum, P., Agnini, C., Alegret, L., Asatryan, G., Bhattacharya, J., Bordenave, A., Chang, L., Collot, J., Cramwinckel, M. J., Dallanave, E., Drake, M. K., Etienne, S. J. G., Giorgioni, M., Gurnis, M., Harper, D. T., Huang, H.-H. M., Keller, A. L., Lam, A.R., Li, H., Matsui, H., Morgans, H. E. G., Newsam, C., Park, Y.-H., Pascher, K. M., Pekar, S. F., Penman, D. E., Saito, S., Stratford, W. R., Westerhold, T., and Zhou, X.: Site U1506, in: Tasman Frontier Subduction Initiation and Paleogene Climate., edited by: Sutherland, R., Dickens, G. R., Blum, P., and the Expedition 371 Scientists, Proceedings of the International Ocean Discovery Program, 371, College Station, TX, International Ocean Discovery Program, https://doi.org/10.14379/iodp.proc.371.103.2019, 2019a.
Sutherland, R., Dickens, G. R., Blum, P., Agnini, C., Alegret, L., Asatryan, G., Bhattacharya, J., Bordenave, A., Chang, L., Collot, J., Cramwinckel, M. J., Dallanave, E., Drake, M. K., Etienne, S. J. G., Giorgioni, M., Gurnis, M., Harper, D. T., Huang, H.-H. M., Keller, A. L., Lam, A. R., Li, H., Matsui, H., Morgans, H. E. G., Newsam, C., Park, Y.-H., Pascher, K. M., Pekar, S. F., Penman, D. E., Saito, S., Stratford, W. R., Westerhold, T., and Zhou, X.: Expedition 371 methods, in: Tasman Frontier Subduction Initiation and Paleogene Climate, edited by: Sutherland, R., Dickens, G. R., Blum, P., and the Expedition 371 Scientists, Proceedings of the International Ocean Discovery Program, 371: College Station, TX, International Ocean Discovery Program, https://doi.org/10.14379/iodp.proc.371.102.2019, 2019b.
Sutherland, R., Dickens, G. R., Blum, P., Agnini, C., Alegret, L., Asatryan, G., et al.: Continental-scale geographic change across Zealandia during Paleogene subduction initiation. Geology, 48, 419–424, 2020.
Sutherland, R., Dos Santos, Z., Agnini, C., Alegret, L., Lam, A. R., Westerhold, T., et al.: Neogene mass accumulation rate of carbonate sediment across northern Zealandia, Tasman Sea, southwest Pacific, Paleoceanogr. Paleocl., 37, e2021PA004294, https://doi.org/10.1029/2021PA004294, 2022.
Thalmann, H. E.: Bibliography and index to new genera, species and varieties of foraminifera for the year 1936, J. Paleontol., 13, 425–465, 1939.
Thomas, E.: Late Eocene to Recent deep-sea benthic foraminifers from the central equatorial Pacific Ocean, Initial Rep. Deep Sea, 85, 655–694, 1985.
Thomas, E.: Late Cretaceous through Neogene deep-sea benthic foraminifera (Maud Rise, Weddell Sea, Antarctica), in: Proc. ODP, Science Results, College Station, Vol. 113, 571–594, 1990.
Thomas, E. and Gooday, A. J.: Cenozoic deep-sea benthic foraminifers: tracers for changes in oceanic productivity?, Geology, 24, 355–358, 1996.
Thomas, E. and Shackleton, N. J.: The Paleocene-Eocene benthic foraminiferal extinction and stable isotope anomalies, Geol. Soc., Lond. Spec. Publ., 101, 401–441, 1996.
Tjalsma, R. C. and Lohmann, G. P.: Paleocene–Eocene bathyal and abyssal benthic foraminifera from the Atlantic Ocean, Special Publication, Micropaleontology, 4, 1–90, 1983.
Trauth, F.: Das Eozänvorkommen bei Radstadt im Pongau und seine Beziehungen zu den gleichalterigen Ablagerungen bei Kirchberg am Wechsel und Wimpassing am Leithagebirge,Denkschriften der Kaiserlichen Akademie der Wissenschaften Wien, Mathematisch-Naturwissenschaftliche Classe, 95, 171–278, 1918.
Van der Zwaan, G. J.: Paleoecology of late Miocene Mediterranean foraminifera, Doctoral dissertation, Utrecht University, 1982.
Van Morkhoven, F. P. C. M., Berggren, W. A., Edwards, A. S., and Oertli, H. J.: Cenozoic cosmopolitan deep-water benthic Foraminifera, Pau, Elf Aquitaine, 1986.
Vella, P.: Studies in New Zealand foraminifera, Part 1 – Foraminifera from Cook Strait, Part 11 – Upper Miocene to Recent species of the genus Notorotalia, New Zeal. Geol. Surv. Paleontol. Bull., 28, 1–64, 1957.
Vénec-Peyré, M. T.: “Les Planches inédites de Foraminiféres d'Alcide d'Orbigny: á l'aube de la micropaleontology”, Muséum national d'Histoire naturelle, Paris, 302 pp., 2005.
Voloshinova, N. A.: Iskopaemye Foraminifery SSRS, Nonionidy, Kassidulinidy i Khilostomellidy, Fossil foraminifera of the USSR, Nonionidae, Cassidulinidae and Chilostomellidae, edited by: Voloshinova, N. A. and Dain, L. G., Vsesoyuznogo Neftyanogo Nauchnoissledovatel'skogo Geologo-razvedochnogo Instituta (VNIGRI), 63, 1–114, 1952.
von Gümbel, C. W.: Beiträge zur Foraminiferenfauna der nordalpinen Eocängebilde, Abhandlungen der K. Bayerischen Akademie der Wissenschaften (1870), 10, 581–730, 1868.
Williamson, W. C.: On the Recent Foraminifera of Great Britain, London, Ray Society, 107 pp., 1858.
Wright, R.: Neogene benthic foraminifera from DSDP leg 42A, Mediterranean Sea, DSDP Initial Reports, 42, 709–726, 1978.
Yassini, I. and Jones, B. G.: Foraminiferida and ostracoda from estuarine and shelf environments on the southeastern coast of Australia, University of Wollongong Press, Australia, 484 pp., 1995.